CONFIRMED: The Last Great Prediction Of The Big Bang!
A leftover glow unlike any other — of neutrinos — has finally been seen.
“When you see how fragile and delicate life can be, all else fades into the background.” –Jenna Morasca
Seventy years ago, we had taken fascinating steps forward in our conception of the Universe. Rather than living in a Universe governed by absolute space and absolute time, we lived in one where space and time were relative, depending on the observer. We no longer lived in a Newtonian Universe, but rather one governed by general relativity, where matter and energy cause the fabric of spacetime itself to curve.
And thanks to the observations of Hubble and others, we learned that our Universe was not static, but rather was expanding over time, with galaxies getting farther and farther apart as time went on.
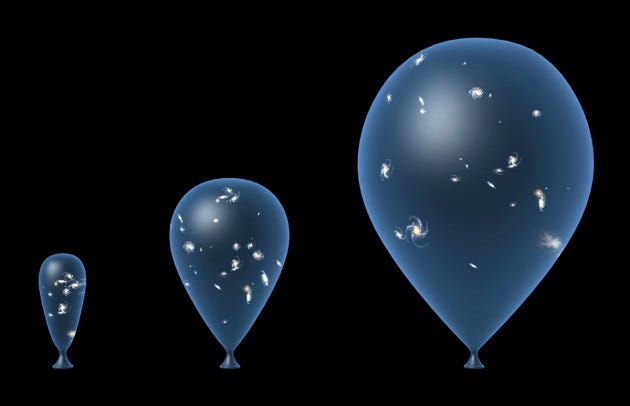
But in 1945, George Gamow made perhaps the greatest leap of all: the great leap backwards. If the Universe were expanding today, with all the unbound objects receding from one another, then perhaps that meant that all those objects were closer together in the past. Perhaps the Universe we live in today evolved from a denser state long ago. Perhaps gravitation has clumped and clustered the Universe together over time, while it was more even and uniform in the distant past. And perhaps — since the energy of radiation is tied to its wavelength — that radiation was more energetic in the past, and hence the Universe was hotter long ago.
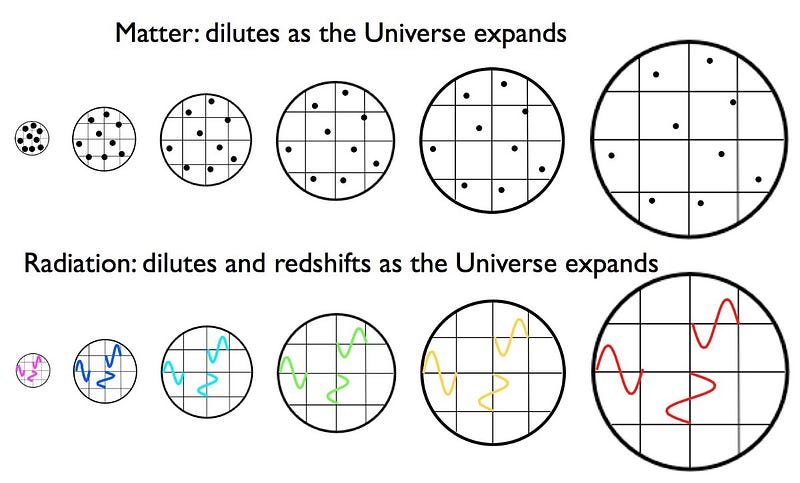
And if this were the case, it brought up an incredibly interesting set of events as we looked farther and farther back into the past:
- There was a time before large galaxies formed, where only small proto-galaxies and star clusters had come to be.
- Before that, there was a time before gravitational collapse had formed any stars, and all was dark: just primeval atoms and low-energy radiation.
- Prior to that, the radiation was so energetic that it could knock electrons off of the atoms themselves, creating a high-energy, ionized plasma.
- Even earlier than that, the radiation reached such levels that even atomic nuclei would be blasted apart, creating free protons and neutrons, and forbidding the existence of heavy elements.
- And finally, at even earlier times, the radiation would have so much energy that — through Einstein’s E = mc^2 — matter-and-antimatter pairs would spontaneously be created.
