JWST finds free-floating planets in the Orion Nebula?
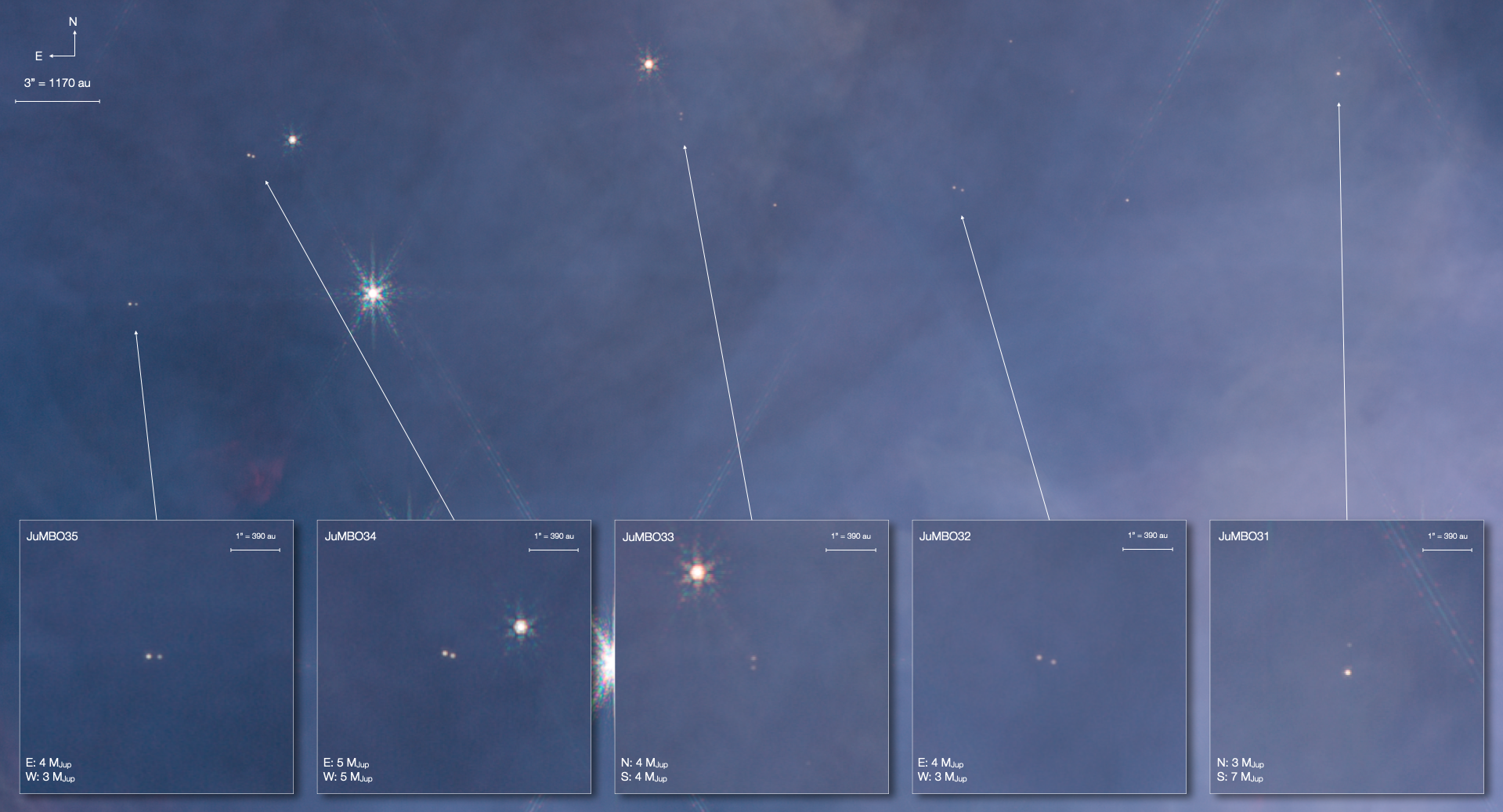
- One of the most studied regions of the sky is the great Orion Nebula: the largest, closest star-forming region to Earth, where thousands of newborn stars, including many with planets, come to life.
- With prior imaging from both space and the ground, we’ve revealed protoplanetary disks, knots of gas, young stellar outflows, and enormous numbers of newly-forming stars.
- But with JWST, we’ve now gone beyond those limits, and revealed hundreds of Jupiter-mass planets in that nebula as well. Our previous best theory of planet formation can’t explain them, suggesting something remarkable.
If there’s one thing that’s almost always true in the science of astronomy, it’s this: whenever you have a new instrument, telescope, or observatory — one that’s more powerful and with new capabilities that surpass all others previously — you’re bound to uncover new details wherever you look, even if it’s peering at an object you’ve viewed thousands of times before. Since mid-2022, when JWST finished its commissioning operations and began observing various aspects of the Universe, it’s revolutionized our views of planets, stars, nebulae, galaxies, galaxy clusters, and the deepest, darkest recesses of the distant Universe.
Recently, however, it turned its attention to the Orion Nebula: the closest large star-forming region to Earth. Located just 1300 light-years away and containing ~2000 times the mass of the Sun, it spans more than a full square degree in the sky, while the densest star cluster within it, the Trapezium Cluster, contains approximately 2800 stars located within 20 light-years of one another.
