There’s A Debate Raging Over Whether Dark Matter Is Real, But One Side Is Cheating

Dark matter feels fake. MOND sounds plausible. What should you conclude?
Imagine I told you that everything you ever saw, touched, or experienced — in this world and in the Universe beyond — was just a tiny fraction of the matter that’s out there. That for every particle of normal matter that existed, there was at least five times as much, mass-wise, of a new form of invisible matter that we’ve never directly detected. And that beyond that, the Universe also contained a mysterious form of energy that caused distant galaxies to suddenly speed up and accelerate away from us some six billion years ago. When all was said and done, all the normal stuff was just 5% of the grand total.
You’d wonder if we didn’t have something fundamentally wrong. If we hadn’t goofed something fundamental, like our theory of gravity. This is the heart of the debate over the existence of dark matter. But before you pick a side, tempting though it is, let’s think about the problem.
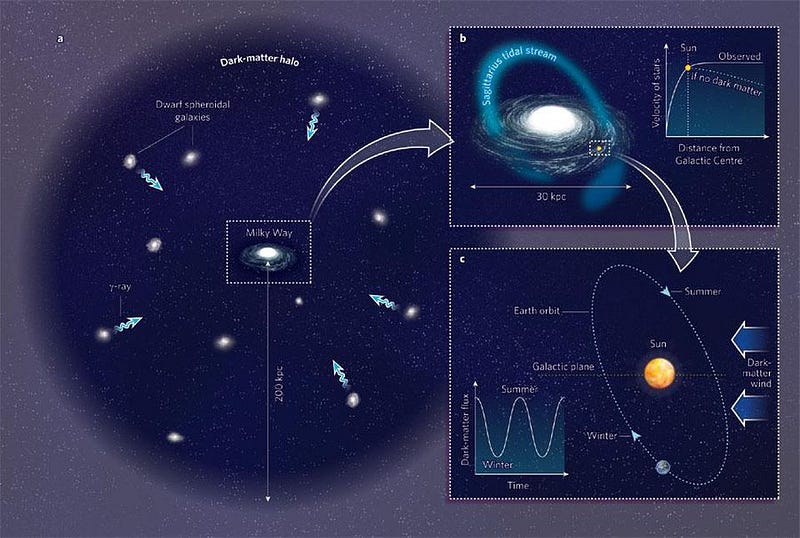
When it comes to any endeavor involving the physical world, the goal is to arrive at the best scientific truth that you can. This is different than what we normally mean when we speak about “truth,” where we mean making only factual statements and not telling any lies. A scientific truth goes deeper than that: it’s the best description of reality we can come up with to explain the full suite of evidence available. That word I just used, description, is of paramount importance. A scientific truth will accurately describe every phenomenon relevant to it. If the idea behind the truth — the overarching framework, model, or theory — is particularly strong, it can even make new predictions about phenomena we haven’t yet observed. It can tell us what to go out and look for.
But we have to be particularly careful, when we test it, that we’re actually testing the relevant predictions and not some confounding factor. If I took a sheet of paper up to the top of a tall building and let it go to test the theory of gravity, I’d be performing a lousy test. In the presence of Earth’s atmosphere, there would be additional forces (like the drag force) other than gravity at play, and they’d throw off my results. I wouldn’t find that the acceleration due to gravity was a constant, because the gravitational force wouldn’t be the only relevant one. If I wanted to perform that test more accurately, I’d need to design an experiment that either minimized the drag force, relative to gravity, or eliminated it altogether.
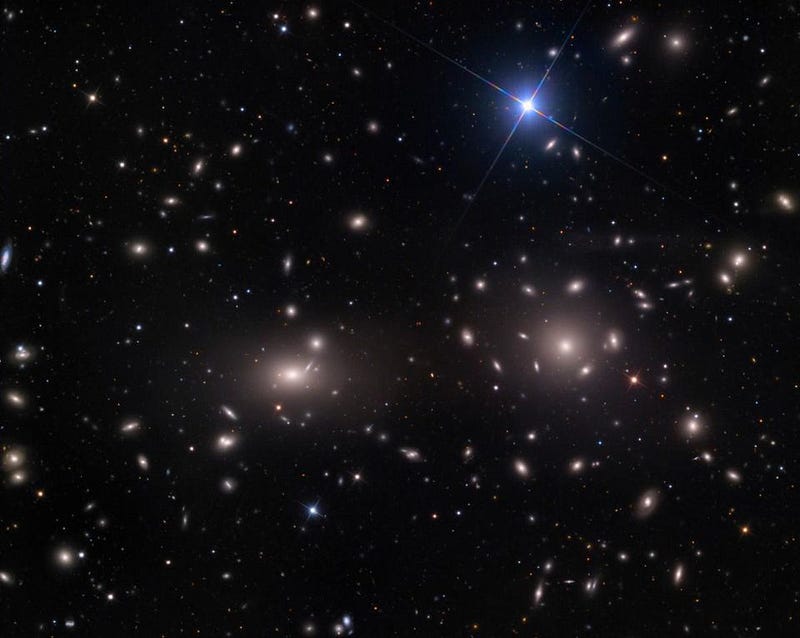
When we take a look at the “dark matter problem,” there are two observations that led us to understand this was a real concern.
