Warp drive’s best hope dies, as antimatter falls down
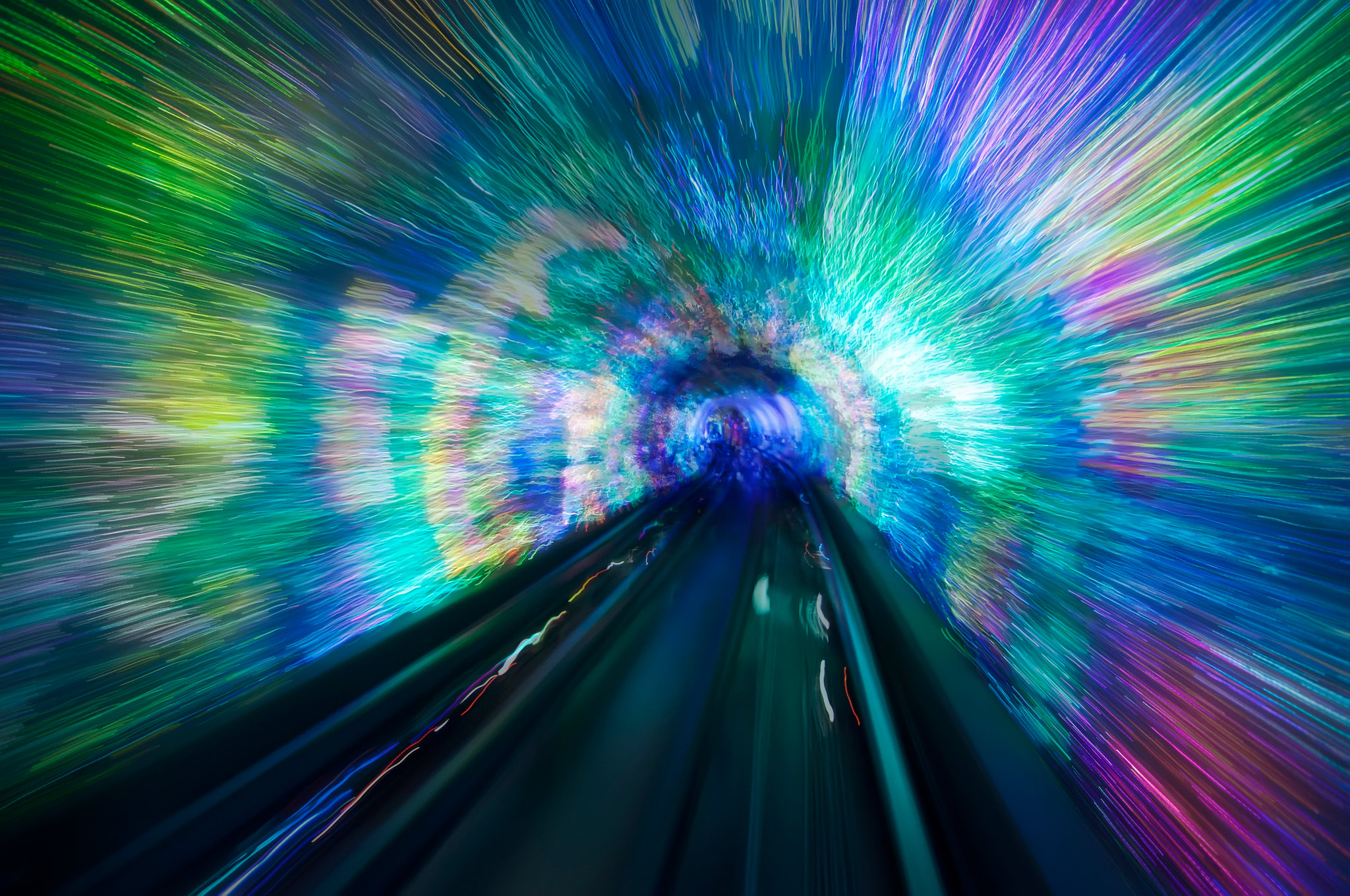
- In order to make a working “warp drive,” there must be some way to not only curve the fabric of space, but to curve it in fundamentally different directions ahead of and behind the spacecraft.
