Ask Ethan: Will our Universe end the same way it began?
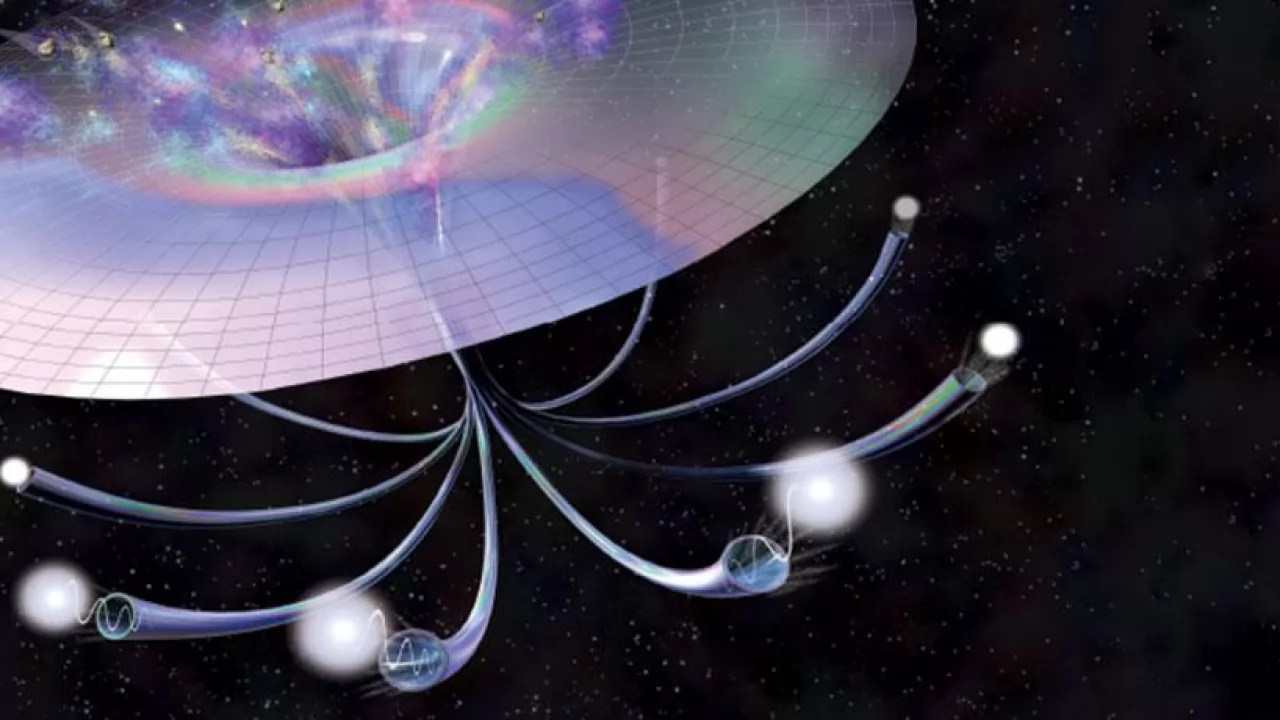
- Our Universe as we know it may have begun with the hot Big Bang, but a very different state, known as cosmic inflation, set up and preceded the Big Bang itself.
- During the end of inflation, the field energy inherent to space itself decayed, transferring itself into energy in the form of particles through a process known as reheating, triggering the onset of the hot Big Bang.
- Today, we still have field energy inherent to space in the form of dark energy, and many have speculated that it could be because we're in a false vacuum state. If that decays, will the Universe end the way it began?
From Peter Pan to Battlestar Galactica, one of the most famous notions in all of fiction is the idea of cyclic repetition: all of this has happened before, and it will all happen again. But does that apply to the cosmos itself? The Universe as we know it began with the hot Big Bang, which itself was set up and caused by a prior state known as cosmic inflation, where the Universe was expanding rapidly and relentlessly for an unknown period of time. When inflation came to an end, the energy throughout space — which had previously been in the form of field energy, or energy inherent to space itself — got converted into the various quanta, kicking off the hot Big Bang.
Today, however, billions of years later, we still have a form of energy inherent to space itself: dark energy. Could this someday trigger a similar scenario, leading to a new sort of Big Bang? That’s the question of Sara Wright, who inquires:
“If I understand this correctly, the big bang occurred when the inflationary field energy was suddenly converted into all the particles and radiation that are present today. But there is also the false vacuum decay scenario, where the zero point energy of space may reach an even lower state than it is now. Are these two events related to each other in any way? If so, is it possible that the same big bang that gave rise to us is still propagating far beyond the cosmic horizon of our observable universe?”
This is a really big question and a fascinating possibility that, to be honest, no one knows whether it describes our Universe’s future or not. Here’s the big idea, and why it’s worth pondering.