- In the 1930s, Fritz Zwicky measured the motions of individual galaxies in the Coma Cluster (above). By estimating the mass from stars, he came up with a figure for the mass of the cluster. By measuring the motions of the galaxies themselves, he could derive what the mass needed to be to keep the cluster gravitationally bound. When the two measurements didn’t match, and more gravitational mass was needed than what was found, this led to the first notion of dark matter.
- In the 1970s, Vera Rubin measured the rotational motions of individual galaxies, finding that the outskirts rotated just as quickly as the inner regions (below). When she looked at the amount of matter present — including stars, dust, and gas — they did not describe the gravity necessary to describe the motions. This also gave support to the notion of dark matter.
Or, did it? In the early 1980s, Moti Milgrom wrote a very interesting paper, where he noted that the galaxy rotation problem could be easily solved without dark matter if you simply made one little tweak to Newton’s law of gravitation. If, instead of using the normal Newtonian force law, you used a modified version that included a minimum value for acceleration, you could accurately describe the internal motions of galaxies. Maybe the solution wasn’t some new form of matter, hitherto undetected, but in changing the law of gravitation. All that scientists needed to do, some conjectured, was to make these modifications — known as MOdified Newtonian Dynamics (MOND) — consistent with Einstein’s relativity on Solar System scales. Do that, and the hope was that the rest of the problems would solve themselves.
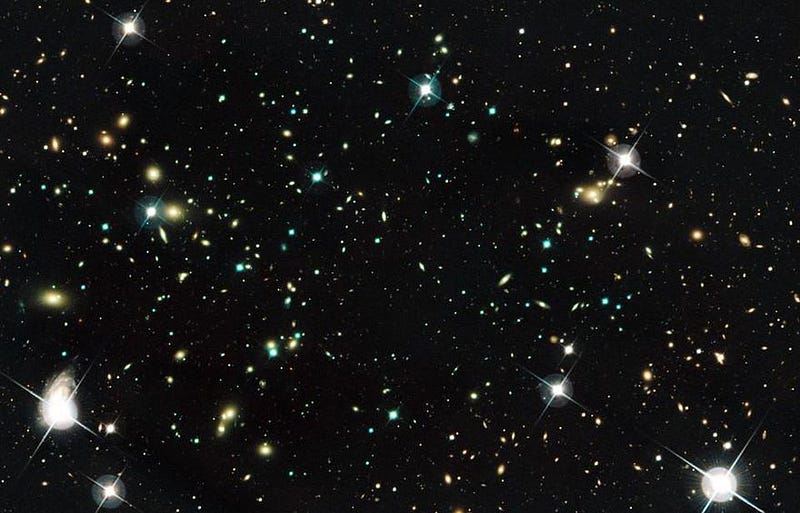
But there were two big, big problems with that idea.
The first problem is that the modifications you’d make to the law of gravity to satisfy individual galaxies would not satisfy the observations of galaxy clusters. The original observations that led to the hypothesizing of dark matter, put forward by Zwicky over 80 years ago, remains unexplained by MOND or any of its alternatives. The “modified” part of MOND cannot be scaled to explain the gravitational measurements we make on larger scales; they really only work on the scales of a single galaxy.