- By creating a positively curved region “contracting” the space in front of a spacecraft and a negatively curved region “rarefying” it behind, a “warp bubble” could be created.
- However, that would require negative mass/energy, and negatively-gravitating antimatter could have done it. Unfortunately, physics said otherwise: antimatter falls down.
Ever since we first looked up at the night sky and saw the glittering tapestry of distant stars, humanity has wondered about what else might be out there. As we learned more about the Universe, our curiosity only increased. We discovered not just the thousands of stars visible to the naked eye, but hundreds of billions within our own Milky Way, and a collection of an estimated trillions of galaxies within our observable Universe. We now know that most of these stars likely contain a system of planets orbiting them, and many of those planets contain properties — like temperature, size, mass, and their atomic compositions — that are very similar to Earth.
Unfortunately, our hopes of visiting and exploring these worlds face a tremendous obstacle: the limitations of the speed of light. While it’s theoretically possible to accelerate objects, even macroscopic ones, close to the speed of light, the laws of physics not only prevent us from achieving or surpassing that speed, but they damn us to the severe experience of time dilation. Even if we were to get in a spaceship and travel at near-light speeds, everyone back at home would age spectacularly while we undertook our interstellar journey.
There was only one potential way out: warp drive, or taking a “short-cut” by severely curving the fabric of spacetime. Long thought impractical, the physics behind this possibility was worked out by Miguel Alcubierre in 1994, and the only requirement was something that anti-gravitated: something like “negative energy” or “negative mass.” With the recent measurement that antimatter falls down in a gravitational field, humanity’s greatest hope for practically achieving our warp drive dreams just suffered a massive blow. Here’s the science behind the amazing, but sobering, result.
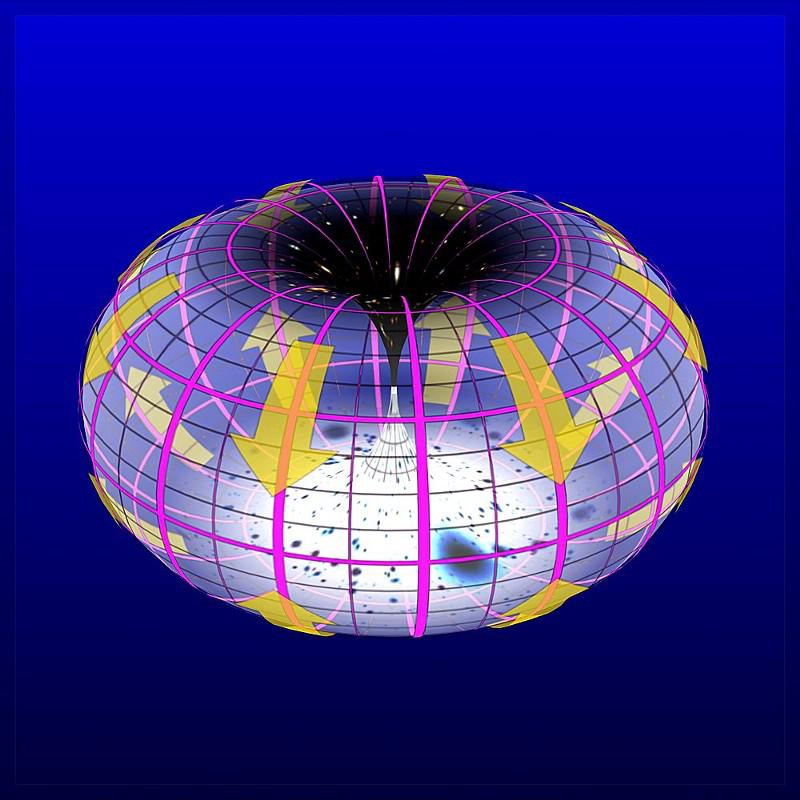
Warp drive’s Einsteinian origins
The seeds of warp drive were planted even before Einstein’s general theory of relativity came along: back in the 19th century, when mathematicians began playing around with new types of geometry that went way beyond the imaginings of Euclid and his successors. For most of the history of mathematics, space could contain up to three dimensions, and each dimension could be correctly represented by a set of Cartesian “grid lines,” where lines that were parallel would always continue forever and never intersect.
