How fast does the Earth move?
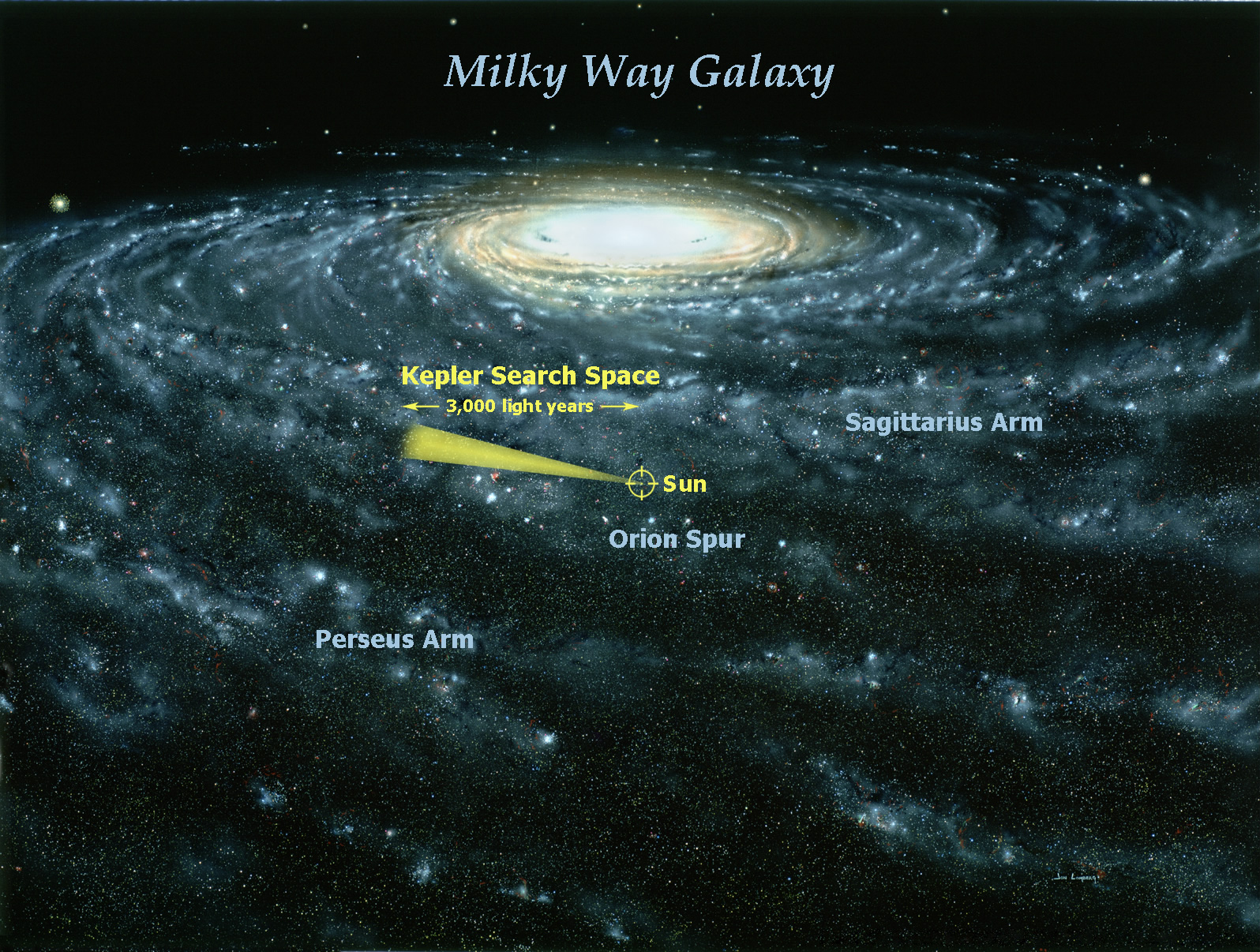
- Although we barely feel it at all, planet Earth is moving relative to every other object in the Universe: through the Solar System, the galaxy, and the Universe at large.
- At each scale, as we zoom out, there's an incredible amount to learn about our motion through the cosmos, and we can even measure what it cumulatively adds up to.