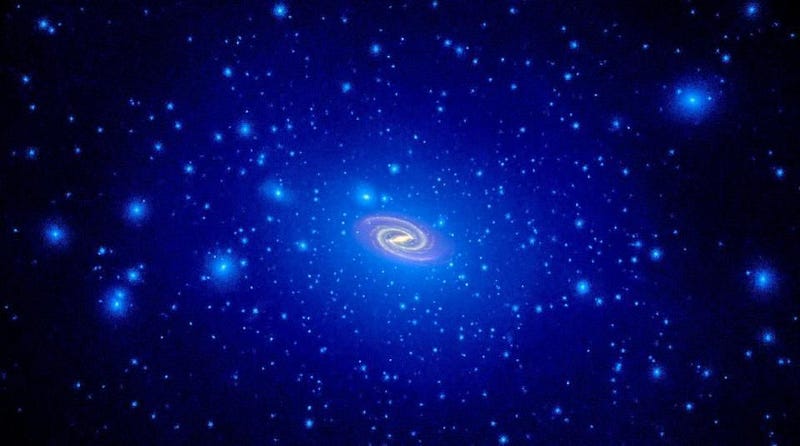
And the second problem is that the environments of individual galaxies themselves are an incredibly unclean, contaminated test of dark matter. Even if it’s a great laboratory for testing MOND, the fact that there is:
- such a large density of normal matter compared to dark matter in the inner regions,
- interplay between radiation and both normal-and-dark matter,
- messy, nonlinear dynamics and feedback mechanisms at play,
- and many forces other than gravitational ones that are important on these scales,
means that even though the galactic predictions of MOND are clear, the predictions of dark matter are murky on the scales of individual galaxies.
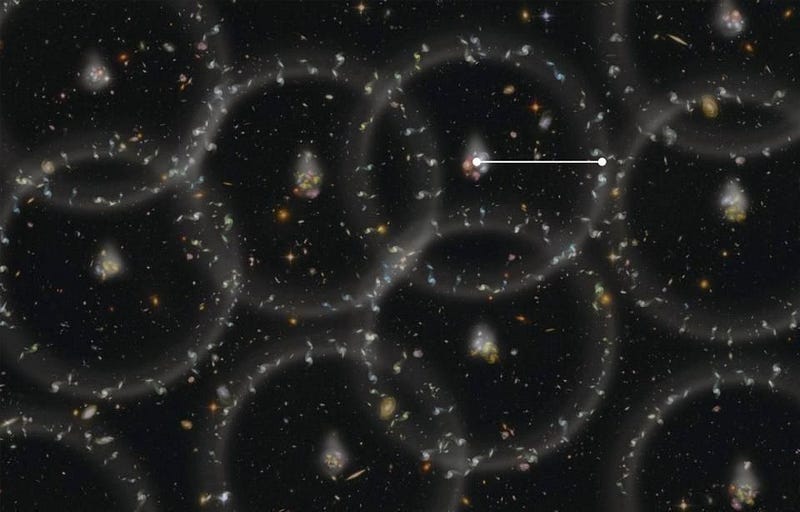
If you add a new ingredient to the Universe, like dark matter, the way you make predictions about it is to simulate the Universe on large scales. When you add a new ingredient, many cosmic observables change in easily quantifiable ways that lead to clean predictions and clean signals. It’s like dropping a sheet of paper or a feather at the surface of the Moon, rather than on Earth; you’ll measure what you intend to measure, rather than the contaminating, messy effects that might get in the way. The best laboratory for that? Examining the large-scale structures present within the Universe.
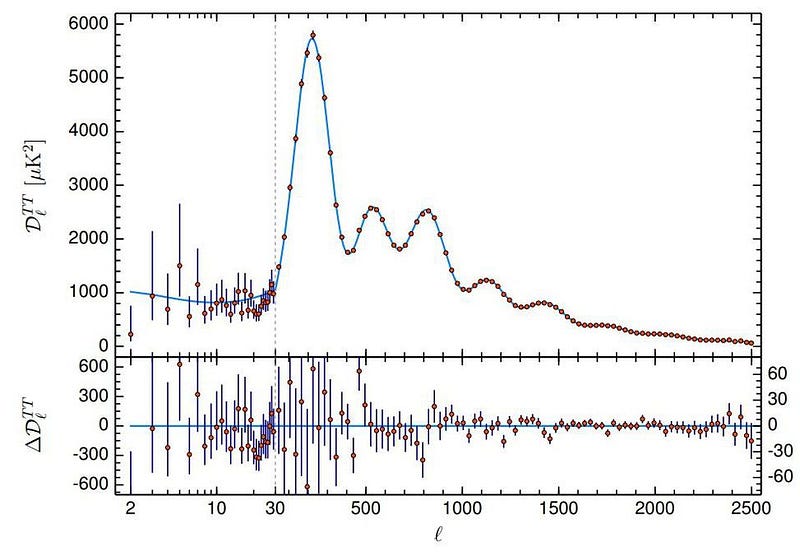
This includes:
- the leftover glow from the Big Bang: the Cosmic Microwave Background, and the minuscule fluctuations present within it,
- the motions of individual galaxies within clusters, like the motions measured by Fritz Zwicky,
- the correlations between where galaxies are located on scales ranging from a few hundred million to many billions of light years,
- the locations of normal matter and a gravitational signal in the aftermath of a massive cosmic collision,
- and the shape, growth, and structure of the cosmic web, including voids, filaments, and their nexuses.