This began to change as mathematicians began to consider two novel possibility at around the same time:
- that there could be “extra” spatial dimensions beyond the three we recognize,
- and that space could be curved as well, rather than spatially flat.
These realizations brought with them a number of immediately profound implications. Triangles, if you summed up the three angles inside of them, could add up to fewer or greater values than 180 degrees, dependent on whether your space was negatively or positively curved. Parallel lines wouldn’t remain equidistant forever, but would either diverge or converge dependent on whether your space was positively or negatively curved.
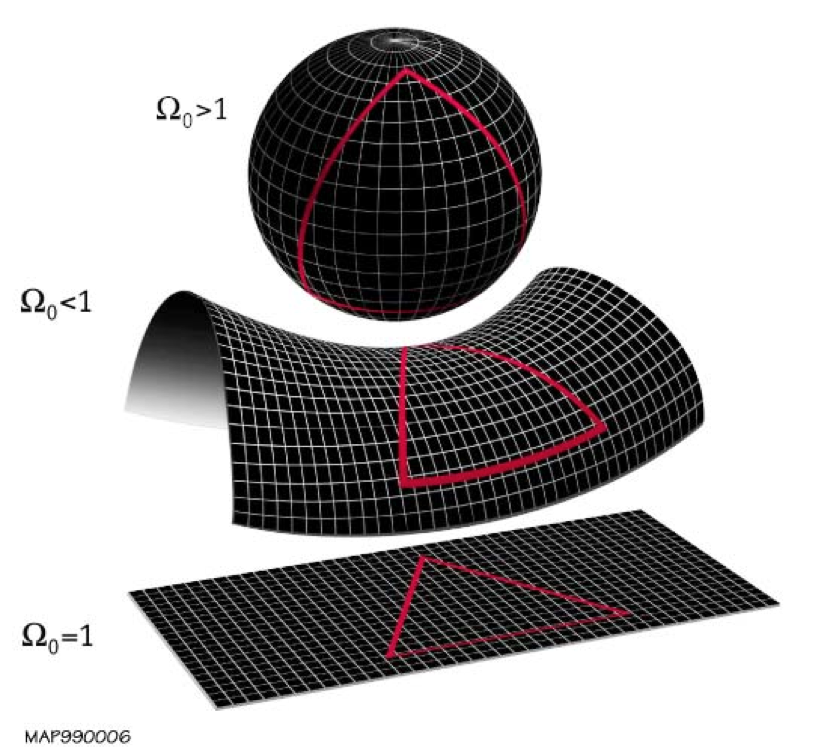
And in an extreme scenario, you could even imagine your spacetime being curved so severely that two points that would conventionally — in a Cartesian/Euclidean-like space — be located far apart are actually “folded up” so that they connect. If this were the case, it would allow almost instantaneous transport between these two seemingly disconnected locations all at once, simply by taking a “short-cut” that was made possible by the severe folding or curving of space.
Many mathematicians played around with these new possibilities, inventing and developing entirely new branches of mathematics, such as non-Euclidean geometry, that worked out the details of a myriad of scenarios under these novel conditions.
When Einstein first came up with his special theory of relativity in 1905, it was swiftly realized that two new consequences were going to come along with his revelation that space and time could no longer be treated as absolute quantities. One was that space and time were inextricably woven together into a single four-dimensional fabric: spacetime. And the other was that each observer within the Universe would experience space and time differently, depending on their relative positions and motions to one another.

