Quantum biology: Your nose and house plant are experts at particle physics
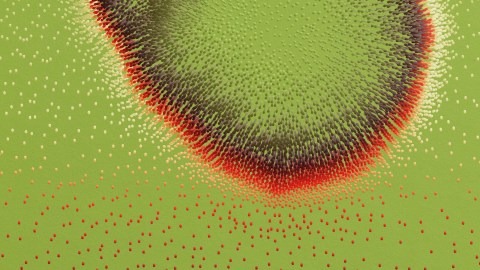
- Quantum processes, normally associated with the very small or very cold, have been found to occur in biological systems. This was unexpected.
- It appears that biology utilizes quantum tunneling, entanglement, and coherence quite effectively.
- Understanding how biological systems use these processes can give us new insights into how they work.
Quantum physics governs the world of the very small and that of the very cold. Your dog cannot quantum-tunnel her way through the fence, nor will you see your cat exhibit wave-like properties. But physics is funny, and it is continually surprising us. Quantum physics is starting to show up in unexpected places. Indeed, it is at work in animals, plants, and our own bodies.
We once thought that biological systems are too warm, too wet, and too chaotic for quantum physics to play any part in how they work. But it now seems that life is employing feats of quantum physics every day in messy, real-world systems, including quantum tunneling, wave-particle duality, and even entanglement. To see how it all works, we can start by looking right inside our own noses.
The quantum nose
The human nose can distinguish over one trillion smells. But how exactly the sense of smell works is still a mystery. When a molecule referred to as an odorant enters our nose, it binds to receptors. Initially, the prevailing theory held that these receptors used the shape of the odorants to differentiate smells. The so-called lock and key model suggests that when an odorant finds the right receptor, it fits into it and triggers a specific smell. But the lock and key model ran into trouble when tested. Subjects were able to tell two scents apart, even when the odorant molecules were identical in shape. Some other process must be at work.
Another, still controversial, model suggests that our noses are sensitive to the vibrations within odorant molecules. These vibrations occur when individual atoms within the molecule oscillate back and forth with respect to one another, as if they were loaded onto tiny springs. When an odorant settles into a receptor, the energy of its vibrations causes an electron to quantum tunnel to another location in the receptor.
The shape and vibrational models can be combined. In the so-called swipe card model, our noses are sensitive to both the shape of and vibrations within an odorant.
A new model, labeled the luminescence hypothesis, proposes still another step. Once the electron tunnels to a new position within the receptor, it loses its energy. As it does, the hypothesis proposes, the electron emits photons. Our noses detect these photons, and this helps us distinguish smells. Interestingly, the authors of this hypothesis suggest that it might help explain why some people with COVID lose their sense of smell.
Entangled navigation
Every year, Arctic terns set off on a migration that takes them around the world. They travel from Greenland to the Weddell Sea off Antarctica — a journey of 90,000 kilometers, all without the help of Google Maps. Birds have an uncanny ability to navigate. They can find the right direction of travel despite changes in weather, and at all hours of the day or night. Scientists believe this is possible because birds can sense the Earth’s magnetic field, allowing them to precisely align their position on the globe.
It appears to work something like this: Normally, two electrons can occupy an orbital, one with spin up, and one with spin down. But occasionally, something, such as a high-energy photon, can knock a single electron off a molecule, transferring it to another molecule. Both the giving and receiving molecules are now radicals — that is, molecules with an unpaired electron within one of their orbitals.
Radicals are very reactive, but what’s more, when they are created in this way, they become entangled with one another, forming what’s known as a radical pair. These pairs are created in proteins called cryptochromes within birds’ eyes. This radical pair will oscillate back and forth remarkably quickly between having spins aligned or anti-aligned with one another. Spin is spin angular momentum, which gives the molecule a magnetic moment. Because of this, the amount of time these molecules spend in each aligned or anti-aligned state is very sensitive to external magnetic fields, so sensitive, in fact, that birds can detect changes thousands of times less than the strength of Earth’s magnetic field.
Quantum evolution
Quantum mechanics might also play a part in evolution. DNA has four bases, called A (adenine), C (cytosine), G (guanine), and T (thymine). The “shapes” of these bases make it so that A always bonds to T, and G to C. During replication, an enzyme “unzips” the DNA strand from top to bottom. Both sides of this unzipped DNA can now be built into identical DNA strands, matching A to C and G to T.
Bases are held via hydrogen bonds, where a hydrogen atom attracts a negatively charged molecule, sort of like a magnet. Rather than sharing electrons, these bonds stick electrostatically together. As the DNA is unzipped, occasionally the hydrogen nucleus (a proton) can quantum tunnel to the opposite side of the unzipped DNA. When this happens, it creates a tautomer. A tautomer is another version of a molecule with the same chemical formula but a different shape, and hence, different connectivity.
Tautomers that survive long enough in the duplication process can cause the wrong bases to pair with one another. At first, it was believed that tautomers didn’t survive long enough to last through the entire replication process. But recently, scientists found that these tautomeres can survive through DNA replication and cause a mutation — and over time, may influence the course of evolution.
Plants with a PhD
Plants and bacteria that utilize photosynthesis use the quantum nature of light to convert sunlight into energy using quantum coherence. When sunlight hits a plant, chlorophyll molecules within the leaf absorb photons of specific colors, and these photons excite an electron within the chlorophyll. That energy then moves from the chlorophyll molecules to a structure called the reaction center, where it is converted into chemical energy stored for the plant’s use.
But the path from chlorophyll to the reaction center is not direct, nor is it easy to find. The energy has to reach its destination fast, otherwise it will be lost as heat. To ease this challenge, the plant uses a clever trick called quantum coherence. Instead of taking one path and hoping this is the way to reach the reaction center, the electron uses its wave-like nature and takes all available paths at once, finding the reaction center every time. Quantum coherence is closely related to quantum entanglement, which is also shown to give plants a boost in efficiency.