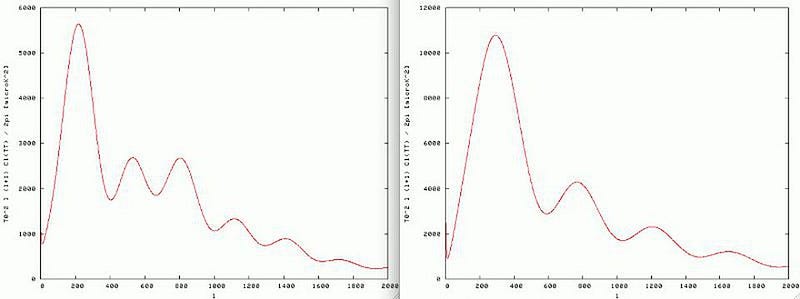
What’s most impressive is that the predictions of dark matter were first made in the 1970s and 1980s, and were observationally confirmed later. This is not a case of tweaking the model to fit the data; this is a case of the best kind of science you hope for: where you make predictions, make the observations, and what you see validates and confirms the predictions you had made.
And yet, even 35 years later, there are no modifications of gravity that achieve the galaxy-scale successes of MOND that also explain these other observations. The best tests of dark matter vs. MOND, which are on large, cosmic scales, have a clear winner and a clear loser.
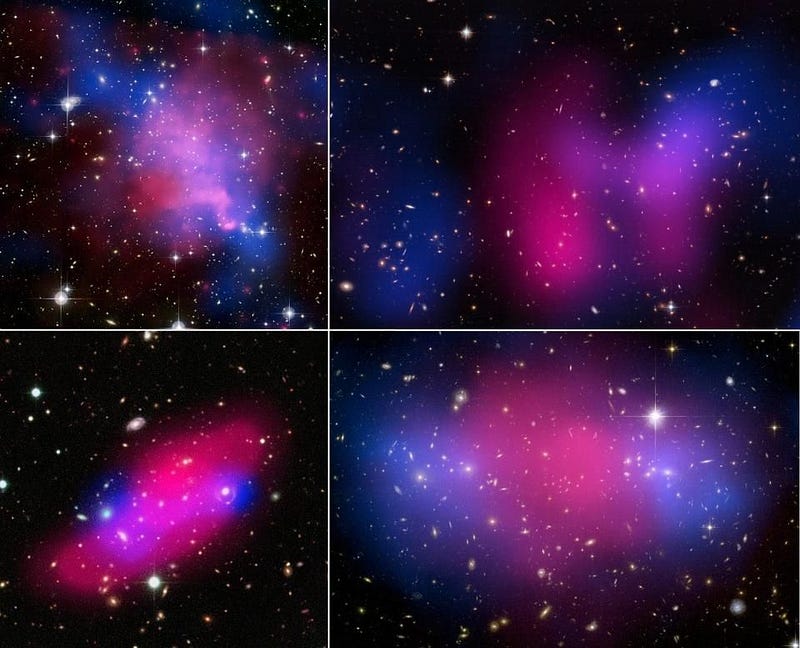
The so-called dark matter vs. modified gravity war, as highlighted in August’s Scientific American story by Sabine Hossenfelder and Stacey McGaugh, sets up a false narrative of a debate between these two camps. Sure, on the scales of an individual galaxy, MOND describes the internal motions and the motions of very small satellite galaxies very well, and dark matter struggles to do so. This may be because something is flawed about dark matter, because there’s no such thing as dark matter, or it may be because we do not fully understand these messy environments to the precision necessary to even make good predictions about dark matter.
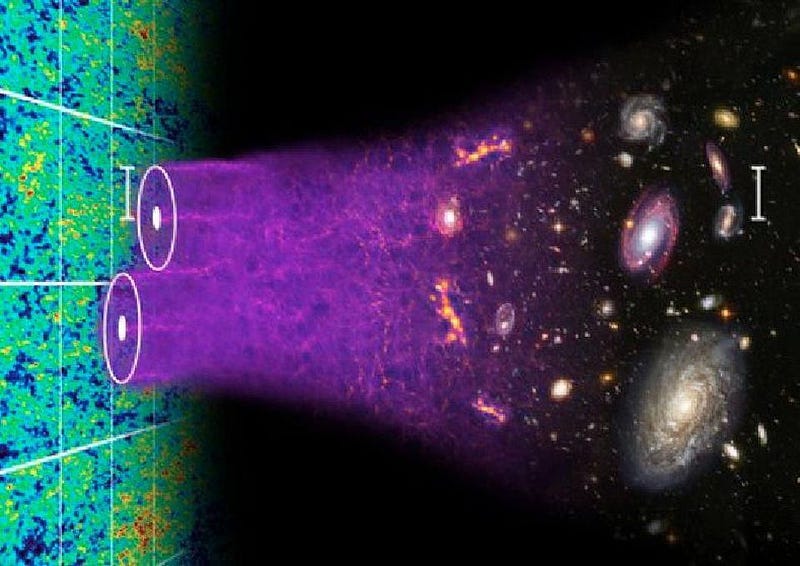
But these are not the decisive tests for dark matter. The cosmological ones are.