Gravitation and general relativity
Einstein was one of many to recognize that his new notion of special relativity and the old formulation of Newtonian gravity were fundamentally incompatible, and that a new theoretical conception of gravity would be required to bring order to the Universe. It was what Einstein would later call his happiest thought — the equivalence principle — that would eventually lead to our new theory of gravity: general relativity.
The equivalence principle asked a simple question: imagine you’re in a room, and all of the objects in your room accelerate downward, toward the floor, at what we conventionally know as g, the acceleration due to gravity at Earth’s surface. From inside this room, what test, Einstein wondered, could possibly be performed that would distinguish:
- whether the room were at rest on the surface of the Earth,
- from whether the room were accelerating due to an outside thrust in the vacuum of space?
The answer, Einstein quickly realized, was nothing. All observations would yield identical results under these two circumstances, and therefore, the two scenarios of acceleration due to gravity and acceleration due to an outside force (i.e., Newton’s F = ma) were equivalent.
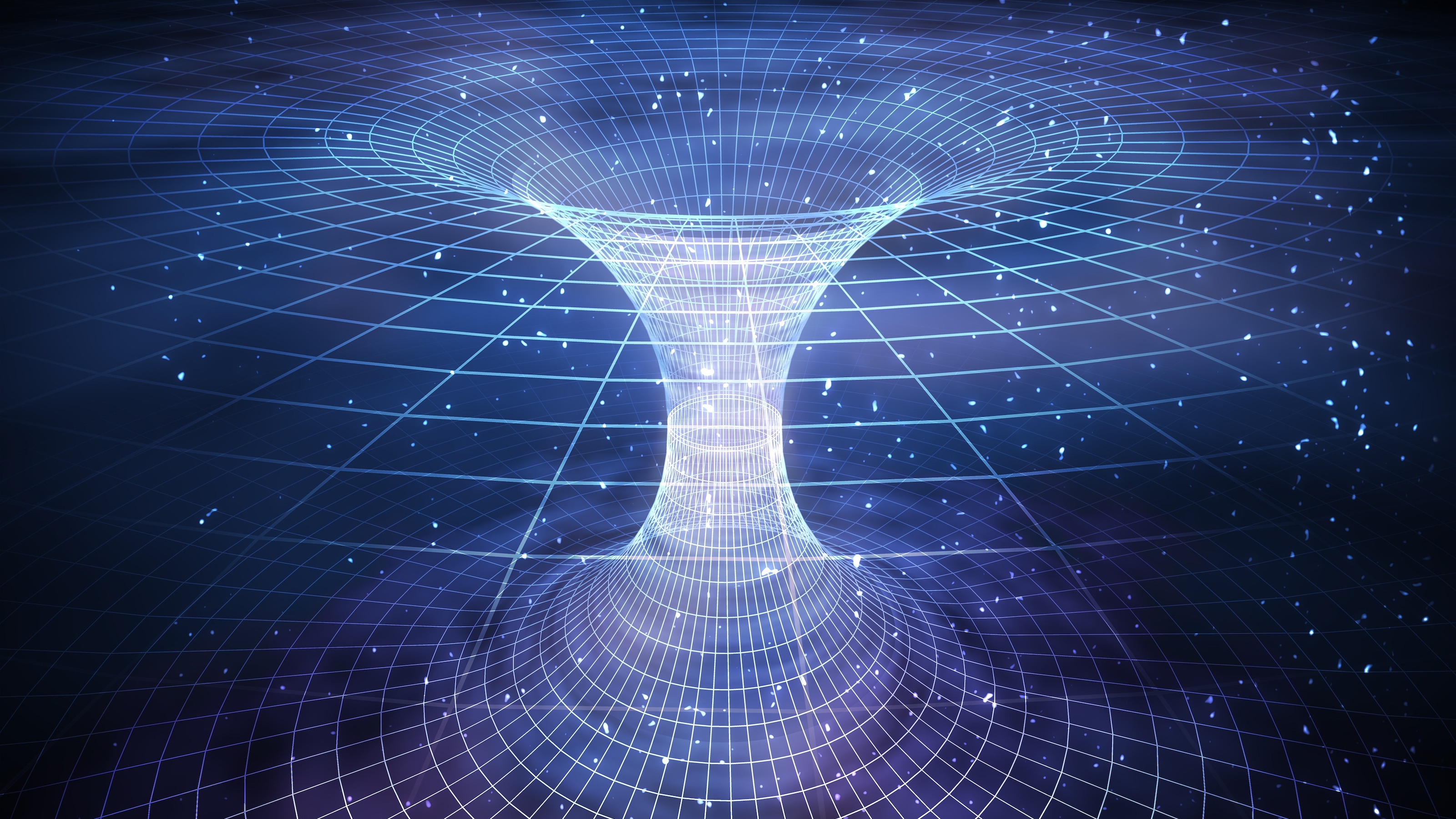
It took Einstein several years, the exploration of numerous mathematical dead-ends, the need of enlisting mathematicians whose skills far surpassed his own, and going back and correcting a number of his own mistakes before finally, in 1915, he arrived at his general theory of relativity (commonly just known as general relativity) in its final form.
