Ask Ethan: What New Evidence Could Revolutionize All Of Known Physics?
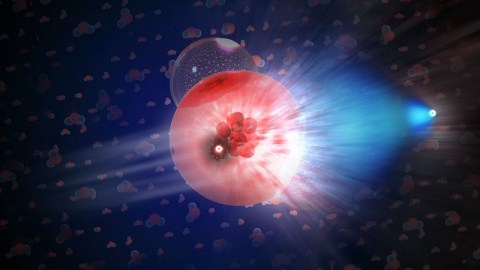
The Standard Model and General Relativity can’t be all there is. But how will we discover what lies beyond them?
One of the biggest problems with physics is that, apart from a few mysteries we cannot adequately explain, the things we do understand work extremely well. Too well, in fact, for our comfort. Almost any changes we attempt to make to the Standard Model or General Relativity, our two best (yet fundamentally incompatible) theories describing the Universe, come highly constrained by the full suite of data we already possess. And yet, there must be more to the Universe, as mysteries like dark matter, dark energy, and the matter-antimatter asymmetry are all hitherto unexplained. So where should we look for the next great revolution in fundamental physics? That’s what John Jordano wants to know, asking:
You’ve been a vocal proponent of the consensus view in physics. Other physicists sometimes spout wild theories, while you’ve clearly explained the current consensus views using succinct arguments, clear data, and in ways that lay people can understand. My question is: what are the areas of the current scientific consensus in physics that you think will possibly be shaken by experiments that we can actually carry out in the next 20 to 30 years?
It’s a fantastic question. Let’s look beyond the current frontiers to see where we’re headed.
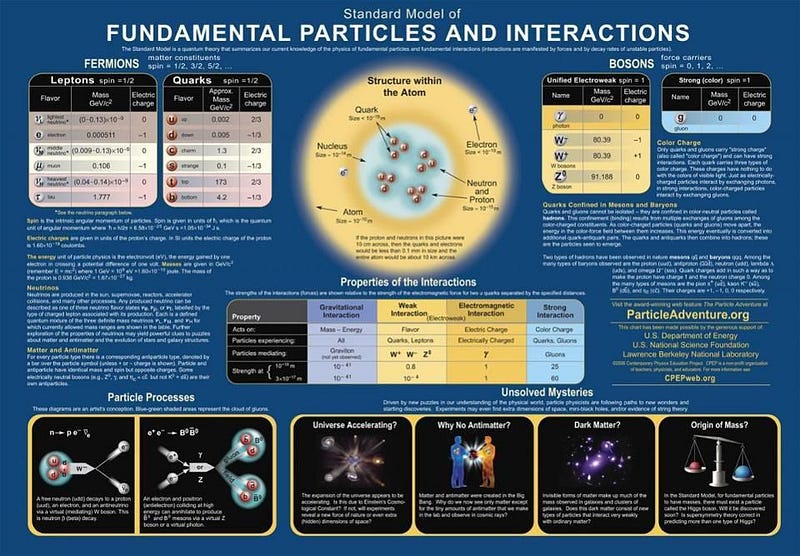
To know where we’re headed, we first need to know where we are. We know that we live in a Universe where the Standard Model of elementary particle physics has successfully explained every known, detected interaction between particles ever observed so far. The Universe is made up of quarks, leptons, and the gauge bosons that mediate three of the four fundamental forces, as well as the Higgs, which gives rest mass to all the massive particles of the Standard Model.
There’s also General Relativity: our (non-quantum) theory of gravity, which puts forth the relationship between spacetime and the matter and energy in the Universe. Put simply, matter and energy tells spacetime how to curve, while that same curved spacetime tells matter and energy how to move.