- Whenever you ask how fast we're moving, it's vital to ask, "Relative to what?" After centuries of advances in astronomy, we finally know the answer, on all cosmic scales.
No matter what perspective you choose to look at it from, planet Earth is always in motion. Our planet rotates on its axis continuously, spinning and completing a full 360° rotation approximately once a day. As we spin, we also revolve around the Sun, completing a nearly 1 billion kilometer journey every single year. Moreover, the entire Solar System — Sun, planets, moons, and all — moves through the Milky Way galaxy, orbiting around the galactic center on timescales far greater than humanity has existed for. And finally, the Milky Way galaxy moves within the Local Group, which itself moves through intergalactic space.
Depending on what we’re measuring our motion relative to, we can quantify just how quickly planet Earth moves through the Universe. Even though our motion is barely detectable through the experiments we can perform here on Earth, a look out at the Universe enables us to understand precisely how we’re in motion on each and every scale. Here’s how we know what our cosmic motion is, from each individual component to the entire cumulative effects of everything combined.
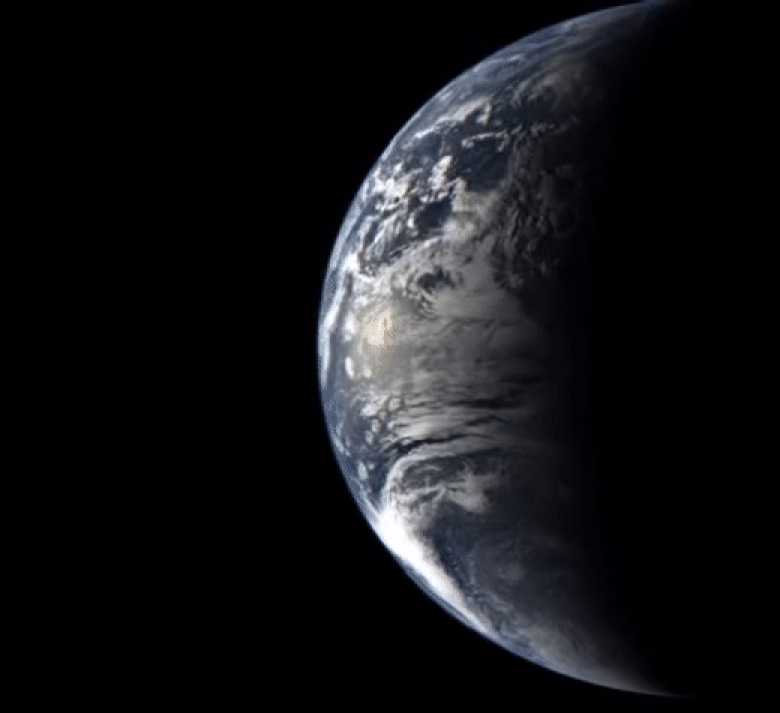
How fast does the Earth spin?
This question, although it might seem simple, has a different answer dependent on where, precisely, you are on the planet’s surface. Planet Earth is a rigid body, meaning that the land masses remain relatively constant with respect to one another over time. As the Earth rotates about its axis, practically every point on the surface completes a full rotation in just under 24 hours: 23 hours, 56 minutes, and 4.09 seconds, to be precise.
The reason we assign 24 hours to the day is because our planetary motion around the Sun — the fact that we don’t just rotate on our axis, but revolve around the Sun as well — changes our position in space relative to where we were when the prior day began. That extra bit of motion means that our planet needs to rotate by slightly more than 360° to go from sunrise to sunrise or sunset to sunset.
It’s often quoted that our planet is around 6371 kilometers in radius, but that’s only on average. In reality, we not only have local topographic features like mountains, valleys, and oceans, but the planet as a whole bulges at the equator and gets somewhat compressed at the poles. The dormant volcano Mount Chimborazo is the farthest point from Earth’s center, at 6384.4 kilometers, while sea level at the North Pole is only 6356 km from Earth’s center.