This theory still contained a four-dimensional fabric of spacetime, with three spatial dimensions and one time dimension, but this time, it also included gravitation. The brilliant realization was simply that mass-and-energy, in both magnitude and distribution, dictates the curvature of the underlying fabric of spacetime, and then that curved fabric of spacetime told all forms of mass-and-energy how to move through it.
About 20 years later, a remarkable solution within general relativity was discovered.
In 1935, Einstein and his student, Nathan Rosen, published a paper where they demonstrated the feasibility of curving space in such a way that two disparate locations — separated by a great distance through space and time — could be connected by a bridge through severely curved space. Known as an Einstein-Rosen bridge, or “ER” for short, this later became synonymous with the notion of a wormhole, with theoretical consequences that are still being uncovered.
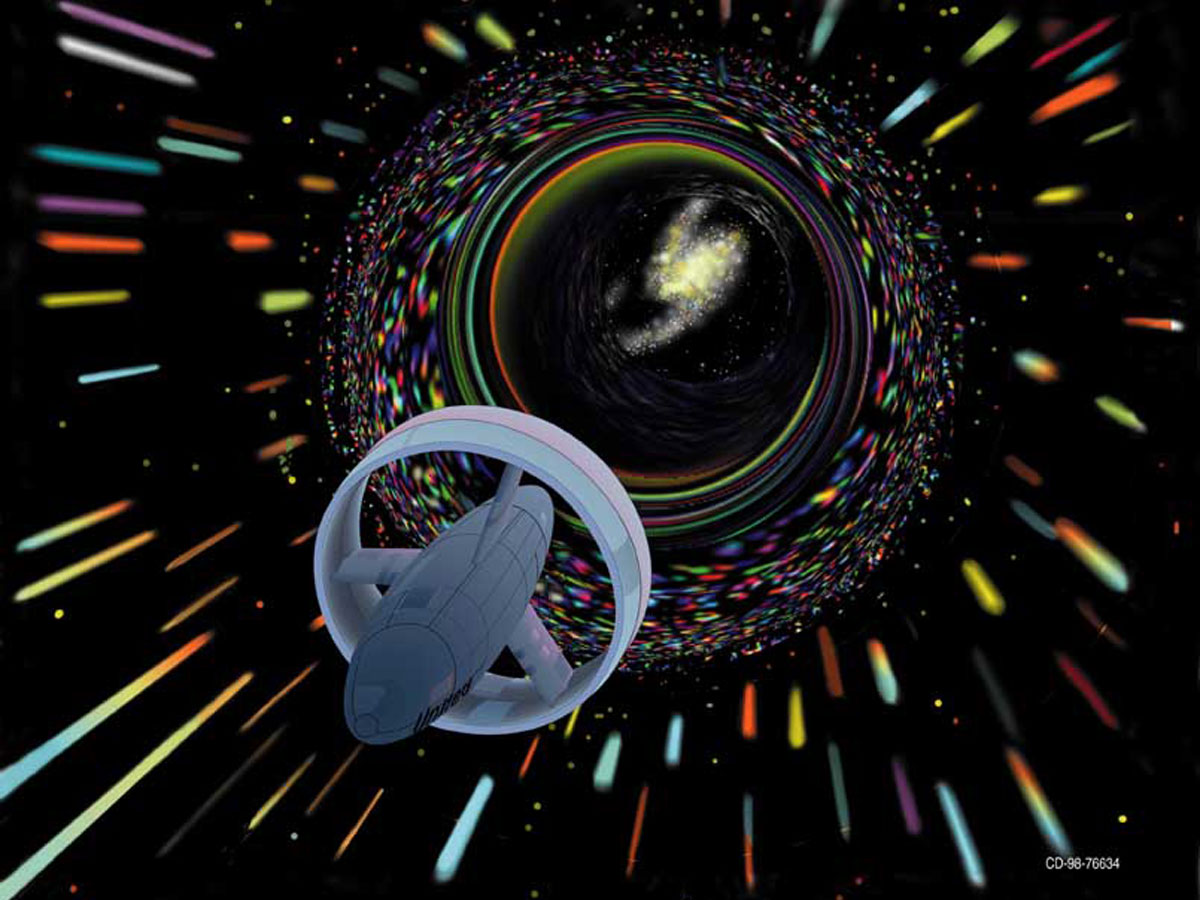
First fiction, then science
These Einstein-Rosen bridges, unfortunately, came along with some very troubling consequences: what physicists often call “pathologies” in the theory. These wormholes would be unstable, in the sense that they could not be maintained as permanently open and traversable entities. They would instantly pinch-off and collapse, for one, and