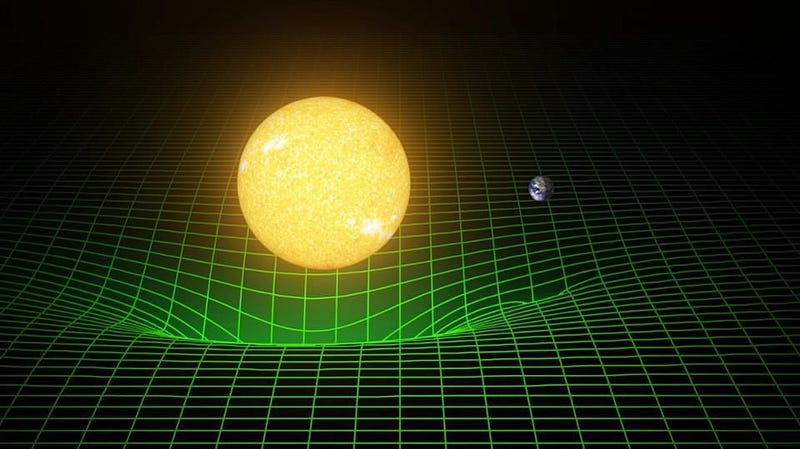
The difficulty with going beyond General Relativity (which accounts for gravitation, black holes, the expanding Universe, and the hot Big Bang) and the Standard Model (which accounts for the other three forces, the known particles and antiparticles, and the results of every particle physics experiment ever) is that if you try to modify them in almost any simple, straightforward fashion, you wind up getting results that conflict with the measurements and observations that we already possess.
It’s easy to play the “both sides” game with our current consensus theories in physics. “Well, Ethan may be a PhD astrophysicist and he says that the Standard Model and General Relativity are correct, but [insert small group of scientists] says [alternative theory] is correct, and I find that scenario more compelling.” Unfortunately, that’s not how science actually works.
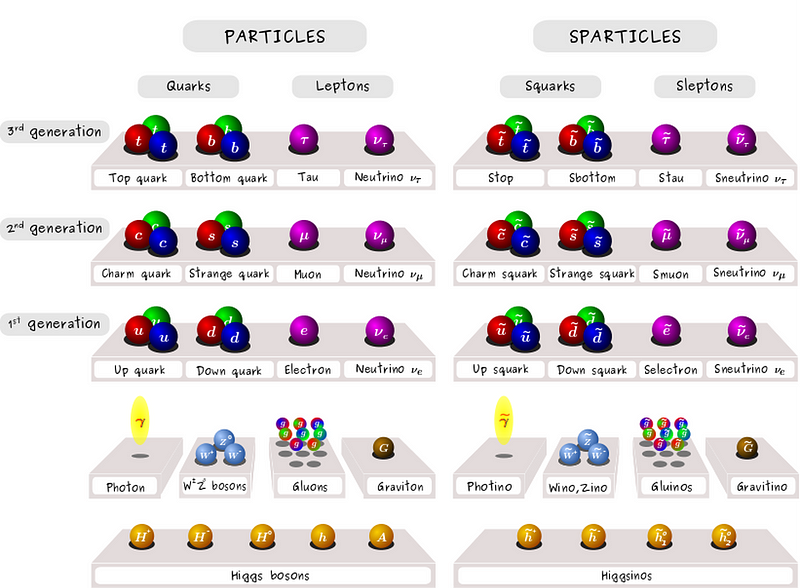
If you want to go beyond our current scientific understanding, you have quite a high burden of proof. In particular, you must overcome the following three hurdles:
- you must successfully reproduce all the successes of the prevailing theory where it’s relevant and valid,
- you must explain already-observed or measured phenomena that the prevailing theory cannot or does not explain,
- and you must make a novel, testable prediction that differs from the prevailing theory, and then go out and perform the critical test.
Yet most attempted extensions fail at even the first step. We have so many precision tests of both gravity and elementary particles that any alternatives you can cook up — from modified gravity theories to extra dimensions to additional fundamental symmetries or unifications — already have very stringent limits on their existence.