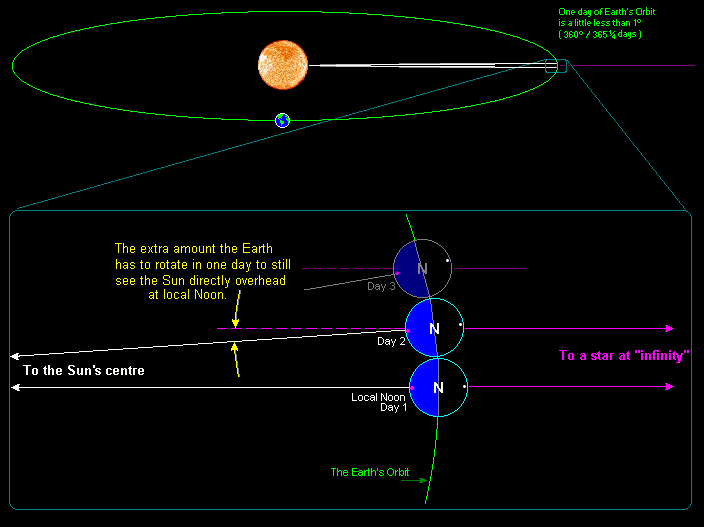
Still, the entire planet completes one 360° rotation in that just-under-24-hour time interval, meaning that someone at the greatest distance from Earth’s center located at the equator — 0° latitude — travels at 1676 kilometers per hour (1042 miles per hour), but the higher your latitude is, the slower you move due to the rotating Earth. Someone at 45° latitude moves at a speed of only 1183 km/hr (735 mi/hr), and someone at either the north or south pole wouldn’t move at all; they’d simply complete a rotation while always remaining at Earth’s geographic pole.
There are some fascinating consequences attached to the fact that Earth is a planet that rotates on its axis.
- The acceleration due to gravity is slightly stronger at higher latitudes, as being closer to the center of the Earth means a stronger acceleration. All told, you’ll accelerate about 0.5% faster at the poles than at the equator.
- The speedier motion of Earth at the equator means that it costs less energy to launch an object into space the closer you are to 0° latitude, which is why terrestrial rocket launches occur almost exclusively in the tropics.
- And the fact that we have tides from the Moon and Sun means that Earth experiences a slight “braking” effect over time. With each passing year, the day becomes ~14 nanoseconds longer than the year before. Extrapolating back to some ~4 billion years ago, in the early stages of the Solar System, our planet spun three-to-four times faster, meaning that a day consisted of only 6-to-8 hours, not 24.
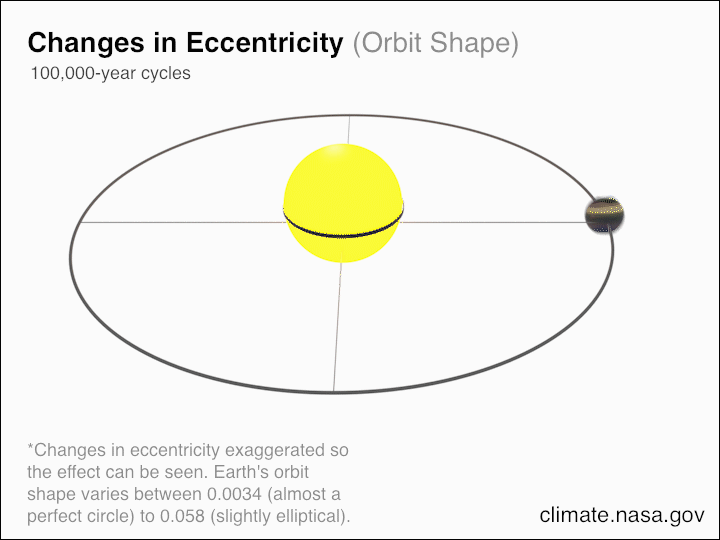
How fast does Earth move around the Sun?
Earth’s rotation gives the fastest point on our planets surface a speed of 1676 kilometers per hour, which translates into about 0.47 km/s. But compared to the speed at which Earth revolves around the Sun, our rotational motion is negligible.
