JWST’s Crab Nebula: Can it solve the mass mystery?
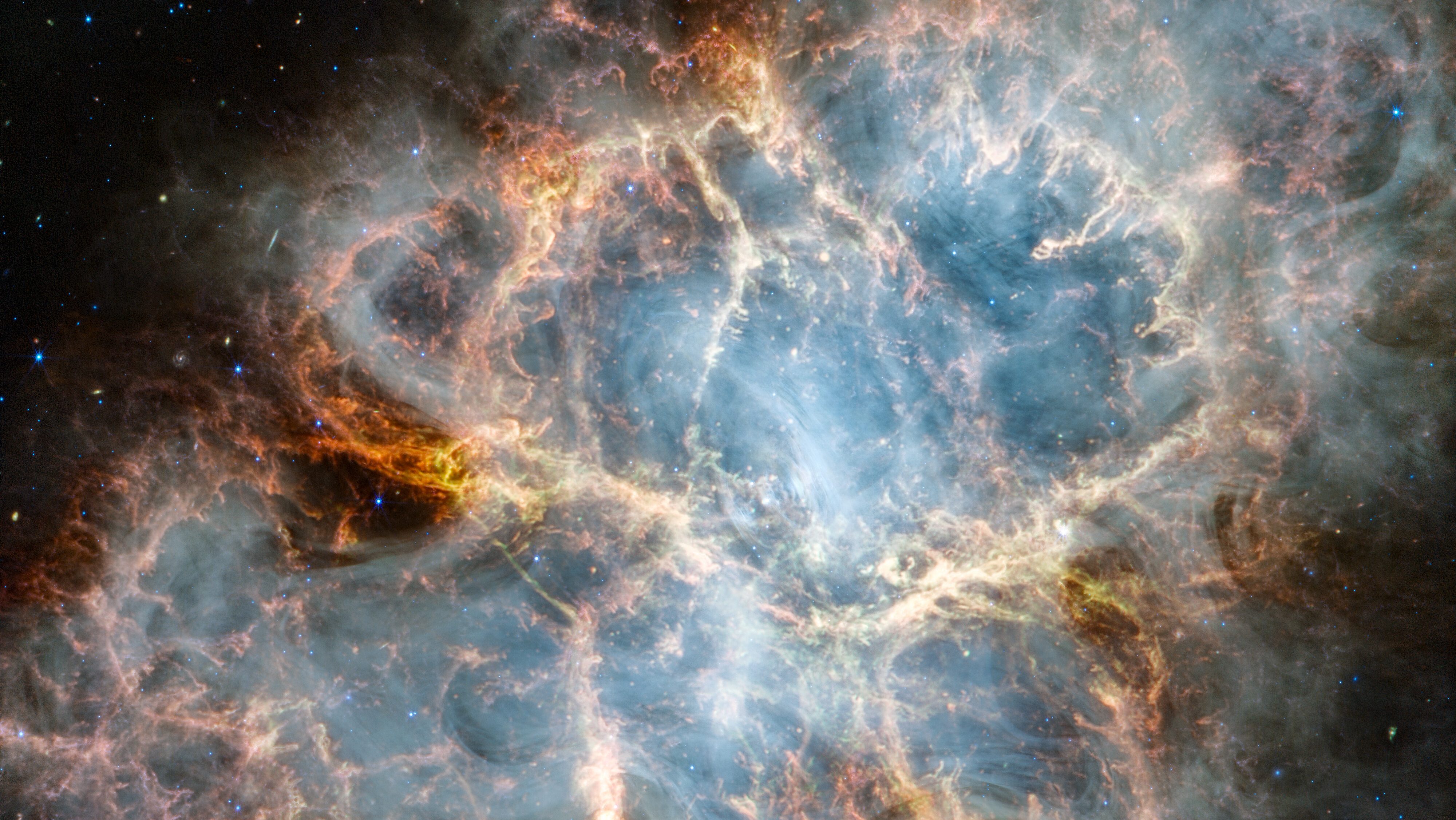
NASA, ESA, CSA, STScI, T. Temim (Princeton University)
- All the way back in 1054, a spectacular sight was seen around the world: a new, brilliant star appeared, remained bright for months, and eventually faded away.
- Hundreds of years later, in the 18th century, what we now know as a supernova remnant was discovered (and rediscovered) in the same region of sky: the Crab Nebula.
- We’ve imaged it, spectacularly, many times ever since. And yet, one enduring mystery — of where all its mass is hiding — remains unsolved. New JWST imaging just might provide the solution.