if you attempted to pass any matter through them, the gravitational tidal forces from the severe curvature of space would absolutely destroy it. Nevertheless, the wormhole, or the notion of using the curvature of space to take “short-cuts” through space, found a home in the imagination of many, including among popular fiction and science-fiction writers.
If any sort of mechanism could exist for circumventing the limitations of conventional travel through spacetime — limitations set by the speed of light — it must involve leveraging the curvature of spacetime to create such a “short-cut” between two otherwise disconnected points. Perhaps the most famous instance in all of fiction to leverage this was the idea of “warp drive” as developed by the Star Trek franchise. By expending a vast amount of energy, the idea was that space could be severely curved, and in some sense, compressed. As the space ship moved through the compressed space, it would take this long-sought-after short-cut, enabling very rapid travel over great distances, without causing the outside Universe to age rapidly relative to the crew.
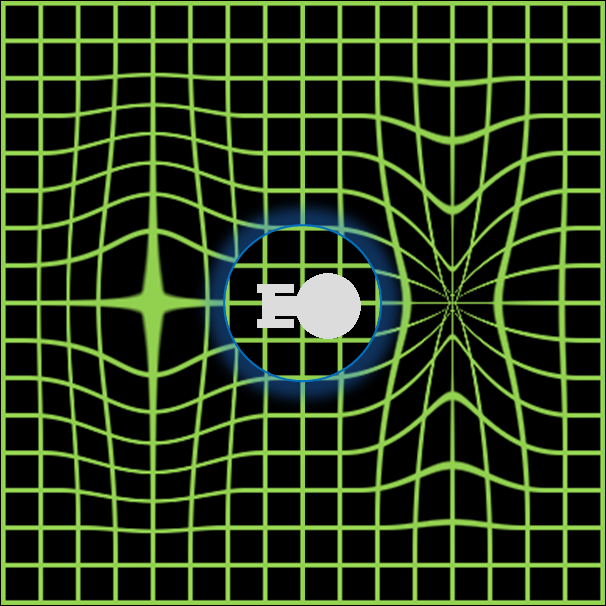
While the idea of warp drive itself, put forth in the 1960s, was extremely hand-wavy and light on the math, a physicist named Miguel Alcubierre — allegedly playing around and stalling while he was supposed to be writing his PhD thesis — worked out some very fun details that could lead to a physically realistic version of warp drive.