Remarkably, as detailed in two brand-new papers by Mark McCaughrean and Sam Pearson, they’ve discovered more than 500 Jupiter-mass planet-like objects freely floating within the surveyed region, with a whopping 9% of them in binary systems, making them JuMBOs: Jupiter-Mass Binary Objects. While the discovery of these objects and their abundance were initially a surprise to everyone, it’s very likely that astrophysics holds the answers. Here’s what we should all strongly suspect, based on these new findings.
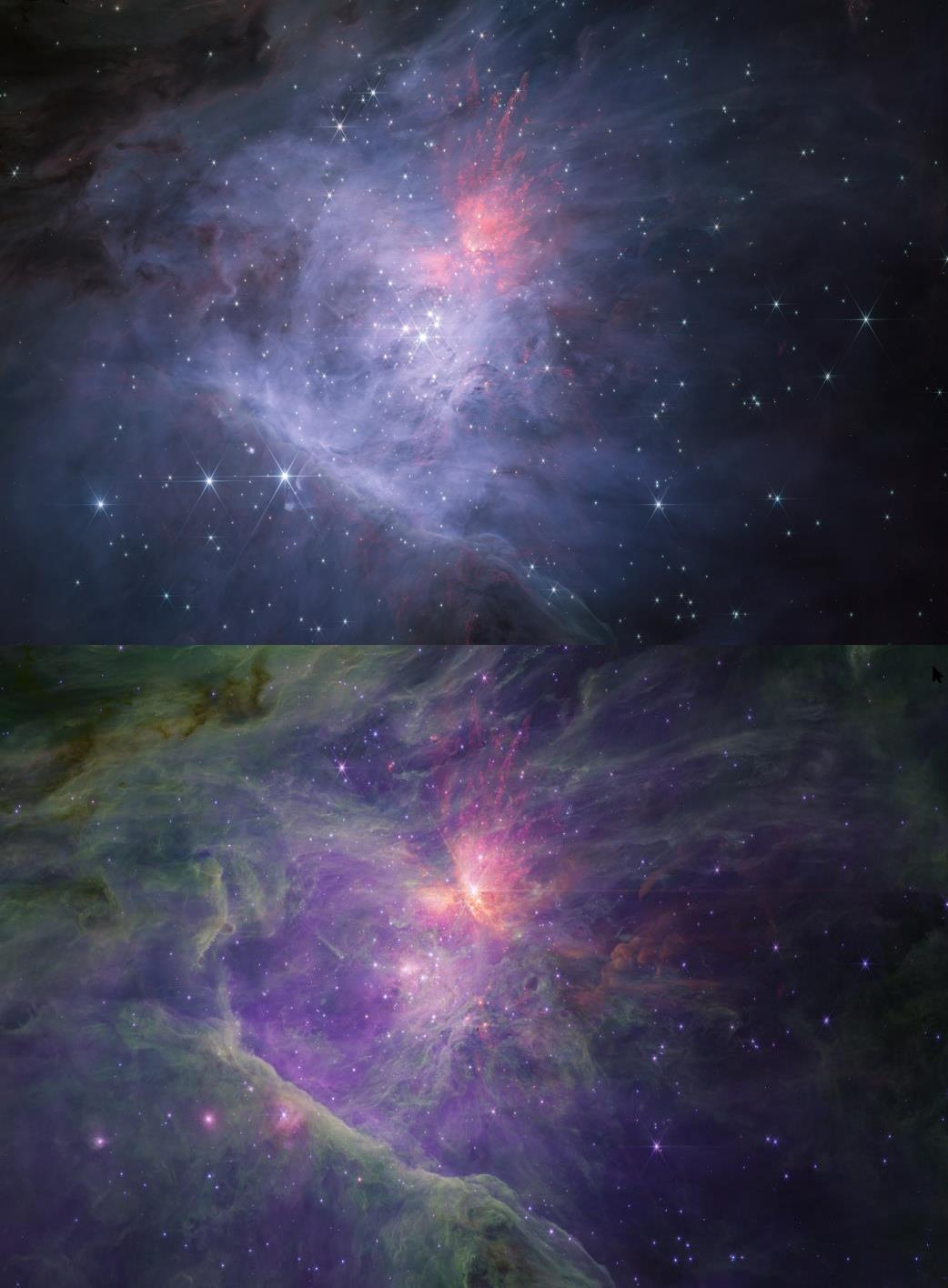
One of the most wonderful aspects of being located where we are in space — on the outskirts of a spiral arm inside a massive, evolved, gas-rich galaxy like the Milky Way — is that we have access to so many things occurring relatively nearby, in a cosmic sense. There’s very little interstellar dust in our location, for example, making it easy to see thousands of light-years in all directions. Within 100 light-years of us, there are thousands of stars, making it possible for us to take a census. Many of these stars have been shown to have planets around them, enabling us to compute which conditions are favorable for stars forming planets.
And, perhaps of very relevant importance, we also see stellar nurseries: regions of space that are in the process of actively forming stars. The Orion Nebula, the closest large star-forming region to Earth, holds the honor of being perhaps the very first astronomical object beyond our own Solar System to be photographically imaged, going all the way back to the early 1880s. Within it are not only newly-formed stars, including many bright, massive stars that are destined to be short lived, but also collapsing clouds of molecular gas, dust-shrouded protostars with protoplanetary (i.e., planet-forming) disks around them, and evaporating gaseous globules, being boiled away by the radiation of nearby, newborn stars.