Nearly a thousand years ago, back in the year 1054, a new star seemingly appeared in the night sky. Outshining all others, including Sirius and even the planets, it was even briefly visible during the day, and then faded away, disappearing for centuries. It wasn’t until well after the invention of the telescope that anyone would see the aftermath of that event: when 18th century astronomers found a remnant of what was a core-collapse supernova explosion lurking in the same region of sky. Over time, as our astronomical capabilities improved, driven by multi-wavelength capabilities, high-resolution telescopes, and instruments capable of tracking details over long periods of time, we finally pieced together what was truly occurring.
Back in 1054, a once-massive star underwent a core-collapse event, dying in a supernova and leaving a pulsing neutron star behind at its center. The remnant that we see today, known as the Crab Nebula, continues to expand and evolve, spanning an impressive 11 light-years across at present. Previous studies have revealed enormous stores of gaseous material ejected into the interstellar medium: about 5 solar masses worth. Combined with the mass of the remnant pulsar, however, a mystery still remains: it should take at least 8 solar masses to trigger a core-collapse supernova, and the material here just doesn’t add up.
Could it be JWST to the rescue? With new imagery from its NIRCam and MIRI instruments, we’re getting a superior view of this nebula compared to all the ones that came before, and new details are already appearing. Could JWST solve this cosmic mass mystery? Let’s dive into the details and see!
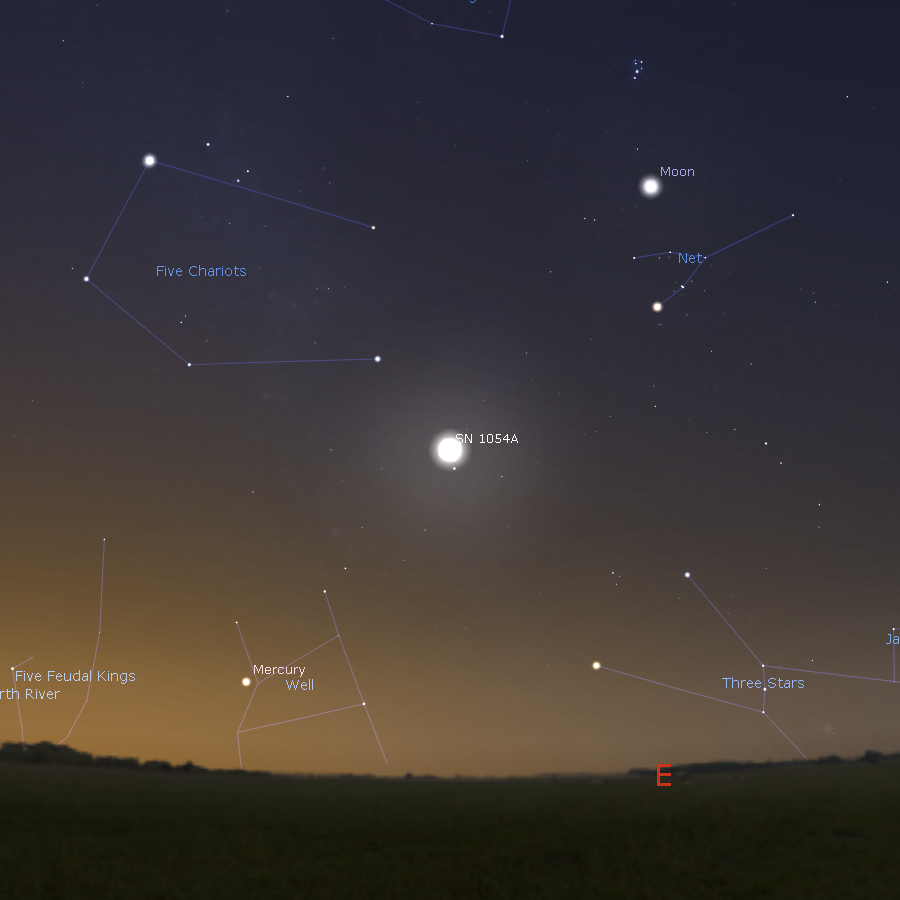
Uncovering the details of the initial explosion required looking back through worldwide records, as no western/European sources recorded it. The first discovered source came from the Chinese empire, where astronomers recorded what they called a “guest star” first appearing on July 4, 1054. Contemporaneously, sightings were recorded in Japan and the Middle East, revealing that this star remained visible for around 2 years, before fading away below the naked-eye threshold. In hindsight, this is fairly typical behavior for a core collapse supernova: swiftly rising to a tremendous peak brightness that’s thousands-to-millions of times the brightness of the original star, then gradually fading away over the span of months-to-years.