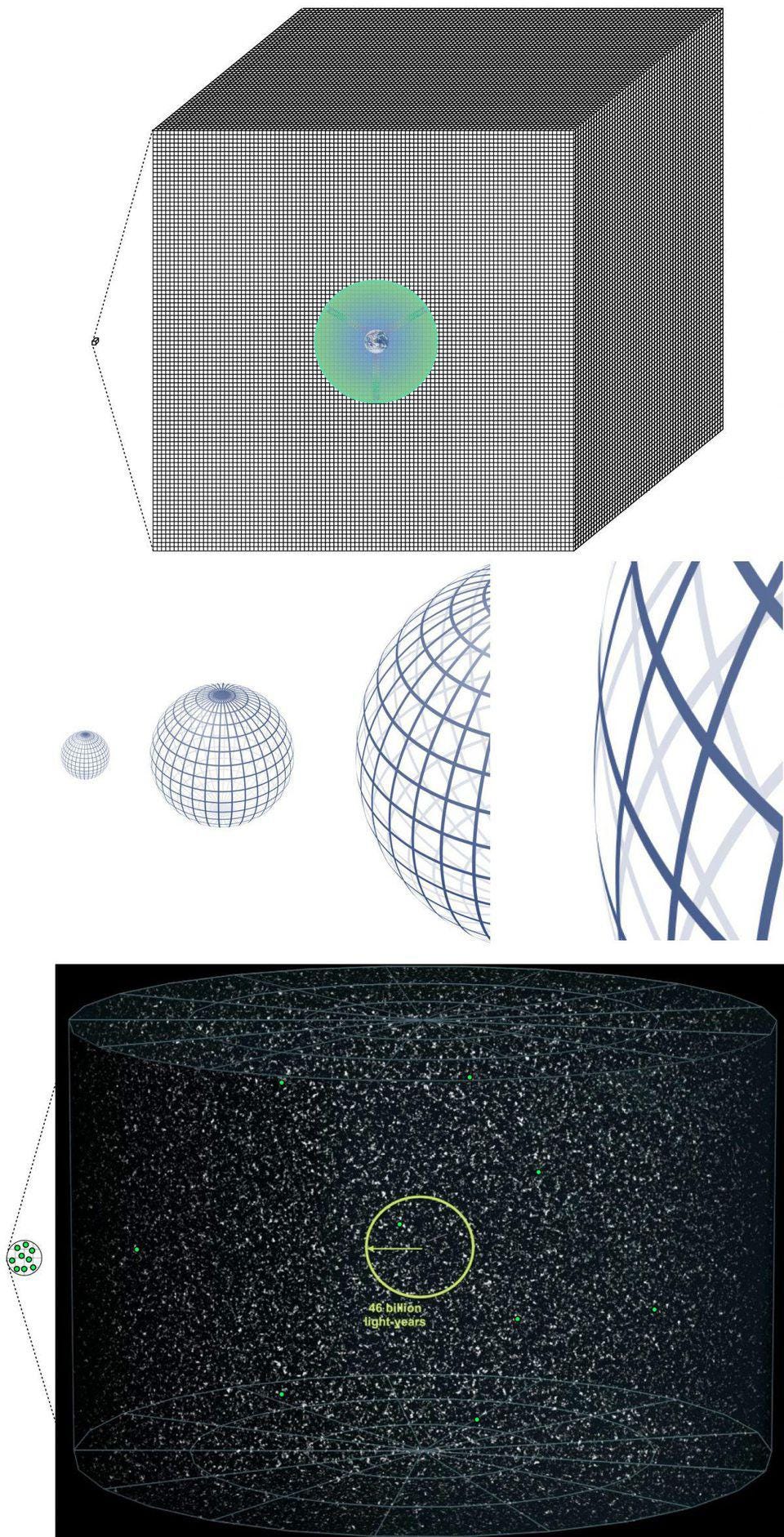
Cosmic inflation, for the uninitiated, came about as a way to explain puzzles about the properties the Universe must have possessed at the start of the hot Big Bang, but that the Big Bang itself provided no explanation for. The original idea of inflation was that the hot Big Bang was preceded by a stage where:
- the energy of the Universe wasn’t in the form of matter and radiation, but rather in a type of energy inherent to space itself,
- which caused the Universe not to cool and slow down as it expanded, but rather to expand at an exponential, relentless rate,
- causing it to double in size with each tiny fraction-of-a-second that passed,
- and then double again in that next tiny fraction-of-a-second,
- and so on,
- until all that remained was flat, empty space with the same properties, such as energy density, everywhere,
- except for the quantum fluctuations superimposed atop this uniform background.
This explains the conditions that are known to have existed at the start of the hot Big Bang, including a nearly-perfectly uniform temperature and density in all directions and locations, a nearly-perfectly spatially flat observable Universe, and a Universe free from leftover high-energy relics such as magnetic monopoles. It also provided predictions that contrast with predictions from the Big Bang without inflation, which have since been validated by observations, including a spectrum of initial fluctuations that are slightly, by only a few percent, greater on large cosmic scales than small ones, where all of those fluctuations are adiabatic and not isocurvature, where fluctuations exist on scales larger than the cosmic horizon, and where those fluctuations are Gaussian in nature, obeying a Bell curve to their distribution.