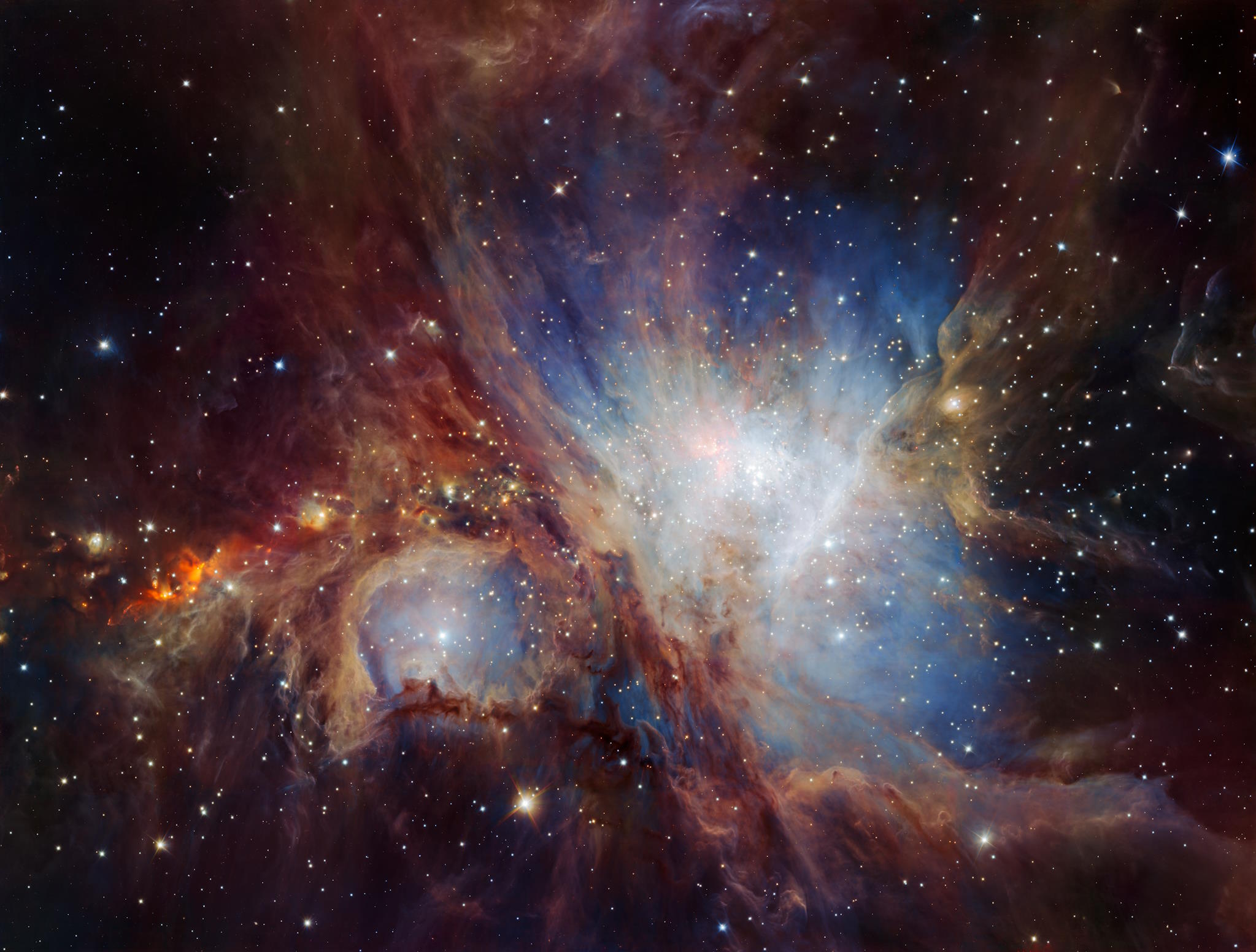
With JWST’s unique capabilities in terms of its infrared reach and sensitivity, as well as its very high energy resolution and spatial resolution, it’s able to resolve details that have never been seen before in a wide variety of systems. Already, it’s looked at nearby stars with protoplanetary disks and seen details we’ve never been able to resolve before, such as an inner disk and the first existence of an analogue “intermediate belt,” found in between where our own Solar System’s asteroid and Kuiper belts lie.
But what would JWST see when it peered deep inside the Orion Nebula, where thousands of new stars, dozens of protoplanetary disks, and copious amounts of light-blocking dust had already been discovered?
One exciting new feature of JWST is its ability to observe at high spatial resolution: something previously only accessible to very large space-based observatories, like Hubble, or extremely large ground-based observatories. However, unlike any of them, JWST is optimized for infrared observing. Not just “a little bit into the near-infrared,” which is what Hubble and most ground-based observatories can do, but over the full suite of near-infrared wavelengths: from 0.7 microns all the way to 5.0 microns, as well as (with a different instrument) in mid-infrared wavelengths (from 5.0-28 microns).