Since positive mass and energy curve spacetime in a particular way, Alcubierre realized that if negative mass/energy was allowed to exist, it could curve spacetime in an equal and opposite way. These two types of mass/energy, both positive and negative, could be configured so that:
- the space “in front of” the spacecraft was bent so that it would be compressed,
- while the space “behind” the spacecraft would be oppositely bent so that it would expand in compensation,
- while the space in between them would be flat and bubble-like, enabling the ship within to not be destroyed.
If there were some type of negative energy in the Universe that could be harnessed and manipulated, then this type of warp-drive spacetime, now known as the Alcubierre metric, could be created in real life. The only problem, of course, is that all of the particles and fields known to exist, even in theory, only have one type of mass/energy: the positive type, and hence they all curve spacetime the same way. If warp drive is to be physically realized, some sort of “exotic matter” with negative mass/energy is required.
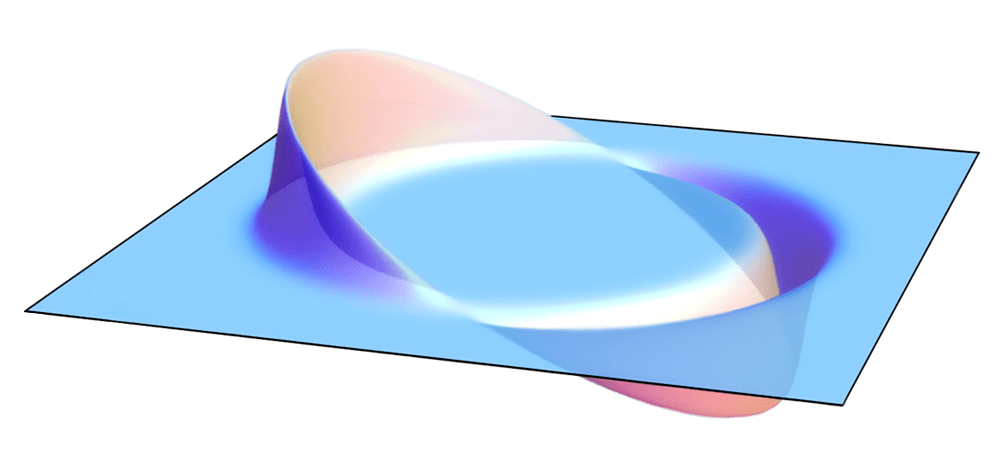
The hope of antimatter
However, as many were quick to point out, the fact that it takes energy to create particles and antiparticles of all types doesn’t necessarily imply that negative mass/energy states don’t exist. In fact, some argued, this assumes that Einstein’s equivalence principle is exactly correct, and that the type of mass that creates particles (i.e., the “m” in E = mc²) is the same as the type of mass/energy that causes spacetime to curve.
This had been tested for all different types of matter many times over, but throughout the 1990s, 2000s, and 2010s, had never been tested for the other type of massive quanta we can create: antiparticles.
If the laws of physics worked as expected, then matter and antimatter would behave in the same way: both curving space in proportion to their inherent energy, both gravitating the same way, and both “falling down” in the gravitational field of Earth. But this would prove difficult to test. Antimatter, remember, annihilates away with matter extremely easily: upon contact. The easiest antimatter particles to make, antiprotons and positrons, are electrically charged, and so are very difficult to “hold” in isolation. It was only at the one place in the world focused on creating and controlling antimatter — the antimatter factory at CERN, the same place that’s home to the Large Hadron Collider — that this could conceivably be tested.