Then, hundreds of years later, the remnant of this ancient explosion — although the connection wasn’t made until much later — was discovered by John Bevis: back in 1731. Of course, back in the early 18th century, astronomers weren’t much interested in these fuzzy smudges that appeared in the sky; they were interested in things that were nearby, like planets, moons, and comets. That’s why Bevis’s discovery went largely unnoticed until 1758: when Halley’s comet was due to return. The comet, previously seen in 1456, 1531, 1607, and 1682, was now due to return, as predicted by Edmond Halley back in 1705.
Although Halley had died back in 1742. astronomer Charles Messier had taken up searching for the comet’s return. While searching a particular part of the sky, he accidentally noticed this object, and first confused it for the vaunted comet before realizing his mistake.
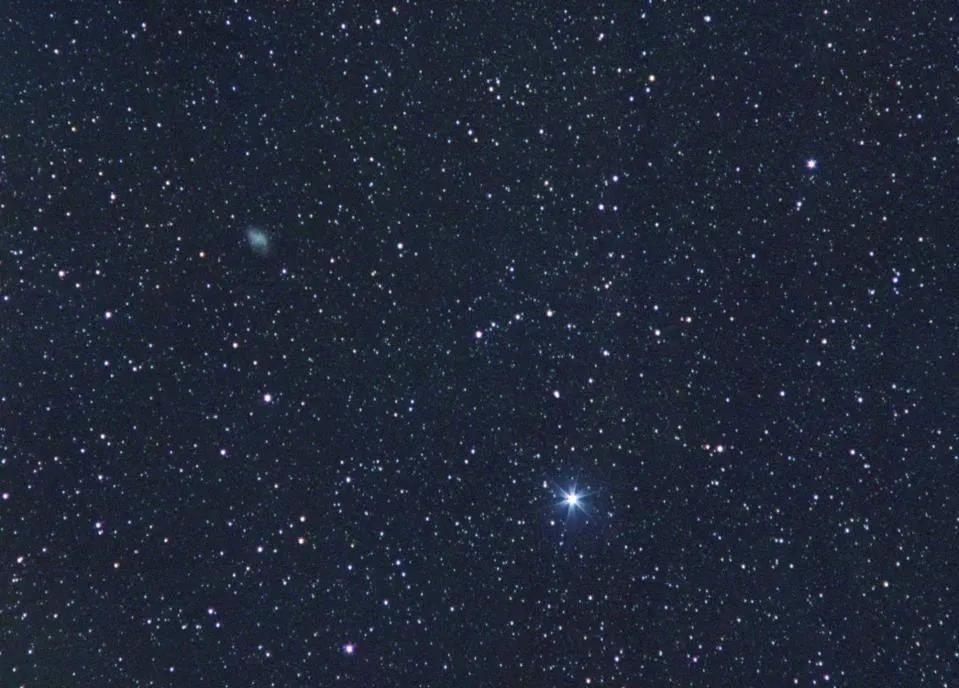
Messier, determined not to let these “permanent” objects in the night sky confuse other comet-hunting astronomers, began creating the famous astronomical catalog of objects that bears his name: the Messier catalog. This object, now known as the Crab Nebula, became the very first object that Messier would catalog, and it still bears the designation of M1: Messier 1. It’s now been an impressive 265 years since its rediscovery, and this nebula remains a fascinating object of study for a large number of bona fide reasons: more than could possibly fit into a single article. However, some of its remarkable properties include:
- it’s one of the closest core-collapse supernovae to occur in modern human history,
- at only 6,500 light-years away, it’s possible to resolve individual features within it, including gas filaments and wind-driven ejecta,
- we can physically see the nebula itself expanding over time,
- and we can determine that its core is powered by a fascinating stellar remnant: a young pulsar, or a neutron star that spins around on its axis an impressive 30 times per second.
This object remains a delight to amateurs and professionals alike, as practically anyone with a telescope can find it and view it for themselves. With off-the-shelf equipment, even a dedicated amateur can measure the expansion of this nebula over decade-long timescales.