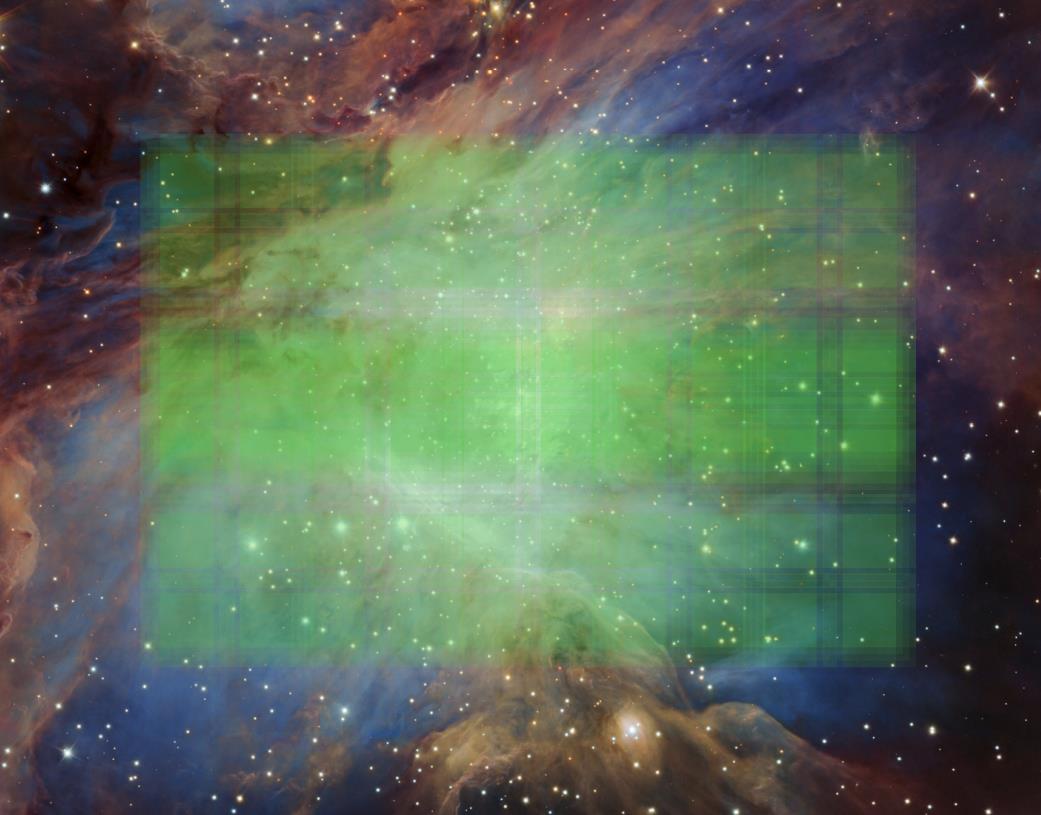
These recent studies that were just performed of the Orion Nebula — studies that were still part of JWST’s first-year science program — focused on a narrow portion of the Orion Nebula, centered on the dense Trapezium cluster and is home to some of the youngest stars known: stars whose median age is only about 300,000 years. For most of human history, the majority of the Trapezium cluster has been obscured by dust.
However, dust is remarkable for a number of reasons, and one of those reasons is that it doesn’t block all wavelengths of light equally. Dust grains, being of a relatively small size (on average), preferentially excel at blocking shorter, bluer wavelengths of light. Red light (at ~700 nanometers) more easily passes through a cloud of dust than blue light (at ~400 nanometers), and so one of the observational effects of dust is what astronomers call reddening.
However, another feature of dust is that it becomes even more transparent to longer wavelengths of light. At 2.0 microns, dust blocks a much smaller portion of the light than it does at 0.7 microns. At 3.5 or 5.0 microns, the light-blocking effects of dust are even smaller, allowing more and more of the light at those wavelengths to pass through. This makes it easier to view a number of important details: warm gas, future sites of star-formation, protostars, and even brown dwarfs and gas giant planets.
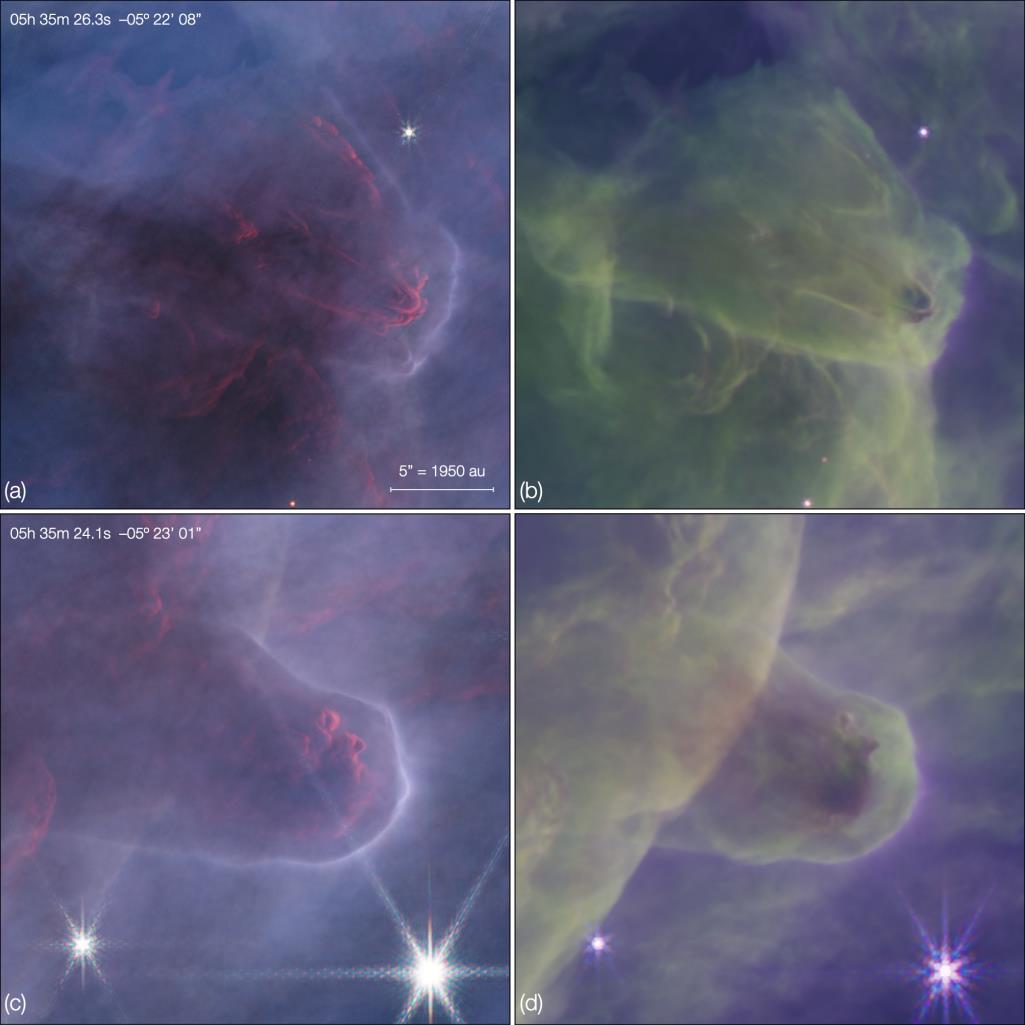
This last fact is generally underappreciated by the general public. Because we’re accustomed to seeing the Universe in visible light, our normal way of thinking is:
- stars emit light,
- other objects in space absorb and/or reflect that starlight,
- and so what we see is a combination of emitted, reflected, and blocked (or “extincted”) starlight.