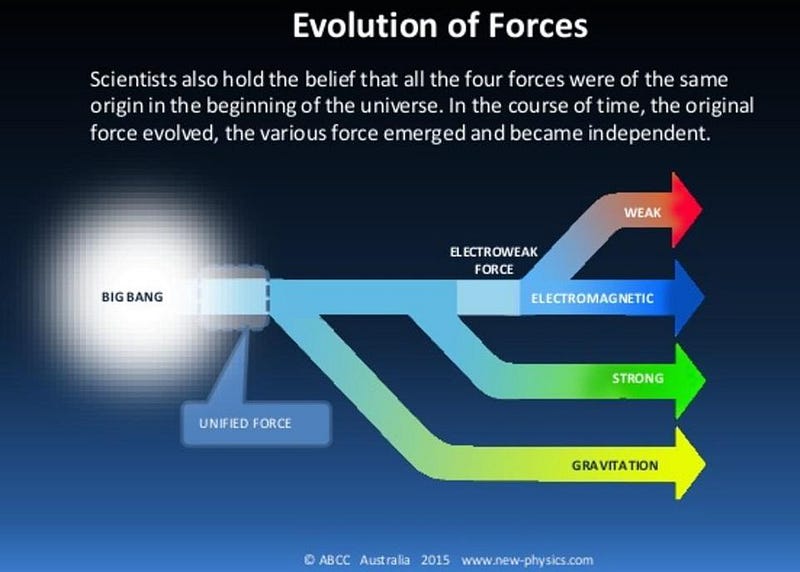
And yet, we already have some very strong pieces of evidence that what we know is true, today, cannot be the full story.
We know that distant galaxies appear to speed away from us at a rate that’s inconsistent with a Universe being filled only with Standard Model particles and governed by General Relativity.
We know that individual gravitational sources — galaxies, clusters of galaxies, and even the great cosmic web — do not agree with predictions unless a new ingredient, such as dark matter, is added.
We know that even though the laws of physics under the Standard Model produce or destroy matter and antimatter in equal amounts, we inhabit a Universe made overwhelmingly of matter, with only a trace amount of antimatter.
In other words, we know that “known physics” doesn’t account for everything that we observe in the Universe.

We’ve seen hints of what might reside beyond the currently known limits of science. On the particle physics front, a number of experiments have yielded unexpected results that, if they hold up at higher significances, could be revolutionary. The Atomki anomaly sees a set of decaying particles displaying bizarre, unexpected behavior that could be an experimental error, or signs of a novel particle that’s not part of the Standard Model. The controversial DAMA experiment, as well as recent XENON results, could represent new physics or, in a more mundane scenario, a novel source of noise.
Meanwhile, in space, the Alpha Magnetic Spectrometer sees an unexplained excess of antimatter, NASA’s Fermi satellite sees an excess of gamma rays from the galactic center, different techniques to measure the Universe yield different values of its expansion rate, and so on.
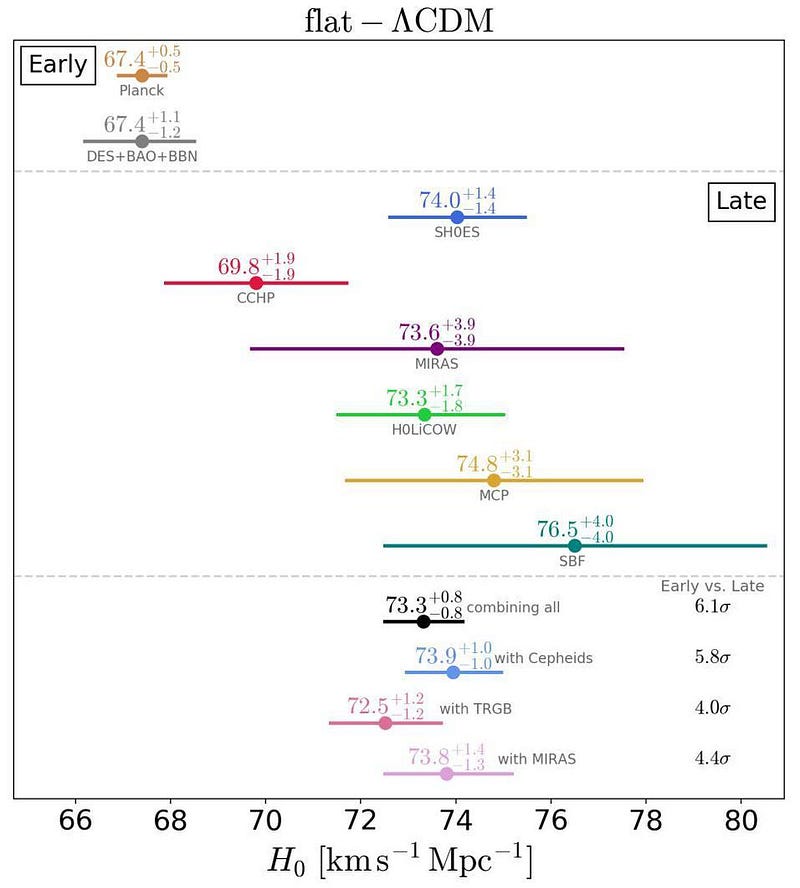
None of these results, however, are so overwhelmingly robust that they must be a sign of new physics; any or all of them could simply be statistical fluctuations or an improperly calibrated apparatus. Many of them could point to new physics, but they could just as easily be explained by known particles and phenomena within the context of General Relativity and the Standard Model.