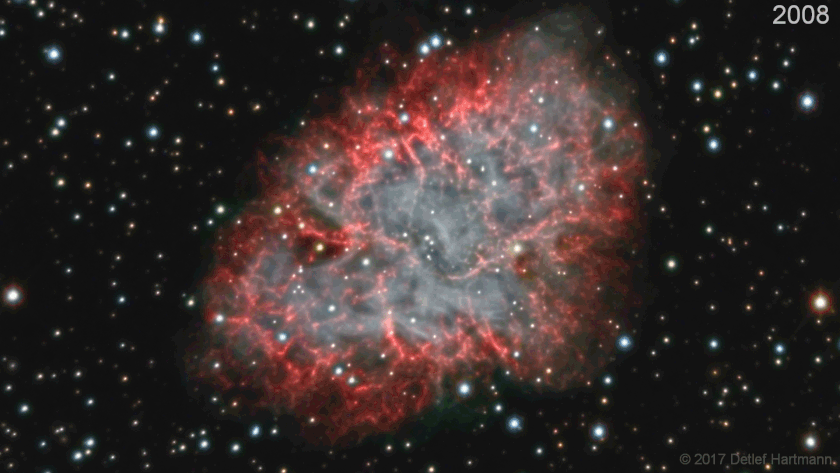
Today, it’s got a remarkable set of properties to it, which have been revealed through a variety of observations that span the full gamut of electromagnetic wavelengths.
- Back in 1054, this supernova reached a peak brightness that saw it shine as bright as 400 million Suns, all combined.
- Now, 969 years after it first detonated, the supernova remnant spans a full 11 light-years across, from end-to-end, with the outskirts still expanding at 0.5% of light-speed: around 1500 km/s.
- X-ray observations, such as those taken by NASA’s Chandra X-ray observatory, are the best at revealing the hot gases and plasmas created by the central pulsar, including how those features change over time in a movie-like format.
- And it’s the innermost regions around the pulsar itself, where relativistic, rapidly accelerating matter is present, that generates winds that transport material and energy to the outer portions of the nebula, driven largely by electrons moving near the speed of light.
The visually stunning filaments in the outer regions, observable in Hubble (visible light) images, only change and grow relatively slowly, as the shocks and instabilities in that region are rather insensitive to short-term changes in the nebula’s overall behavior.
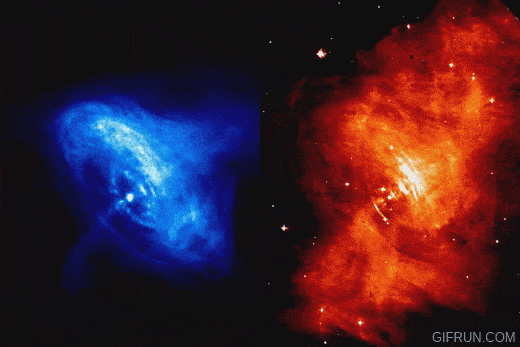
When we take a multiwavelength view of this object, we can see a variety of features, and infer a large amount of information about the physical properties of this supernova remnant and the event that gave rise to it.
- The central pulsar, first discovered only in 1968, is the young neutron star left behind by the supernova event of 1054. The pulsar itself is slowly changing in period, is only about ~10 kilometers in radius, and contains a mass of about 1.4 solar masses.
- The majority of the light coming from the Crab Nebula is far more energetic than what the Sun produces, where it’s actually the brightest X-ray source (above a certain energy threshold) in the entire sky.
- The heated material surrounding the central star also emits a tremendous amount of ultraviolet light; if you added up all the light coming from the Crab Nebula, total, you’d find it’s still 75,000 times as luminous as our Sun, overall.
- Many elements, including hydrogen, oxygen, silicon, and more have been discovered in the Crab Nebula, providing evidence that many of the elements heavier than oxygen but lighter than zirconium are primarily produced in core-collapse supernovae.
- And at lower energies, gaseous filaments, ejected jets of material, and ionized loops of gas appear.
These can be combined into a single composite image, showcasing just how varied and intricate the Crab Nebula truly is.

But even with all this information, there’s still a problem that emerges when it comes to the Crab Nebula: the problem of mass. Astronomers are a big fan of the idea that a star’s initial mass — the amount of mass that it has when it’s born — is what determines its eventual fate. We know that this is largely true, as:
- Stars like the Sun, which generally includes stars between 40% and 800% the mass of the Sun, will burn through their core hydrogen, evolve into red giants, begin fusing helium in their cores, and then will gently die, blowing off their outer layers into a planetary nebula while their cores contract to form a white dwarf.
- The lowest mass stars, which includes stars below 40% of the Sun’s mass, will have time to fully convect: bringing “burned” material out of the core and into the star’s outer layers, while bringing new, hydrogen-rich material into the core. When these stars run out of hydrogen, they won’t get hot enough to fuse helium, leading to a state of slow contraction, ending in a white dwarf.