At our distance from the Sun of approximately 150 million kilometers (93 million miles), the mass of the Sun determines how quickly a planet — or any satellite, for that matter — would need to move in order to remain in a stable, nearly circular orbit. That speed comes out to just a whisker under 30 kilometers per second, on average, with our planet’s actual speed varying throughout the year as our planet travels from its closest point to the Sun (perihelion) to it’s farthest (aphelion). At its fastest, Earth moves at 30.29 km/s (18.82 mi/s), while at its slowest, it moves at only 29.29 km/s (18.50 mi/s): a difference of about 3%.
The innermore planets orbit more quickly, with Mercury moving the fastest at around 47 km/s, with each planet orbiting more slowly the farther out we move, all the way down to Neptune at just 5.4 km/s. But just as the Earth’s revolutionary motion around the Sun tremendously outclasses our planet’s rotation about its axis, there are other cosmic motions that far outstrip the Earth’s motion around the Sun.

How fast does the Solar System move through the Milky Way?
If the Sun were more massive, the planets would need to orbit more quickly to maintain their current positions; it’s the nature of gravitation. Well, the Milky Way galaxy itself contains hundreds of billions of stars, and even at our substantial distance from the galactic center — nearly 27,000 light-years — the Sun and the other stars in our vicinity are pulled into elliptical orbits around our galaxy.
To complete a full revolution around the Milky Way takes an incredibly long time: somewhere between 220 and 250 million years, meaning that the last time we were in this position relative to the Milky Way, the first dinosaurs were only just beginning to arise.
It’s estimated that our Sun’s speed around the Milky Way is somewhere around 220 km/s: about seven times as great as our planet’s motion around the Sun. There’s an uncertainty to this figure of around ~20 km/s: we only know how fast we’re moving through the Milky Way to a precision of around 10%. This value, of ~20 km/s, is also approximately the speed at which the nearby stars we observe move relative to us. Of all the different ways that Earth (being part of the Solar System) moves through the Universe, our motion through the Milky Way is the one component with the largest uncertainty.
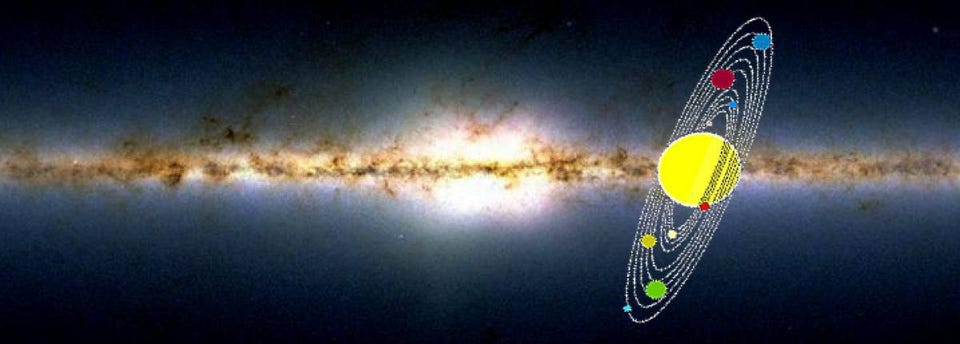
It’s also worth pointing out that the motion of the planets around the Sun is not perfectly aligned with the motion of the Sun and the Solar System through the galaxy. Whereas the Solar System orbits roughly in the plane of the Milky Way — a plane that’s a little over 100,000 light-years in diameter but only approximately ~2,000 light-years thick — the plane in which the planets themselves orbit the Sun are inclined at about 60° relative to our motion through the galaxy.
As a result, our cumulative motion on Earth, through the Milky Way, can vary from as little as 208 km/s to as much as 237 km/s, depending on whether the Earth is traveling in the same direction that the Solar System moves through the galaxy or in the opposite direction; this changes over the course of a year. Although the Solar System itself no doubt moves in an ellipse around the Milky Way’s center, we do not have a good value for how eccentric the orbit is (i.e., how different closest approach is from most distant approach to the galactic center). While it’s likely a bit under 10%, the fact of the matter is that close approaches to other stars, which occur at random a few times every million years, are constantly changing our orbital parameters through the galaxy.
In a messy environment such as this, the old Danish proverb that, “it is difficult to make predictions, especially about the future,” clearly applies.

How fast does the Milky Way move within the Local Group?