This picture is part of what’s known as the hot Big Bang, and it makes a whole slew of predictions.
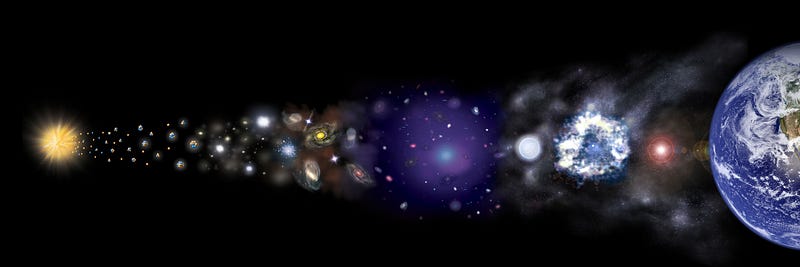
Each one of these predictions, like a uniformly expanding Universe whose expansion rate was faster in the past, a solid prediction for the relative abundances of the light elements hydrogen, helium-4, deuterium, helium-3 and lithium, and most famously, the structure and properties of galaxy clusters and filaments on the largest scales, and the existence of the leftover glow from the Big Bang — the cosmic microwave background — has been borne out over time. It was the discovery of this leftover glow in the mid-1960s, in fact, that led to the overwhelming acceptance of the Big Bang, and caused all other alternatives to be discarded as non-viable.

But there was another prediction we haven’t talked about much, because it was thought to be untestable. You see, photons — or quanta of light — aren’t the only form of radiation in this Universe. Back when all the particles are flying around at tremendous energies, colliding into one another, creating and annihilating willy-nilly, another type of particle (and antiparticle) also gets created in great abundance: the neutrino. Hypothesized in 1930 to account for missing energies in some radioactive decays, neutrinos (and antineutrinos) were first detected in the 1950s around nuclear reactors, and later from the Sun, from supernovae and from other cosmic sources.
But neutrinos are notoriously hard to detect, and they’re increasingly hard to detect the lower their energies are. That’s a problem.
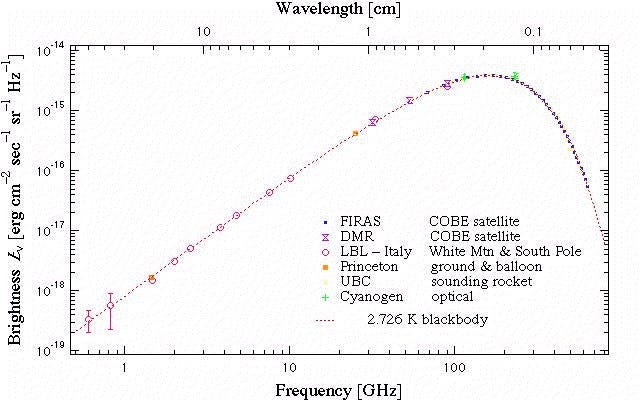
You see, by time we come to the present day, the cosmic microwave background (CMB) is only at 2.725 K, less than three degrees above absolute zero. Even though this was tremendously energetic in the past, the Universe has stretched and expanded by so much over its 13.8 billion year history that this is all we have left today. For neutrinos, the problem is even worse: because they stop interacting with all the other particles in the Universe when it’s only about one second after the Big Bang, they have even less energy-per-particle than the photons do, as electron/positron pairs are still around at that time. As a result, the Big Bang makes a very explicit prediction:
- There should be a cosmic neutrino background (CNB) that is exactly (4/11)^(1/3) of the cosmic microwave background (CMB) temperature.
That comes out to ~1.95 K for the CNB, or energies-per-particle in the ~100–200 micro-eV range. This is a tall order for our detectors, because the lowest-energy neutrino we’ve ever seen is in the mega-eV range.