This is generally true for visible light, as non-stellar objects are only very rarely hot enough to emit visible light: in the wavelength range from 400-700 nanometers (0.4-0.7 microns).
However, the farther we go into the infrared, the better we get at seeing “cooler” objects, which emit their own, longer wavelengths of light. When JWST photographed Saturn, it completed its portrait of the gas giants in our Solar System, from Jupiter through Neptune, inclusive. However, the four worlds appeared very different from one another for a very good reason. On Saturn, Uranus, and Neptune, the features that JWST saw were mostly due to reflected sunlight: from clouds and ices in their atmospheres and rings. However, Jupiter is a different story; owing to its large mass, Jupiter possesses a substantial amount of internal heat, even more than 4.5 billion years after it formed. Unlike the other giant worlds in our Solar System, part of Jupiter’s infrared light comes from the fact that it emits its own heat: substantial enough, in fact, to appear visible to JWST’s eyes.
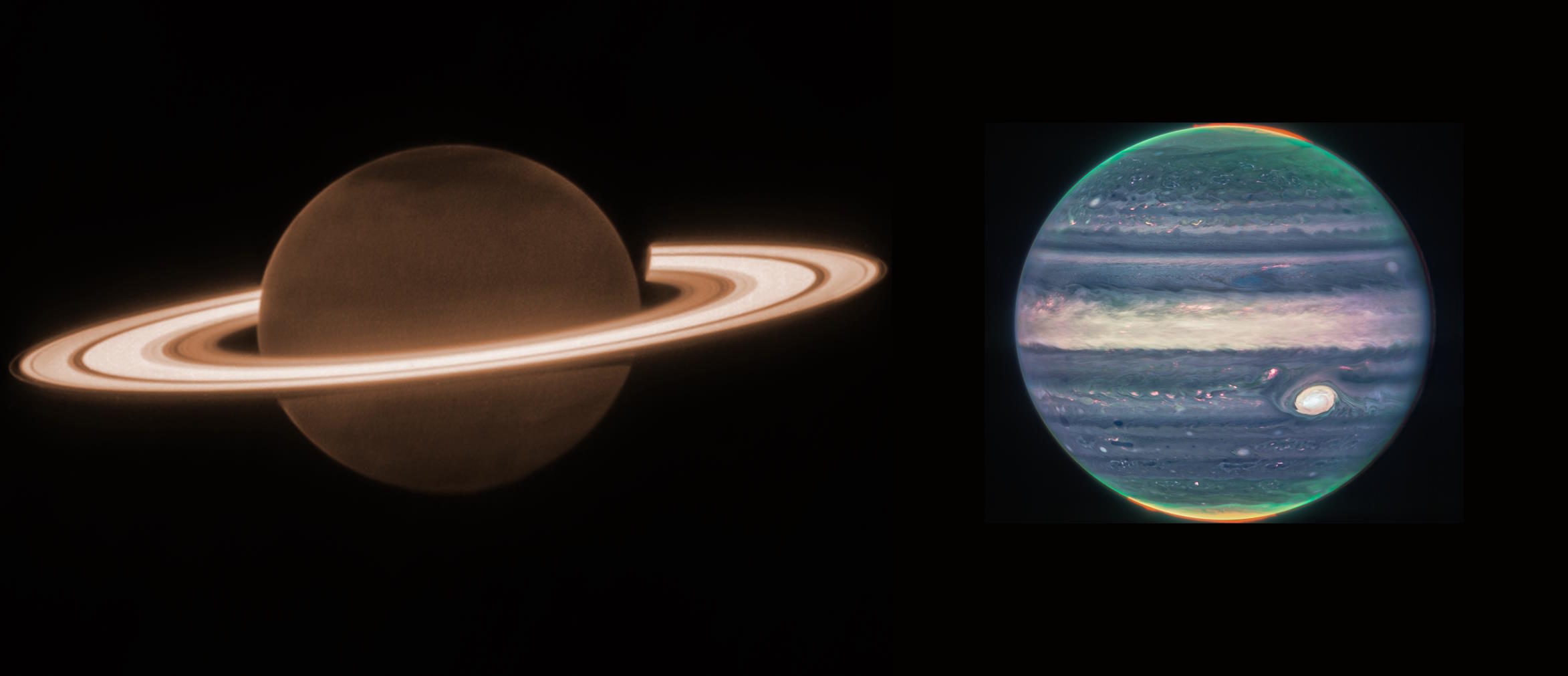
What this means is that, in observing this portion of the Orion Nebula in many different infrared “filters” of light, JWST will be sensitive to a wide variety of objects, including some classes of objects that were inaccessible to all prior generations of telescopes. It will see:
- full-fledged stars,
- protostars with protoplanetary disks around them,
- brown dwarfs (i.e., failed stars) that are burning deuterium, but not hydrogen, in their cores,
- and sub-stellar objects that produce their own infrared light,
among the other knots of gas and dusty features that are so common in nebulae like these.