The strength of the gravitational force between two massive objects determines how quickly they accelerate toward one another under their mutual influence, and that acceleration, over time, leads to those masses moving rapidly through space. Even as Earth spins on its axis and revolves around the Sun, all while the entire Solar System orbits through the Milky Way, there are larger cosmic motions at play. The one closest to home happens right here in our Local Group, where the Milky Way galaxy is the second largest of perhaps ~60 individual galaxies, all bound together.
The largest and most massive galaxy in the Local Group is Andromeda, located around 2.5 million light-years away and containing perhaps double the Milky Way’s mass. Andromeda is too far away for us to measure precisely how its position changes over time — at least, on human timescales — but we can measure its speed along our line-of-sight to it. Based on the cosmic blueshift of the spectral lines that we observe, we can tell that Andromeda moves toward our Sun at a speed of 301 km/s.
When we factor in the direction and speed with which the Sun moves through the Milky Way, we can determine that the Milky Way and Andromeda are speeding toward one another at 109 km/s; we’re on a collision course that should culminate with a great galactic merger that will begin in about ~4 billion years.
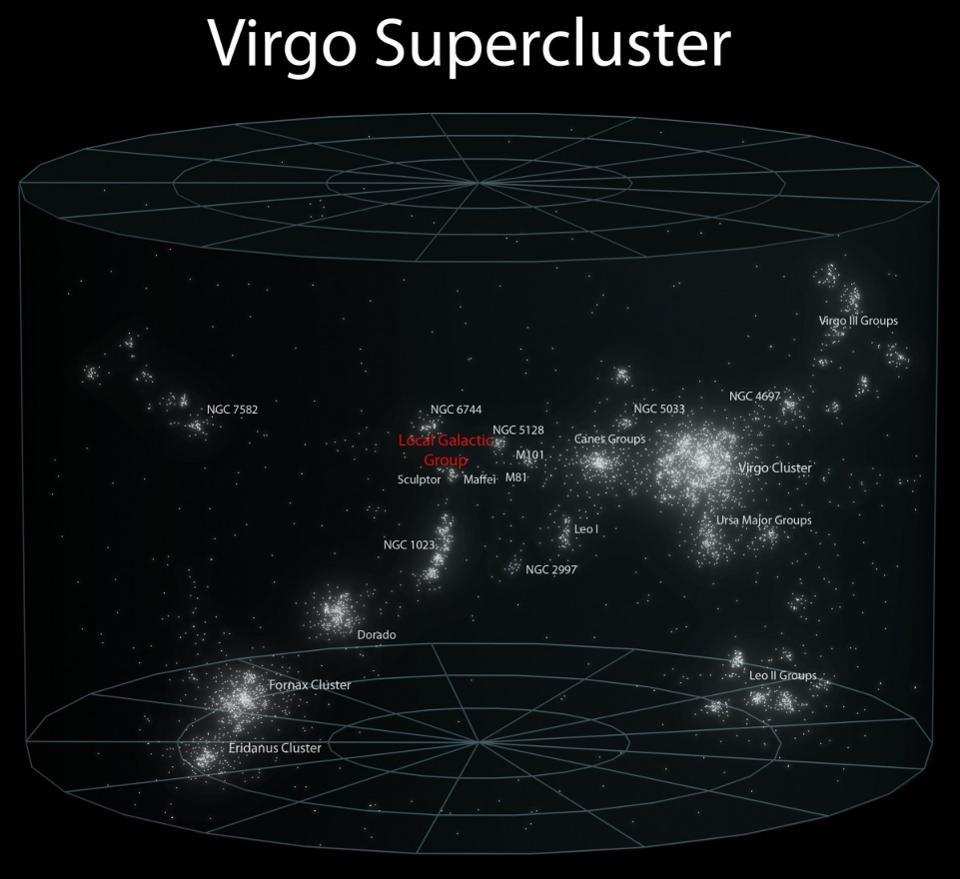
How fast does the Local Group move through intergalactic space?
Finally, there are the grandest scales of all that we can only probe once we map out the full suite of galaxy groups, clusters, superclusters, and even larger structures in the Universe. The other massive objects in our nearby Universe all pull on us, while the underdense regions — the ones that have given up the matter they once possessed to the denser regions around them — pull on us with a force that’s well below the cosmic average, making them, effectively, repellers.