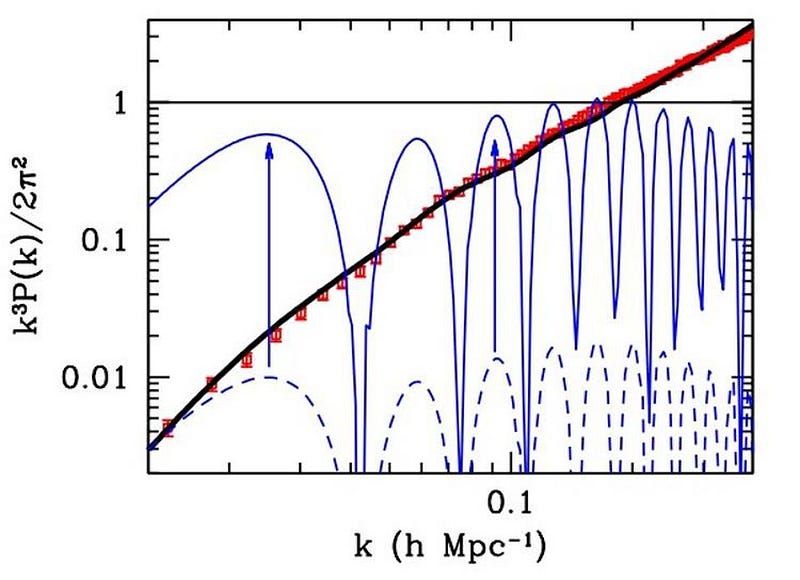
The tests on the largest scales give us the best tests for dark matter. And these are the ones that dark matter not only universally passes, but that MOND has failed spectacularly for, on every account, for the past 35 years. Among cosmologists*, there is no debate, because there is no alternative to dark matter that reproduces the observed successes.
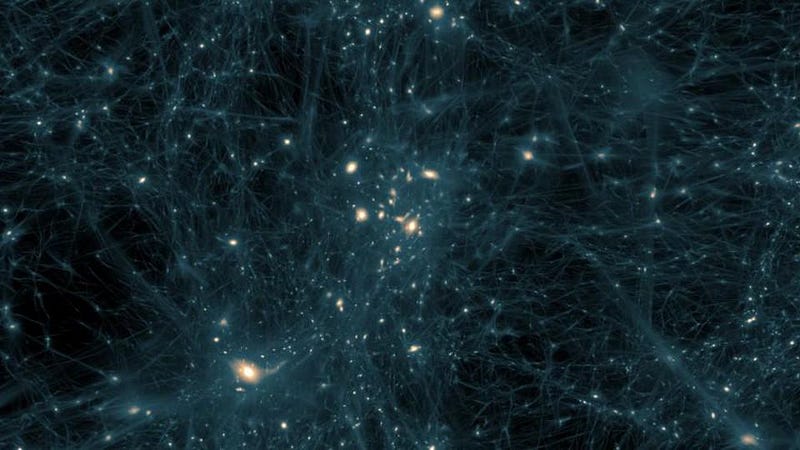
On the scales of groups of galaxies, individual galaxy clusters, colliding galaxy clusters, the cosmic web, and the leftover radiation from the Big Bang, MOND’s predictions fail to match reality, whereas dark matter succeeds spectacularly. It’s possible, and perhaps even likely, that someday we will understand enough about dark matter to understand why and how the MOND phenomenon on the scales of individual galaxies arises. But when you look at the full suite of evidence, dark matter is practically a scientific certainty. It’s only if you ignore all of modern cosmology that the modified gravity alternative looks viable. Selectively ignoring the robust evidence that contradicts you may win you a debate in the eyes of the general public. But in the scientific realm, the evidence has already decided the matter, and 5/6ths of it is dark.
* — Full disclosure: the author of this piece has a Ph.D. in theoretical cosmology.
Ethan Siegel is the author of Beyond the Galaxy and Treknology. You can pre-order his third book, currently in development: the Encyclopaedia Cosmologica.