The key to telling “what’s what” is simply to observe at a series of different wavelengths, including at a variety of short infrared wavelengths and a series of longer infrared wavelengths, as objects emit different amounts of light dependent on their temperatures, and temperature — at least, for objects that emit their own energy — is a direct function of mass. It therefore becomes possible, assuming we correctly understand the relationship between mass, temperature, and emission at different wavelengths, to use JWST to not only identify various types of stars, brown dwarfs, and giant planet-mass objects, but to distinguish them from one another, even within a location as complex and distant as the Orion Nebula.
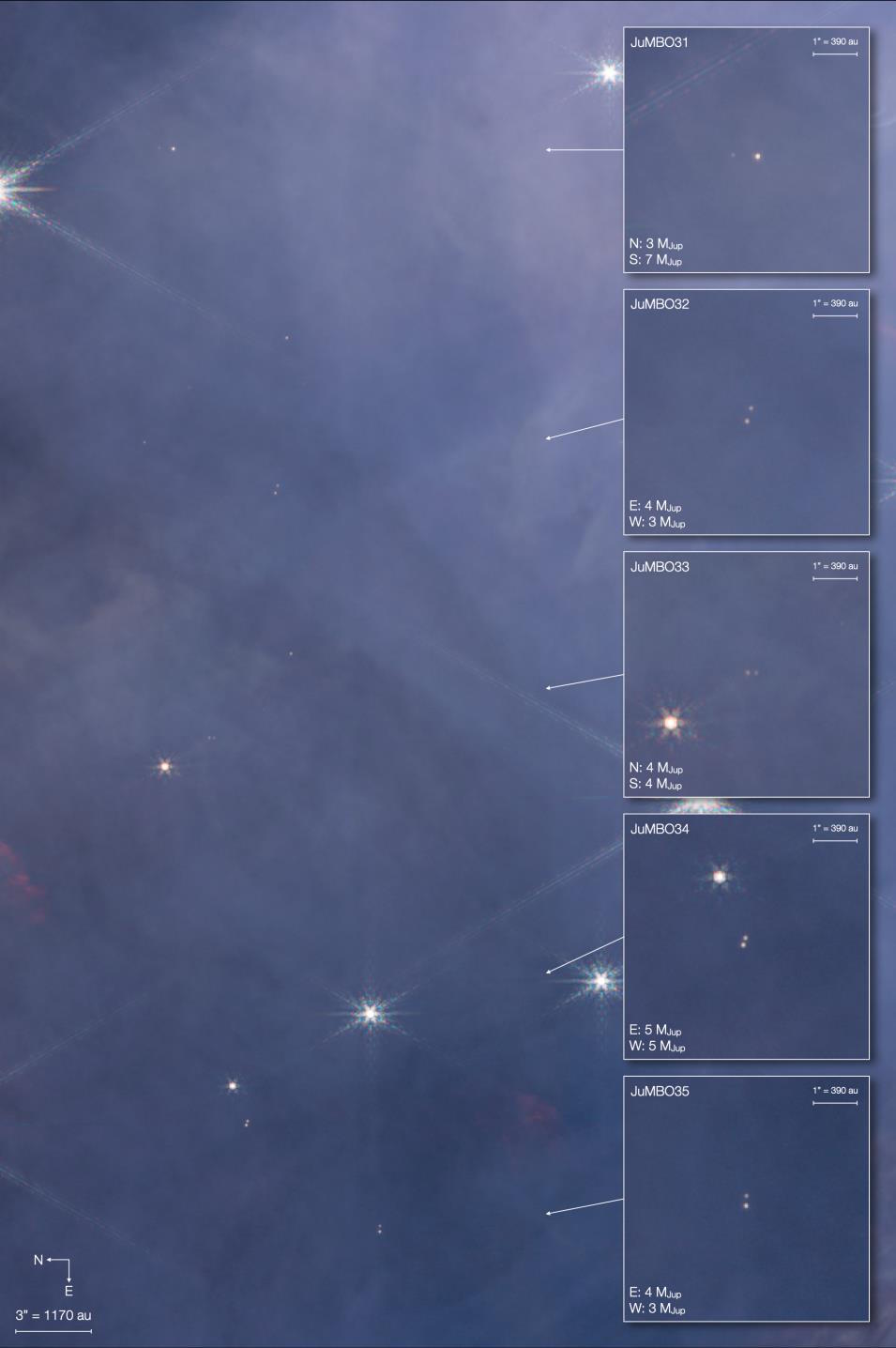
Although they certainly found a lot of expected things in this region of space, there was a tremendous surprise in there: a brand new class of object that the authors call JuMBOs: Jupiter-Mass Binary Objects. All told, about 540 Jupiter-mass objects were spotted, ranging from about 0.6 Jupiter masses, roughly the lower limit of what JWST can identify, all the way up to around 13 Jupiter masses, or the approximate line between the most massive planet and the least massive brown dwarf. This represents a lot of planets; far more than simulations and previous models of star-forming nebulae would have predicted.