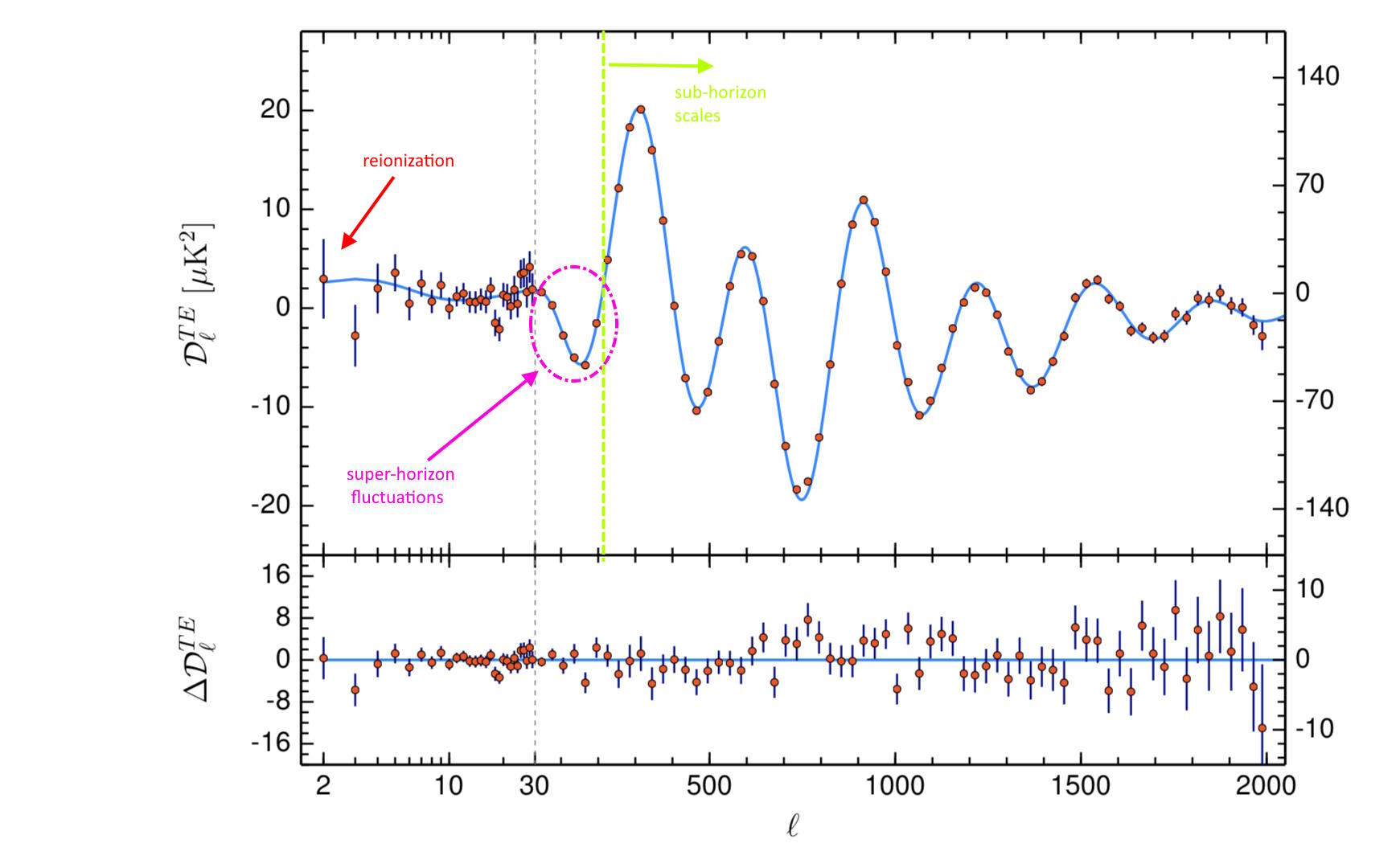
But the relevant part of inflation for the puzzle we’re considering — if the beginning of our Universe as we know it, defined by the transition from inflation to the hot Big Bang, is related to a potential fate of our Universe through vacuum decay — demands that we look into the working of how inflation comes to an end. In physics, we refer to this as a phase transition: where something was one way before, in a seemingly stable or quasi-stable fashion, and then in short order was converted into being a different way, where that new way is now stable or quasi-stable, and it won’t go back to being the way it was before.
The way we view a phase transition, in general, is to imagine you have a ball that lives on a hill, and that hill — a stand-in for what physicists know as a “potential” — can be any general shape that describes your system’s behavior. The simplest type of hill (or potential) we can draw is going to be a one-dimensional line, which is continuous (i.e., without any gaps) and differentiable (i.e., smooth), but can take on any form or shape that fits those criteria. Initially, when it was first proposed, people who were thinking about inflation considered a potential that had multiple local minima to it, but only one true minimum, and they imagined inflation as a state that got caught in one of those “false” minima.