- But the highest-mass stars, born with 8 solar masses of material or more, will not only ignite hydrogen and then helium burning in their cores, but will go on to fuse carbon, neon, oxygen, and then silicon and sulfur, eventually dying in core-collapse supernovae, leading to a neutron star for the lower-mass varieties and a black hole for the more massive ones.
That’s where the big puzzle arises: there just isn’t enough mass in the Crab Nebula, as inferred by these multiwavelength observations, to explain its core-collapse supernova (and neutron star) fate.
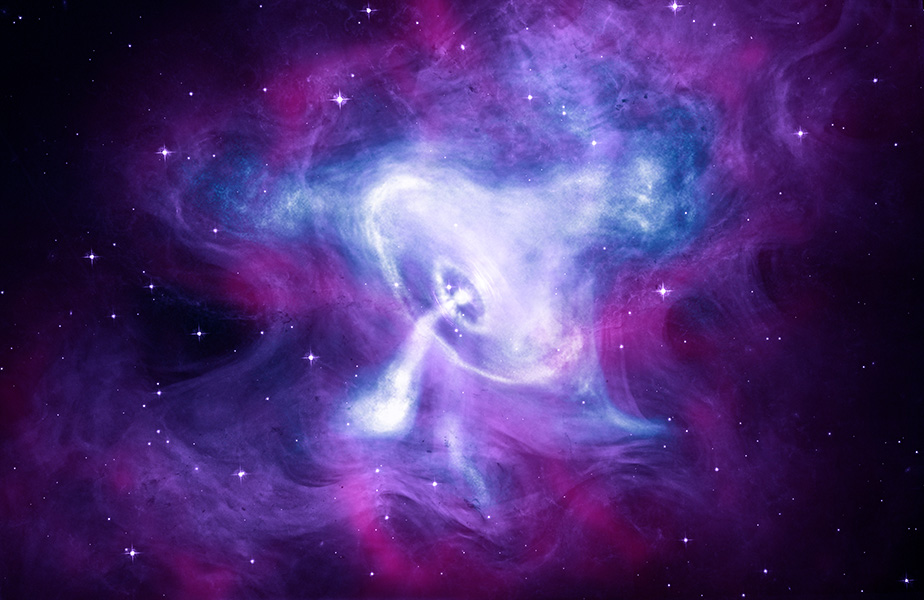
The Crab Pulsar, or the neutron star at its core, comes in at only 1.4 solar masses. From all the previous multi-wavelength data, we’ve been able to constrain the mass of the Crab Nebula (the nebulous material that doesn’t include the central pulsar) to be between 2-and-5 solar masses, with obviously a fair amount of uncertainty there. But observations at greater distances around the nebula, where it’s plausible that a shell of material could have been blown off in earlier stages, reveal a complete absence of any detectable material at all: there’s no shell, plasma, or diffuse gas present, to the absolute limits that our instruments can see.
Even if we take the high-mass value for the Crab Nebula, that still doesn’t give us enough matter/material to trigger a core-collapse supernova! There’s got to be a flaw in our understanding somewhere, but exactly where is a big mystery.
- Could we be modeling the nebula wrong? If so, improved data can help us better estimate the total mass of the Crab Nebula.
- Could we be measuring the mass of the neutron star incorrectly? It’s possible, but not by that much: the most massive neutron star ever found is only slightly heavier than 2 solar masses.
- Could there be material that was ejected long ago that’s now been blown away? Perhaps, but that doesn’t align very well with our understanding of stellar evolution in the late stages of a massive star’s life.
- Could we be misunderstanding the conditions for a supernova? It’s unlikely, but we’ve observed so few in detail that we have to consider it.
Fortunately, we’re about to get an assist: from the full JWST view, now finally available, of the Crab Nebula.