Most of the attractive force around our Local Group comes courtesy of the Virgo Supercluster, which attracts us toward it at about ~300 km/s relative to the rest of our cosmic motion. On the other side of the sky, however, opposing the center of the Virgo Cluster, lies a significantly underdense region of space. Whereas the massive regions are known as clusters, the underdense regions are known as voids, and the void we have effectively repels us from it, also at about ~300 km/s.
With a large, massive cluster on one side of us and a sparse, matter-poor void on the opposite side, the attractive and repulsive forces both point in the same direction, resulting in a net motion of around ~600 km/s for our Local Group.
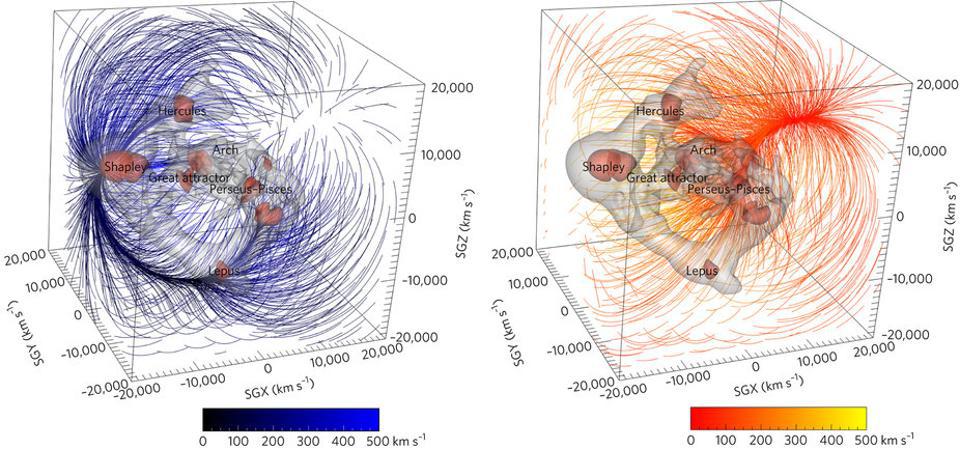
So what’s our total, cumulative motion through space?
When you add all of these motions together:
- the Earth spinning on its axis,
- the Earth revolving around the Sun,
- the Sun moving through the galaxy,
- the Milky Way heading toward Andromeda,
- and the Local Group being attracted to nearby overdense regions and repulsed by nearby underdense ones,
we can obtain a value for how fast we’re actually moving through the Universe at any given instant.
Overall, our total motion through space comes out to about 368 km/s in a particular direction: toward the constellation of Leo. Over the course of a year, this can vary by as much as ~30 km/s, driven largely by the Earth’s changing motion around the Sun. This is confirmed by measurements of the cosmic microwave background radiation, which appears preferentially hotter in the direction we’re moving (toward Leo), and preferentially colder in the direction opposite to our motion (away from the constellation of Aquarius).

Ignoring the variations in our annual motion, we can determine that the Sun moves relative to the cosmic microwave background at 368 km/s, with an uncertainty to that figure of merely ± 2 km/s. When you use the full suite of measurements that we’ve taken, we can calculate the motion of the Local Group with respect to the surrounding large-scale structure of the Universe: 627 ± 22 km/s, moving in the direction of the constellation of Centaurus. The uncertainty in that figure, of ± 22 km/s, is almost entirely due to the uncertainty in the Sun’s motion around the galactic center: the most difficult component of our cumulative motion to measure.
If you want to know how quickly the Earth is moving through the Universe, you have to ask the question, “With respect to what?” Are you asking solely about its rotation? About its motion relative to the Sun? Relative to the Milky Way? The Local Group? The large-scale structure of the Universe? Or the leftover glow from the Big Bang itself: the cosmic microwave background?
All throughout the Universe, nothing is ever static, stationary, and isolated. Today, we know how we’re moving through the Universe on every scale. In the future, when we have a superior map of our own galaxy, we might be able to predict our future motion through the cosmos to precisions that are scarcely imaginable at present.