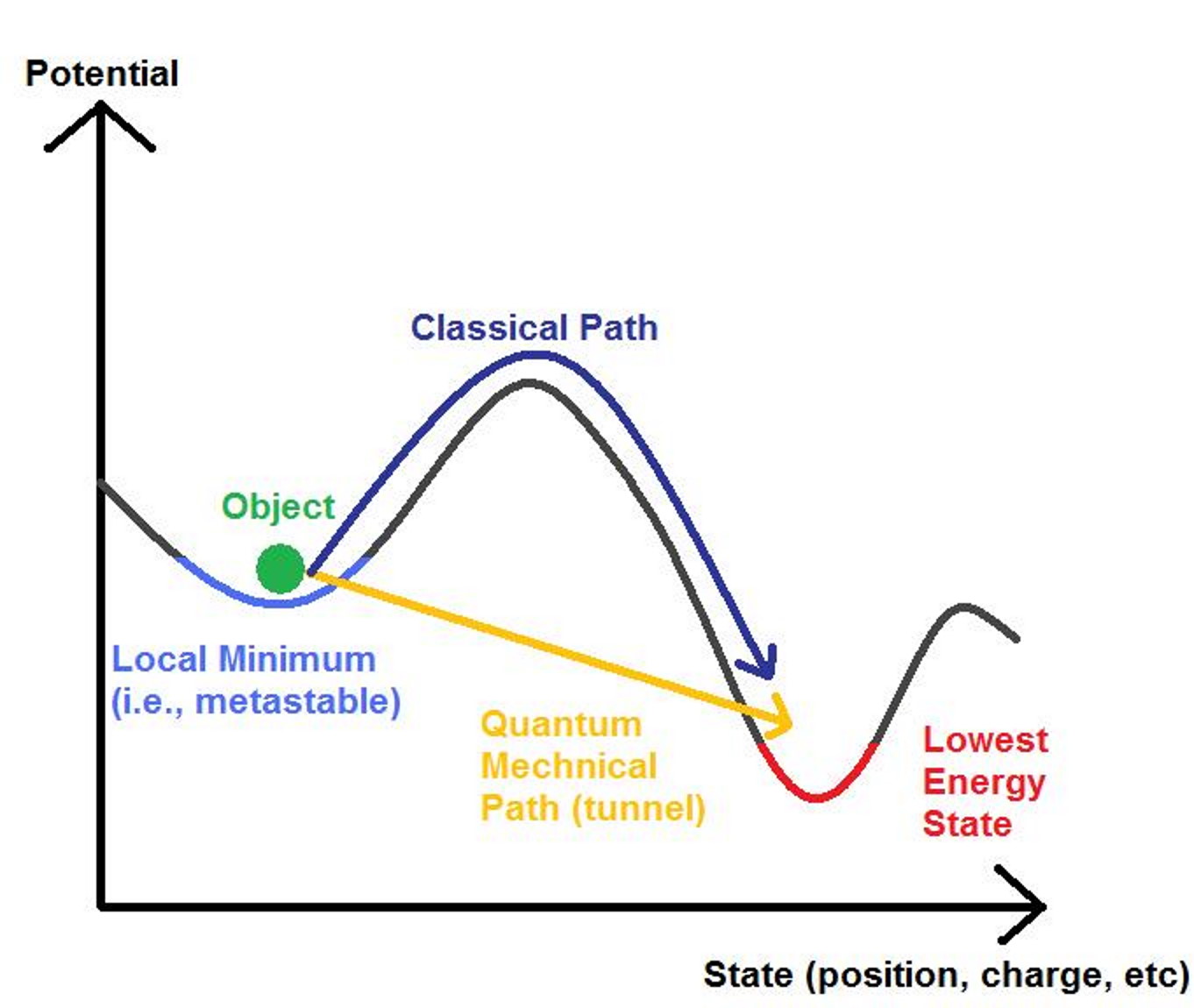
This was the first proposal for inflation, and today is known as “old inflation” among cosmologists. First proposed by Alan Guth, generally regarded as inflation’s founder, it was swiftly realized that the idea of old inflation faced a major problem: it couldn’t reproduce the hot Big Bang as required. During inflation, you can consider a region of inflating space as a three-dimensional volume, where the interior of that volume is the region that is inflating. The end of inflation can be thought of as applying to one specific “bubble” within that volume, where that bubble, in the example above:
- has an interior (the bubble’s insides),
- has a boundary (the bubble’s walls),
- and where the end of inflation occurs through quantum tunneling.
Remember that the defining characteristic of the hot Big Bang is that space needs to be filled with matter-and-radiation uniformly (to within the limits of quantum fluctuations): the same at every location and the same in all directions. But for that to happen, the energy that’s bound up inside that bubble-like region has to wind up in the interior of the bubble, not in the bubble walls.
That’s the big problem for this quantum tunneling model of inflation — of “old inflation” — that makes it so suspect: this type of phase transition would put the energy into the bubble walls, rather than into the interior. Because of this problem, this model was eventually abandoned.