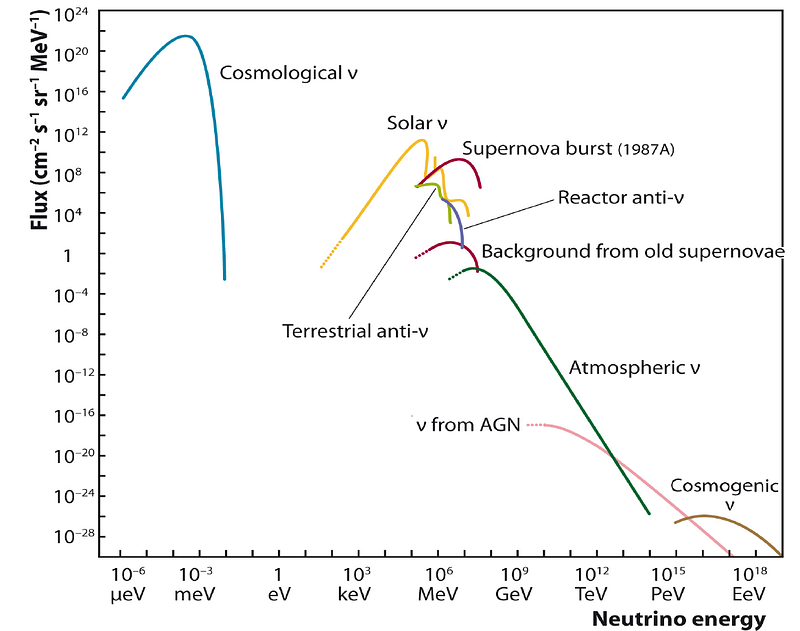
So for a long time, it was assumed that the CNB would simply be an untestable prediction of the Big Bang: too bad for all of us. Yet with our incredible, precise observations of the fluctuations in the background of photons (the CMB), there was a chance. Thanks to the Planck satellite, we’ve measured the imperfections in the leftover glow from the Big Bang.
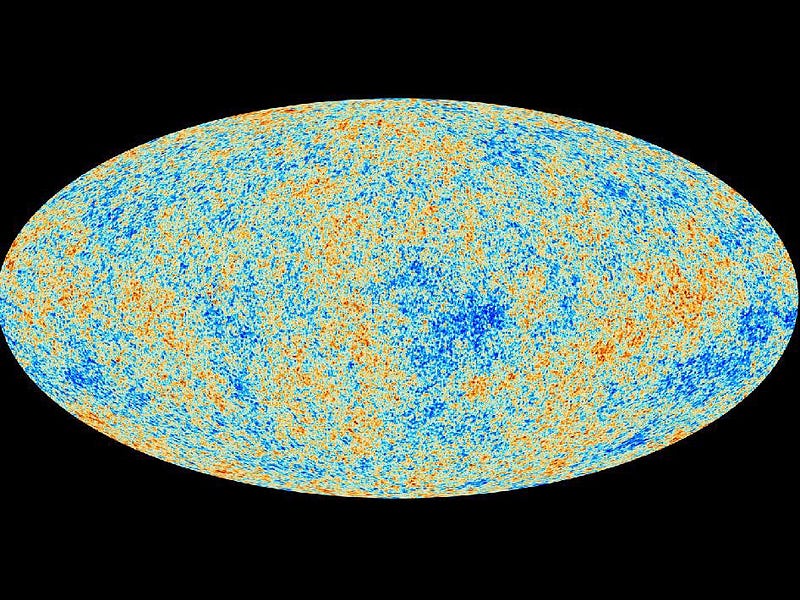
Initially, these fluctuations were the same strength on all scales, but thanks to the interplay of normal matter, dark matter and the photons, there are “peaks” and “troughs” in these fluctuations. The positions and levels of these peaks and troughs tells us important information about the matter content, radiation content, dark matter density and spatial curvature of the Universe, including the dark energy density.