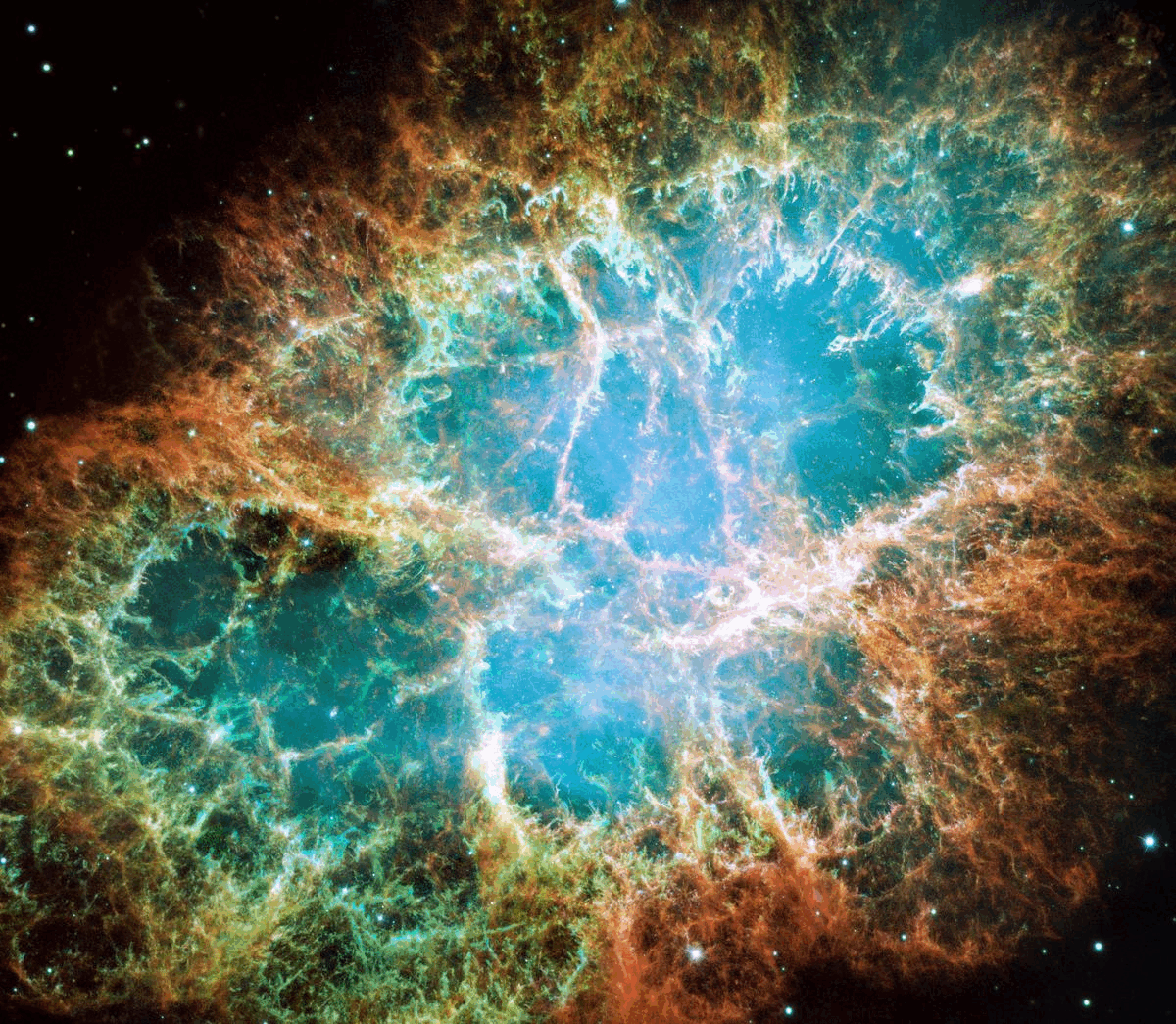
The greatest new detail that’s finally revealed with JWST imaging — something that quite notably, JWST’s predecessor, Spitzer, could not reveal — is the first complete and comprehensive map of the dust distribution within the Crab Nebula. Since the spectral features that reveal individual elements only apply to individual atoms, not to dust grains that may contain those elements, it’s possible that we’ve failed to sufficiently account for dust in previous observations. As you can see, above, the central yellow-white and green filaments that appear in the JWST/infrared image are dust-dominated, and could be incredibly rich in material.
Also in the JWST images, as opposed to the optical Hubble images, you can see what appears as grayish-white “smoke” filling in the interior of the cavity carved by the expanding gases. This isn’t smoke, by any means, but rather a phenomenon known as synchrotron radiation: where fast-moving electrons are accelerated by a strong magnetic field, and the action of that magnetic field causes the electrons to radiate electromagnetic radiation as they pass through the magnetic field. It just so happens that the wavelength range that the synchrotron radiation comes in matches the wavelengths that JWST is sensitive to.