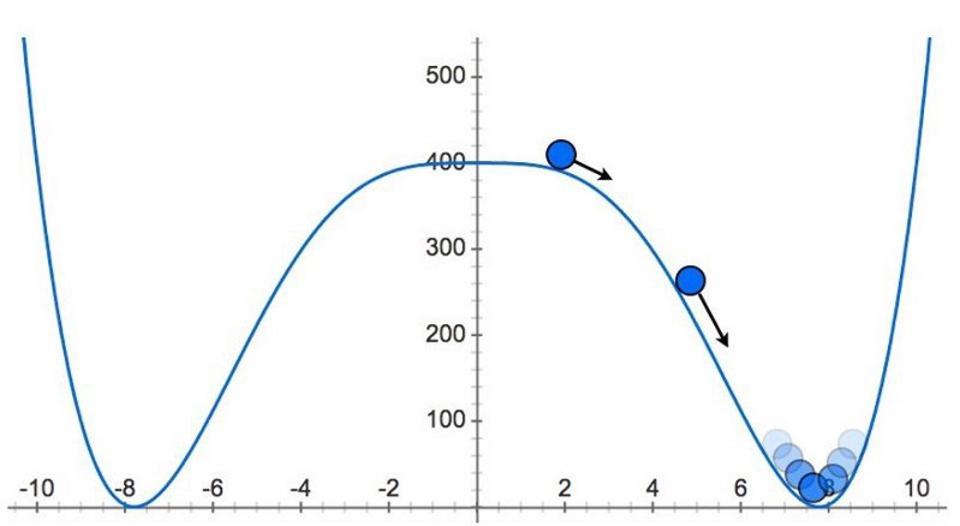
And what was it replaced by? By a model of inflation with a different type of potential and a different type of transition: cleverly named “new inflation” instead. Everyone should know that, in physics, there are two main classes of phase transition in physics, very cleverly named “first-order” and “second-order” phase transitions.
- A first-order phase transition is like the quantum tunneling variety, where you have a false minimum that abruptly transitions to the true minimum. In this instance, the “ball” spontaneously switches from being in that false minimum state to the true minimum state, and the change from one state to another is instantaneous.
- A second-order phase transition, however, is like the above diagram, where a ball begins atop a plateau and gradually rolls into the valley below, where it oscillates down at the bottom around the minimum it encounters.
In the case of inflation, a first-order transition would lead to all of the energy being within the bubble walls of any one region where inflation comes to an end. However, a second-order transition leads to all of the energy being converted into various quanta (e.g., particles and/or antiparticles of the Standard Model) in the interior of that bubble region. This is important, because within the context of inflation, the regions where inflation ends — which is to say, different “bubbles” within inflating space — never overlap or contact one another.
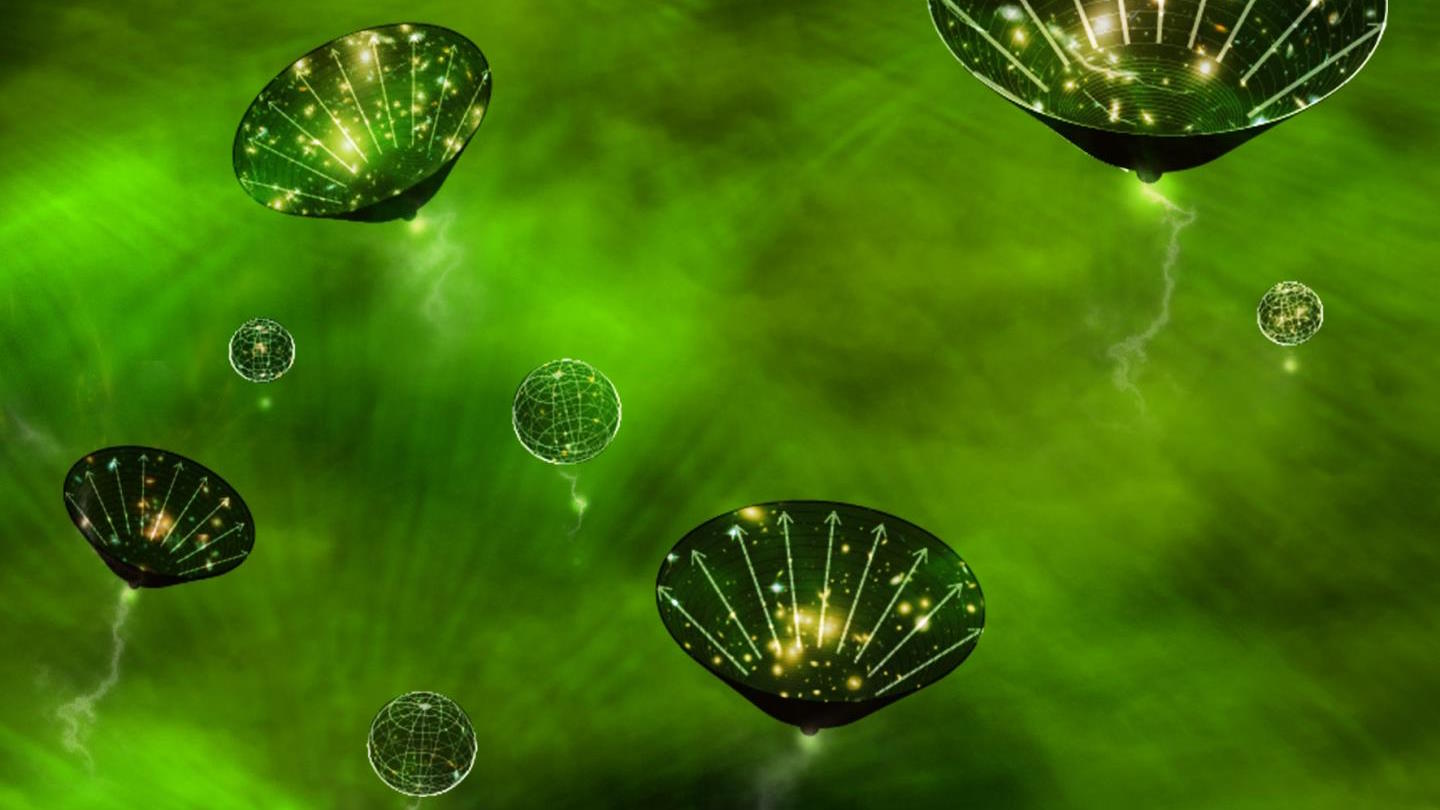
That’s because during inflation, the expansion rate never drops. But as soon as inflation ends in any location, the expansion rate suddenly plummets. The reason is simple: it’s because it’s the energy density of the Universe that determines the expansion rate. When your Universe is filled with field energy, or energy inherent to empty space, the energy density remains constant. However, once your Universe becomes filled with quanta — things like matter, antimatter, and radiation — the energy density drops, since the number of quanta remains fixed while the volume of space expands.
Therefore, in an inflating Universe, the expansion rate remains at a high value indefinitely, for as long as inflation takes place. Wherever there’s an inflating region, it keeps inflating relentlessly, driving all regions apart from one another in an exponential fashion.