These and other experiments will continue to go on, probing these anomalies and looking for others while we continue to refine our picture of the Universe. But over the coming decades, new experiments and observatories will come online, pushing our frontiers farther than ever before and opening up what we call “new discovery potential” by exploring the Universe in novel ways. Here are the ones I’m most excited about.
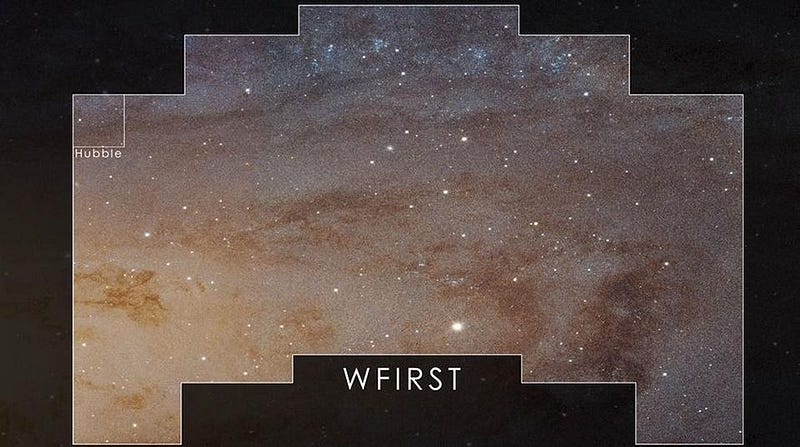
Is dark energy truly a constant? Right now, it seems constant, but there’s quite a bit of wiggle-room. Based on upcoming large-scale galaxy surveys (led by the Vera Rubin Observatory) and distant supernova data (provided by the upcoming Nancy Grace Roman telescope, formerly WFIRST), we should know to within 1% whether dark energy evolves over time. If it does, our “standard” cosmological model will have to be revised.
Can dark matter be directly detected? The newest results from the XENON experiment provide the most exciting candidate evidence for particle dark matter we’ve ever seen, but the next generation of experiments will put that to the test. The upgraded XENONnT experiment, as well as the LUX-ZEPLIN experiment, will either turn up particle dark matter or eliminate the best (and arguably, only) current candidate we have.
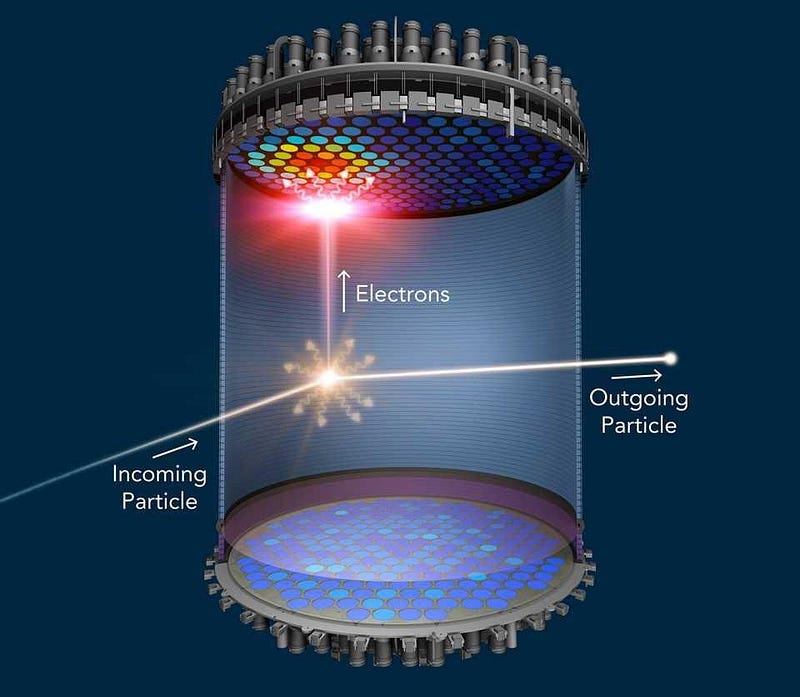
What happens at the highest energies of all? Cosmic ray experiments that look for neutrinos, Cherenkov radiation, or other high-energy signals have found particles with millions of times the energy that the Large Hadron Collider (LHC) can achieve. If there’s new physics at high energies, this is our best probe.