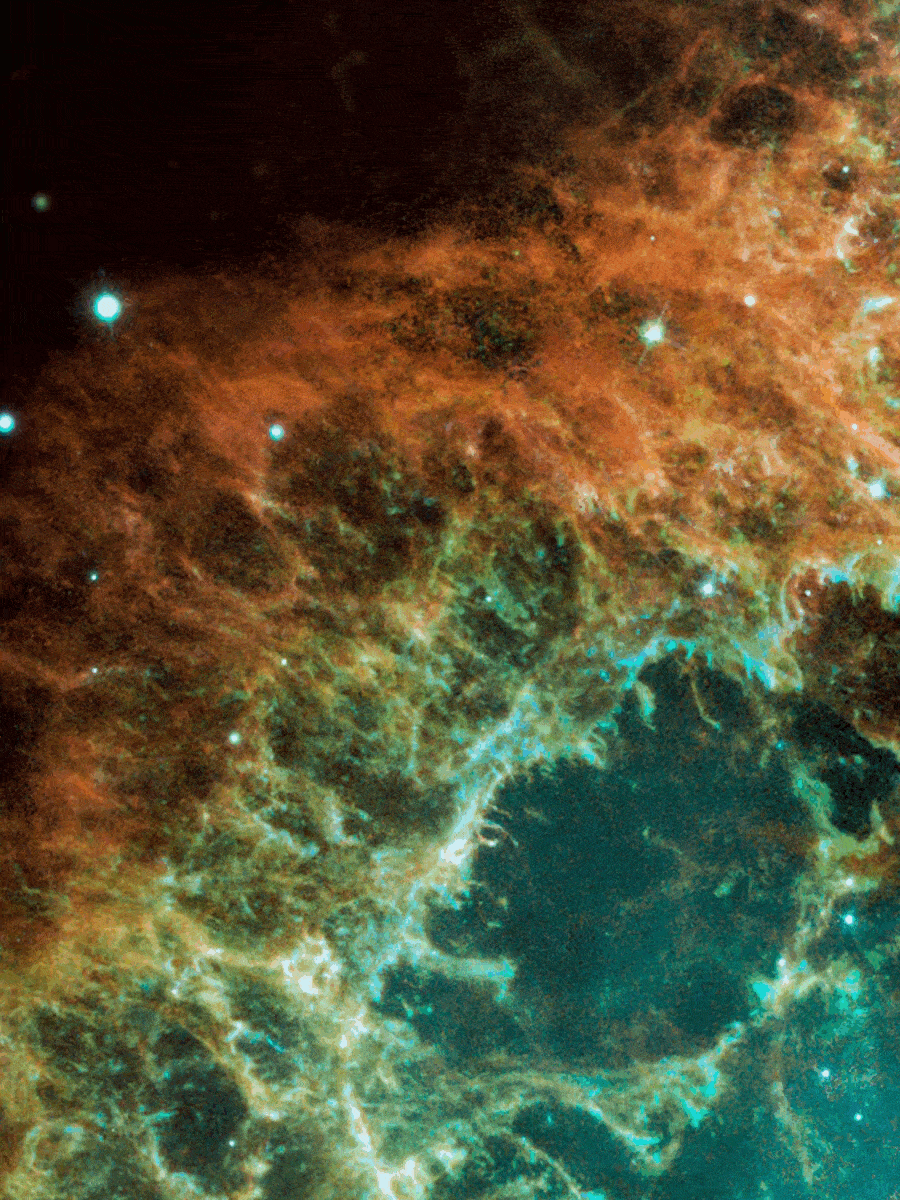
On the outskirts of the nebula, you can see that the smoke-like wisps are curved and pinched: like they’re being funneled into a central disk-like shape. While there are numerous possible explanations for this appearance, one tantalizing one is that there’s a belt of dense gas confining where the supernova’s winds can flow; this is another possible store of massive material that has gone undetected thus far.
There are also hotter, heavier elements revealed by the JWST observations, particularly on the outskirts of the nebula. The red-orange filaments of gas seen by JWST trace out doubly-ionized sulfur atoms, which peter out at smaller distances than the lighter hydrogen atoms that Hubble was sensitive to, farther toward the nebula’s outer edges.
But perhaps most interestingly, there are novel details revealed about the very heart of the nebula: in the region where the pulsar is located. The smoke-like wisps located toward the center trace out the magnetic field lines created by the central pulsar, and you can see many curved wisp-like features all grouped together, indicating the locations where the magnetic field is strongest. This represents material still being transported away from the central regions of the nebula, farther toward the outskirts.