But wherever inflation ends, the expansion rate immediately begins plummeting, so any “bubbles” where inflation ends always expand more slowly than the inflating regions surrounding them. This implies that:
- no two bubbles where inflation ends will ever touch,
- no two bubbles will ever overlap or collide,
- and so you cannot have multiple bubbles smashing into one another and energy exchanging between the various bubble walls,
- and therefore inflation cannot end in a first-order phase transition (i.e., through quantum tunneling),
- but must instead end in a second-order phase transition: through rolling down a hill and into a valley.
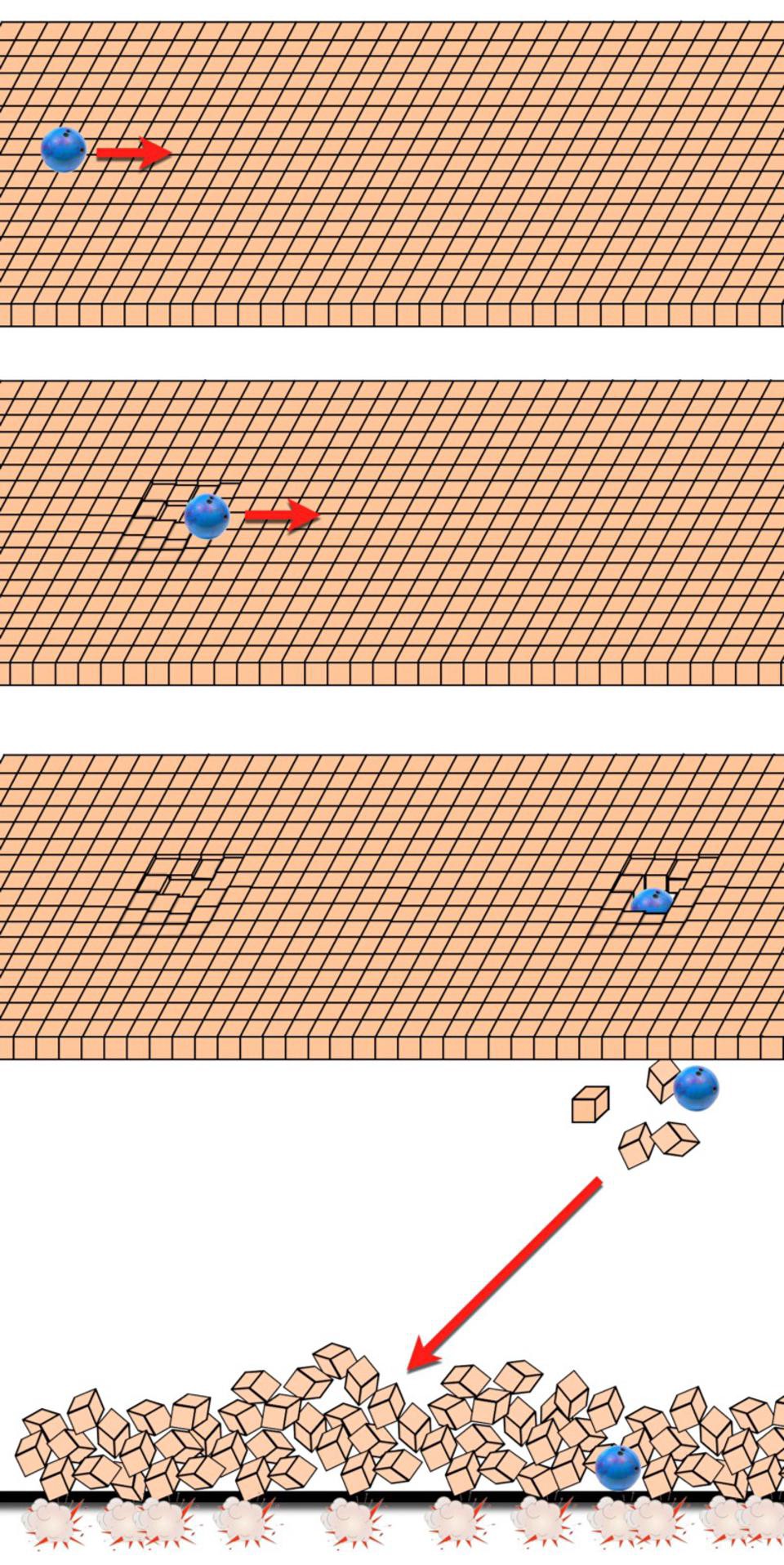
Inside each individual bubble where inflation comes to an end, the “ball” rolling down the hill into the valley will oscillate at the bottom, which converts field energy (or energy inherent to space) into particles: a process known as cosmic reheating. The fact that there’s an upper limit to the temperature achieved during the hot Big Bang — a temperature much lower than the Planck temperature — is a further piece of evidence in favor of inflation and against extrapolating the Big Bang back to arbitrary temperatures, densities, and energies.
So that’s the idea of inflation: we once had all of the Universe’s energy locked up in the form of a field, or alternatively, in the form of energy inherent to space itself. This species of energy caused the Universe to expand relentlessly and in an exponential fashion, doubling in size with each tiny fraction-of-a-second that elapses. Inflation continues so long as this “ball” remains atop a relatively flat plateau, and ends when the ball rolls down into the valley: an example of a second-order phase transition. And that transition causes inflation to end, resulting in the hot Big Bang as part of the aftermath.
It is for this reason that we no longer think of the Big Bang as a singular beginning to time and space, but merely the start of the Universe as we know it. There was a phase prior to it, cosmic inflation, that preceded it and set it all up.
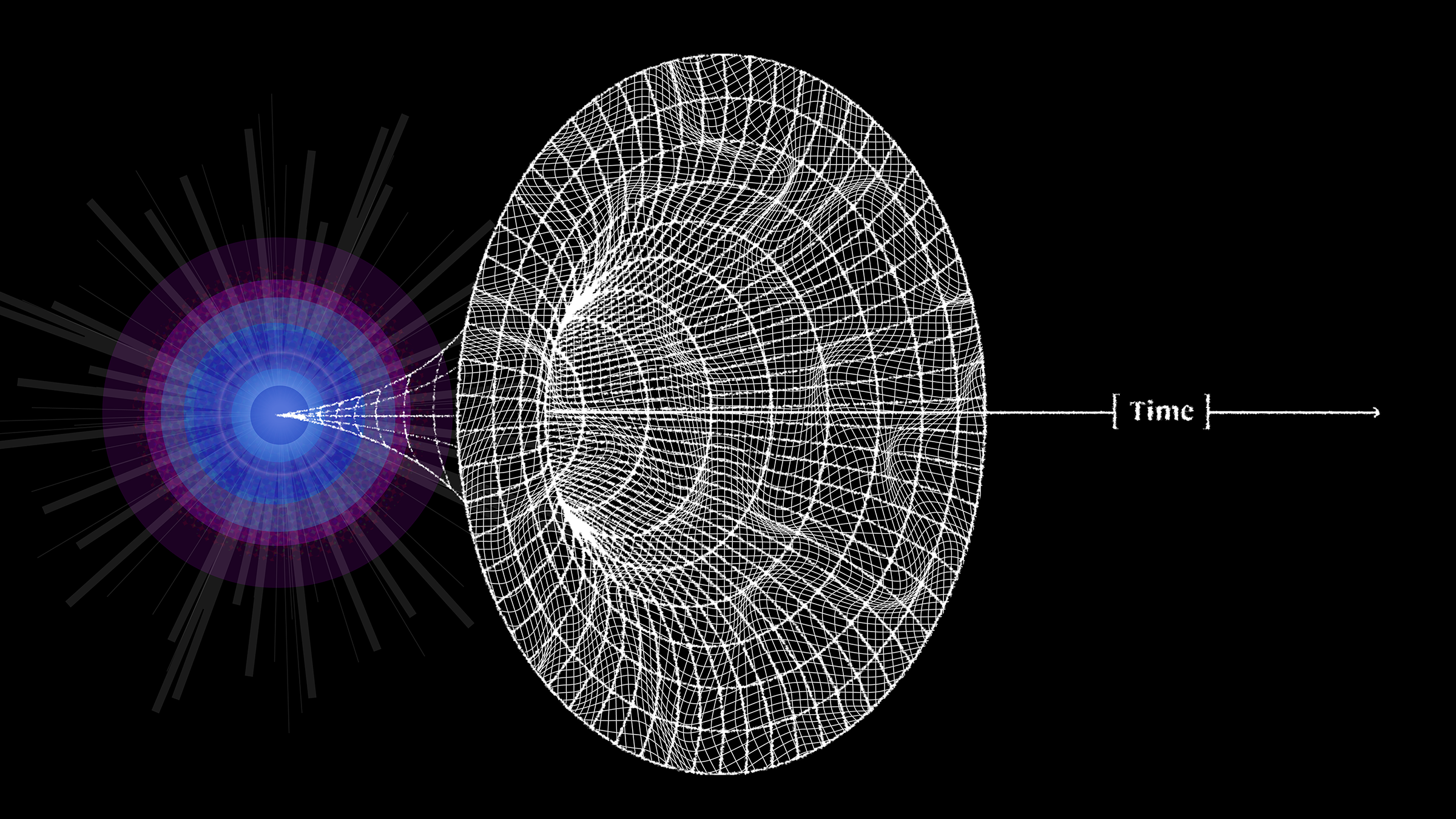