When did the first stars truly form? Hubble is fundamentally limited by its light-gathering power (i.e., its size), its field-of-view, and its wavelength range. NASA’s upcoming James Webb Space Telescope, as well as the upcoming generation of ground-based 30 meter class telescopes, can probe the earliest, most distant stars and galaxies as never before, seeking a greater understanding of structure formation at the earliest times.
Are there particle physics hints that defy the Standard Model? Maybe. We’re working to better-measure the magnetic moments of the electron and muon; if they disagree, there’s new physics. We’re working to uncover how neutrinos oscillate; there may be new physics there. And if we build a precision electron-positron collider, either circularly or linearly, we might find hints beyond the Standard Model that the LHC cannot find.
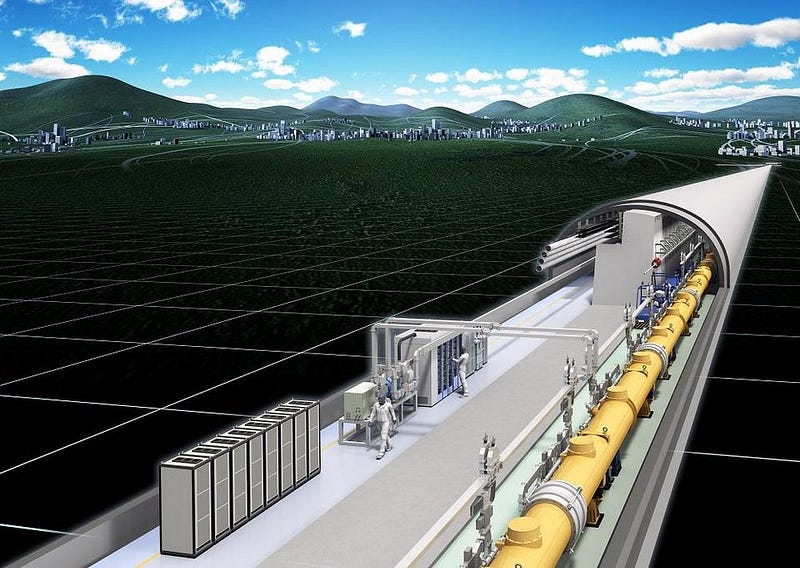
There are plenty of other options as to where new physics might be hiding, and plenty of other options as to what experiments or observations might reveal it. It’s possible that the Laser Interferometer Space Antenna (LISA) will reveal surprises; it’s possible that annihilating dark matter or sterile neutrinos will reveal themselves; it’s possible that clever tabletop experiments will provide us with our first hints of quantum gravity. Until we look, we cannot know.
But what’s most exciting to me is the “none of the above” option. Sure, it’s possible that nothing fundamentally new will be discovered when we look, but it’s also possible that we’ll find something we haven’t even stopped to consider. The beauty of scientific investigation lies in the journey of finding things out. It will take a herculean effort to discover what secrets lie beyond the current frontier. But with thousands of scientists on the case, devoting their lives to the effort, unprecedented knowledge is sure to be a reward we can all appreciate and enjoy.
Send in your Ask Ethan questions to startswithabang at gmail dot com!
Ethan Siegel is the author of Beyond the Galaxy and Treknology. You can pre-order his third book, currently in development: the Encyclopaedia Cosmologica.