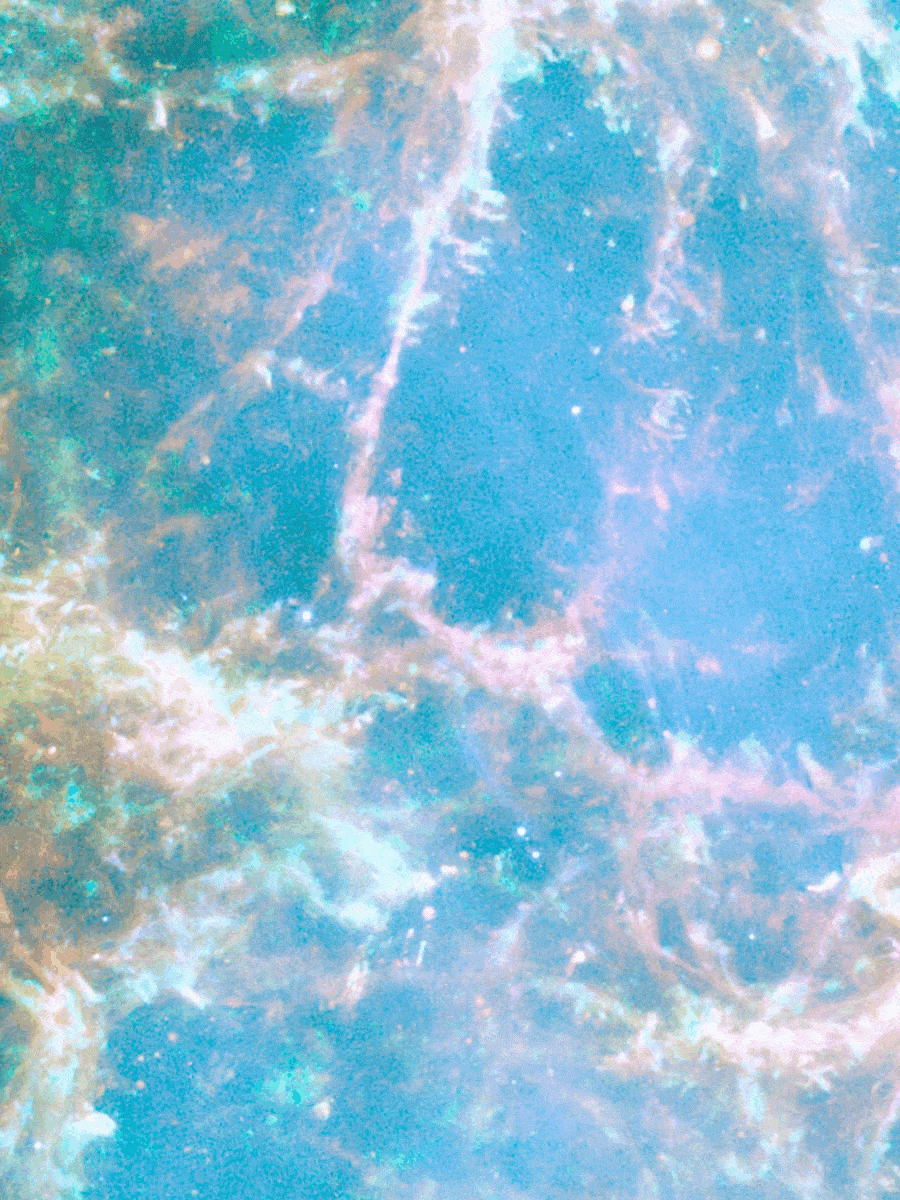
You can also see, by looking at the full-field view of these images, that there’s an asymmetry: the filaments appear to be lengthened toward the upper-right of the pulsar, while simultaneously being relatively shortened in the opposite direction. Although it’s still worth additional efforts investigating this phenomenon, it’s notable that the pulsar itself is moving toward the top-right of the nebula; perhaps the extent of the nebula has something to do with the motion of the central stellar remnant?
Hubble hasn’t taken another look at the Crab Nebula since the early 2000s — more than 20 years ago — but that’s about to change. Just as JWST is observing the nebula now, it’s important to get simultaneous data from Hubble in order to paint a more complete picture of this fascinating region of the sky. Perhaps, with new, superior data from both observatories, combined, we’ll not only be able to map out the various details within it, but come up with a more satisfactory accounting of where all the mass is.
The combination of a central pulsar, ionized plasmas, a wide variety of atoms, dust grains, heated gas, and expanding matter-rich filaments not only makes the Crab Nebula a spectacular sight for almost any observer or observatory, but a scientifically rich place to explore the Universe. As the science papers associated with these images have yet to be published, it’s sure to be an exciting time for anyone who wants to understand the end-stages of a massive, but not ultra-massive, star’s life. After all, this is one of the closest, best-studied examples in all the Milky Way galaxy!