And now, we get to fast-forward to the present day: where the end of inflation and the start of the hot Big Bang is billions of years in the cosmic past. Today, our Universe is not even dominated by matter or radiation any longer, but by a mysterious form of energy that causes the Universe’s expansion to speed up with time. We call this form of energy dark energy, and have inferred its properties by measuring the expansion of the Universe throughout its cosmic history. Perhaps surprisingly, its properties look a little bit familiar.
- It appears to be a form of energy that was undetectable early on in cosmic history, when the matter and/or radiation densities were large.
- About 8 billion years ago, its effects start to appear in the data, causing the Universe to expand more quickly than it would have without dark energy.
- About 6 billion years ago, it became the dominant effect in the expanding Universe, marking the transition between where distant objects would slow down (as they did previously) in their recession from us versus where they would speed up (as they do subsequently) and recede faster and faster.
- And about 4.5 billion years ago, right around when planet Earth was forming, it became the dominant form of energy in the Universe: outstripping dark matter and normal matter and radiation and neutrinos combined.
Remarkably, it behaves as though the dark energy density remains constant over time: just as the energy density behaved all the way back during inflation.

Where does dark energy come from, then, and what is its true nature?
In each of our theories that describe the fundamental forces and interactions in the Universe, a possible origin for dark energy arises. One is in the context of general relativity, our theory of gravitation. In the context of general relativity, there is a form of energy that behaves just like the observed dark energy does: Einstein’s cosmological constant. A form of energy inherent to space itself, it represents what remains if you remove all the matter, antimatter, radiation, and any other quanta from the Universe. Empty space still has energy, and the cosmological constant, which we measure to be positive, quantifies it. It may be over 100 orders of magnitude (a factor of 10100) smaller than the energy density present during inflation, but it still exists.