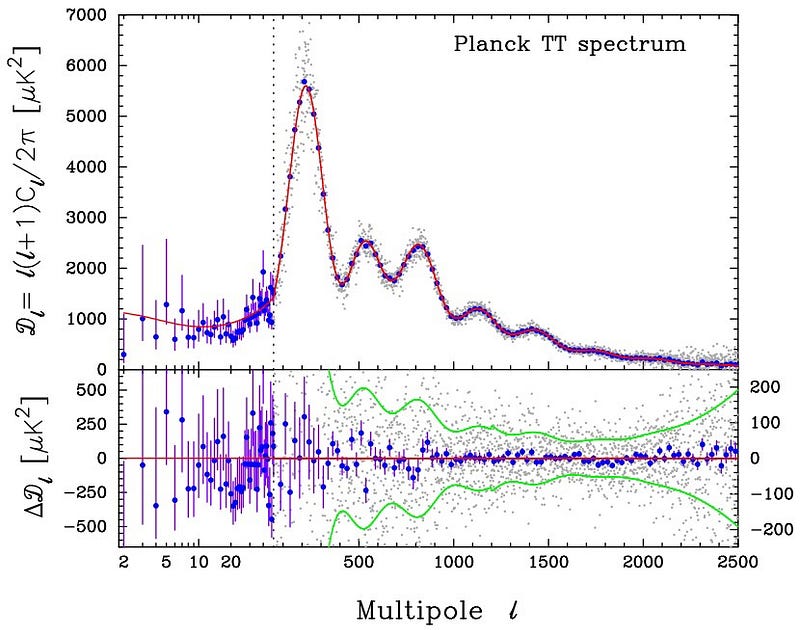
There’s also a very, very subtle effect: neutrinos, which only make up a few percent of the energy density at these early times, can subtly shift the phases of these peaks and troughs. This phase shift — if detectable — would provide not only strong evidence of the existence of the cosmic neutrino background, but would allow us to measure its temperature, putting the Big Bang to the test in a brand new way.
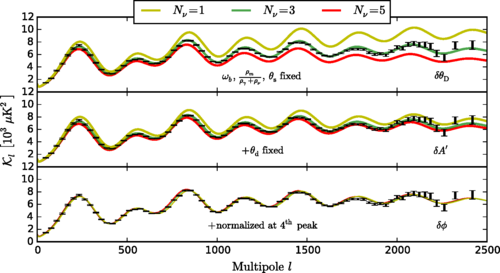
Last month, a paper by Brent Follin, Lloyd Knox, Marius Millea and Zhen Pan came out, detecting this phase shift for the first time. From the publicly-available Planck (2013) data, they were able to not only definitively detect it, they were able to use that data to confirm that there are three types of neutrinos — the electron, muon and tau species — in the Universe: no more, no less.
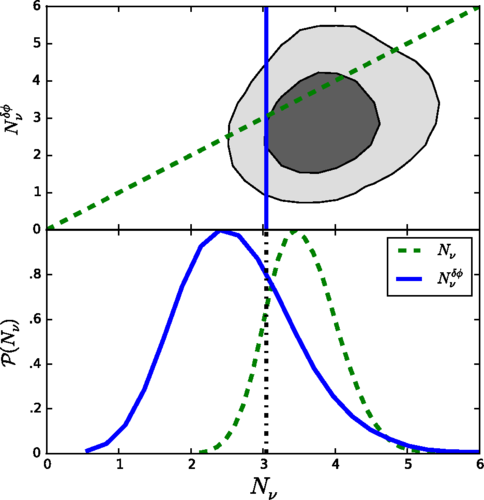
What’s incredibly promising about this is that there is a phase shift seen, and that when the Planck polarization spectra come out and become publicly available, they’ll not only allow us to constrain the phase shift even further, but — as announced by Planck scientist Martin White at the AAS meeting in January of this year — they’ll finally allow us to determine what the temperature is of this Cosmic Neutrino Background!
This neutrino background is definitely there; the fluctuation data tells us this must be so. It definitely has the effects we know it must have; this phase shift is a brand new find, detected for the very first time in this paper. And as soon as the Planck team releases their full polarization data/spectra, we’ll be able to determine — at long last — whether the standard Big Bang picture is right in that final way: in terms of its temperature.
Two degrees above absolute zero was never so hot.
Leave your comments on our forum, and support Starts With A Bang on Patreon!