But what’s even more surprising is that a whopping 9% of those objects are found to be in wide-binary systems, a much greater fraction than one would expect. The researchers themselves call this, “a result that is highly unexpected and which challenges current theories of both star and planet formation.”
However, it may not be such a surprise, after all, if we consider not only the most common two means of planet-formation, known as the “disk instability” and the “core accretion” scenarios, but a third option that is expected to arise wherever new stars form, but has never been observed directly before: one where protostar and protoplanet formation is abruptly cut off, as gravitational collapse of matter loses the race against the forces of evaporation of star-forming material due to the ultraviolet radiation from nearby stars.
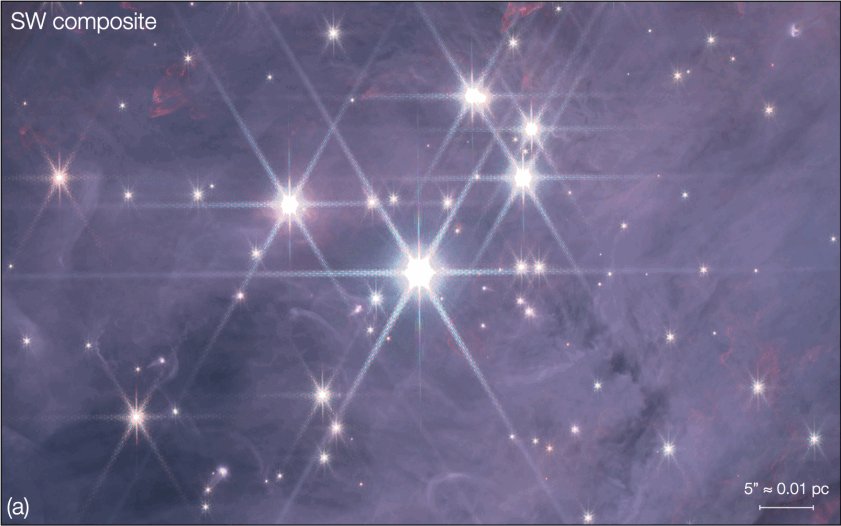
If all you have is a cloud of molecular gas, the way star-formation proceeds is typically as follows.
- The gas cloud will fragment, collapsing into contracting clumps throughout.
- Where densities rise the highest the fastest, new protostars begin to form.
- These protostars generally acquire protoplanetary disks around them.
- Within each disk, gravitational imperfections arise, grow, and lead to protoplanetary cores.
- The largest protoplanetary cores accrete into planets (or even protostars) themselves, and can develop their own circumplanetary disks where lunar (or planetary) systems will form: the “core accretion” scenario.
- At even larger distances from the central protostar, gravitational instability and the rapid, early collapse of matter can create giant planets or even additional stars: the “disk instability” scenario.
- And then, from all the newborn stars, cumulatively, large amounts of ultraviolet radiation are emitted.
- This radiation boils away the collapsing matter around the contracting clumps that collapsed more slowly or grew from smaller initial density imperfections.
- And, when enough material is boiled away, whatever “stunted” state of growth the system was in at that time will be all that remains.
It is very likely that it isn’t “ejected giant planets” that comprise all of these Jupiter-mass planets, but rather that a substantial fraction of them are these boiled-away, failed stellar systems. And it’s even more likely that the “failed stellar system” explanation is the culprit behind most, or perhaps even all, of these JuMBO-class objects: the Jupiter-Mass Binary Objects seen by JWST.

One of the reasons this is surprising is that you can calculate, from simulations that involve gravity, gas, feedback, radiation, and a host of other contributing factors, what the “minimum mass” of a system needs to be to initiate that first step of “fragmentation” from an initial molecular cloud of gas. The answer, although it’s perhaps a naive answer, is typically around 3-to-5 Jupiter masses.
So how, then, is it possible that we’re seeing Jupiter-mass objects, including an increasing fraction of binary objects at lower masses, all the way down to just 0.6 Jupiter masses?