The other possible source is from the quantum vacuum: the energy of empty space owing to the zero-point energy of the quantum field theories that describe our Universe. The lowest-energy state of a quantum system isn’t necessarily zero, but can often take on a positive, non-zero value, just as it does for the hydrogen atom. And it’s in this context that people have proposed vacuum decay scenarios, theorizing that perhaps the Universe isn’t in its true minimum state, but that — as evidenced by dark energy’s positive, non-zero energy density — it may be in a false minimum, with the potential to transition to an even lower state in the future.

But now, we have to remember the two types of phase transitions that we talked about: the first-order ones, which correspond to quantum tunneling from a false minimum into a true minimum, and the second-order ones, which correspond to slowly rolling from a flat-topped hill down into a valley below. Cosmic inflation, in order to reproduce the Universe we observe ourselves to have, had to have been a second-order phase transition. But vacuum decay scenarios all rely on quantum tunneling, strongly suggesting that a first-order phase transition would be the culprit.
In other words, although dark energy and inflation may be related and analogous in many ways, the scenarios of “the end of inflation and the start of the hot Big Bang” and “the vacuum decay of dark energy into a lower-energy state” have important fundamental differences to them! It still may be the case that there is some sort of relationship between the long-ago inflationary state and today’s dark energy, and there is still the possibility that, for both inflation and any future vacuum decay scenario, the shape of the potential that leads to such a transition can change over time. However, any discussion of a vacuum decay scenario in our future remains purely speculative, as there is no evidence indicating that such a decay ever has occurred or ever will.
When it comes to the great cosmic unknowns, we have to keep our minds open to all possibilities that have not yet been ruled out. However, without any evidence to validate a fanciful scenario like vacuum decay, we’re forced to admit that there’s no evidence that such a thing will ever occur, and that if it does, we’d have to learn something profoundly unexpected about it to link it back to inflation. The idea can’t be ruled out, but it’s firmly in the realm of pure speculation at this point.
Send in your Ask Ethan questions to startswithabang at gmail dot com!