The answer could very well be that star-formation is a great cosmic race, and in this race, there’s a tremendous difference between the 1st place finisher and the 1000th place finisher, and that the 10,000th place finisher — in an environment like the Orion Nebula — is unlikely to even get a chance to finish the race. Instead, what’s likely to occur is that once sufficient numbers of massive enough stars begin producing large enough amounts of ultraviolet radiation, those early-stage protostars, the ones that had yet to grow to a large enough mass to initiate nuclear fusion in their cores (or even, realistically, to get close), find their gas reserves depleted from the outside-in. They simply run out of matter, and come to permanently exist in whatever embryonic state they were in at the moment their stellar nurseries were blown away.
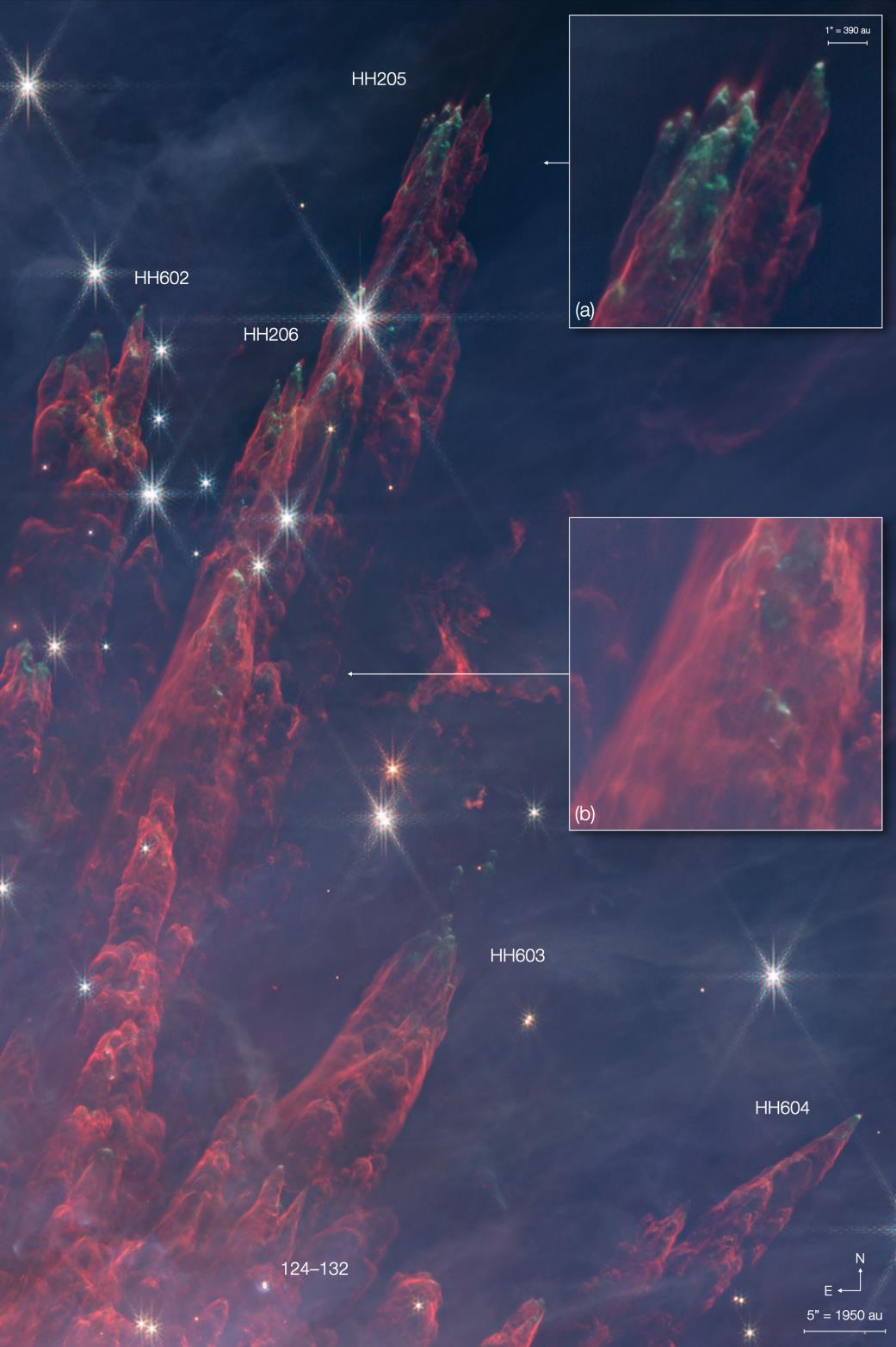
It’s still healthy speculation at this point, as this new class of object has never been seen before, and the critical steps that result in their formation have not yet been observationally identified, but these JuMBOs may be the direct result of a new class of planet: not the rogue planets that were ejected from a star system that was in the process of forming planets, but rather the seeds of what would have been a star, if not for the violence of their environments. In other words, whereas ejected planets are orphans, ripped from their parent star(s) through gravitational violence, many of these Jupiter-mass planets, including the JuMBOs detected here, are the remnants of an abortive process that simply prevented them from growing up into a full-fledged stellar system of their own.
The good news is, with longer-term observations of the more nebulous regions that are undergoing active star-formation — including within the Orion Nebula but also in other locales within the Milky Way — we should be able to gather the necessary observational data to see precisely how and from where these objects, as well as all objects formed in these regions, arise. For right now, however, we have no choice but to accept the observational data for what it is at face value: a puzzle. After all, finding a plausible mechanism by which something can arise is easy. Demonstrating that it’s actually the mechanism at play is the hard part.