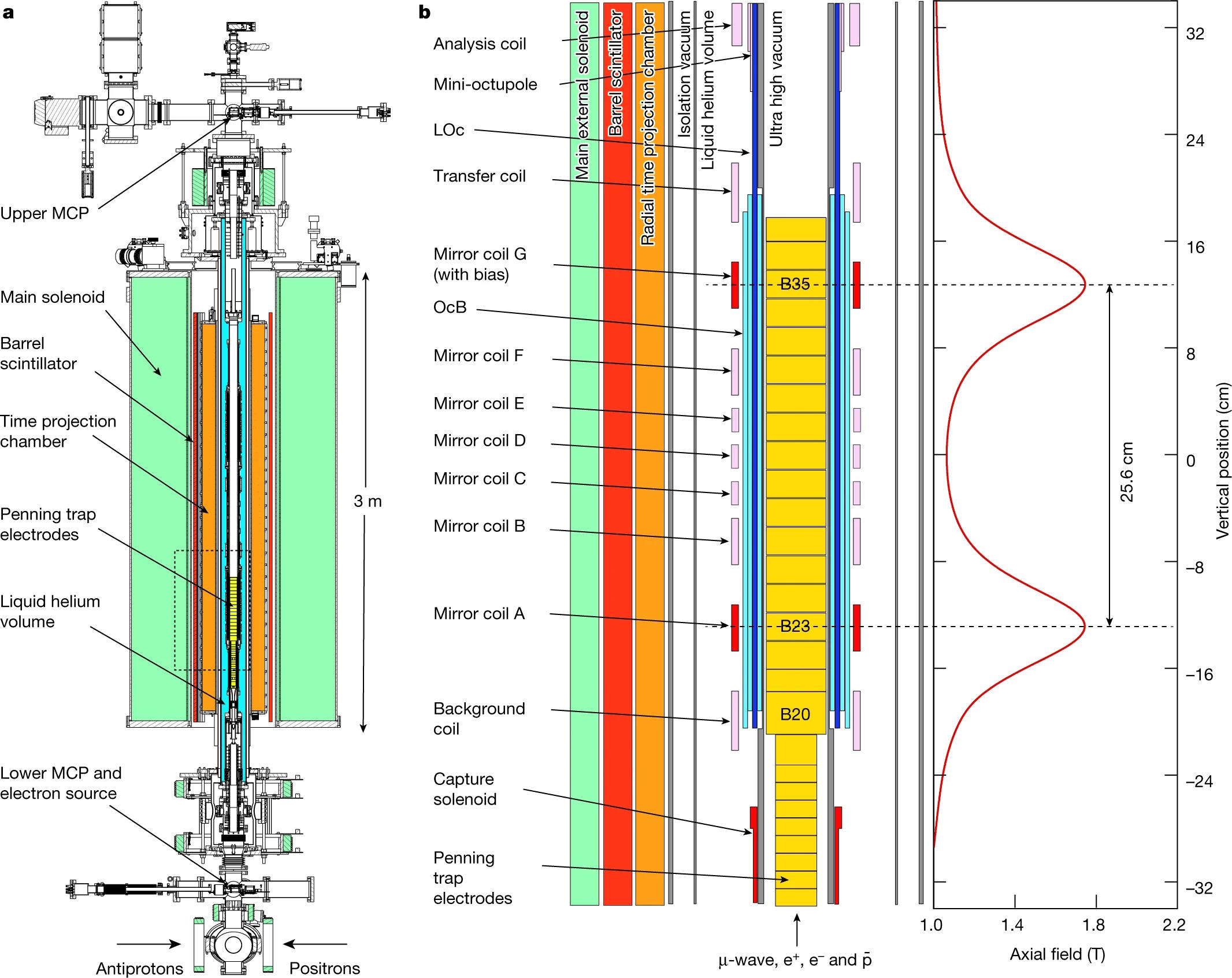
One such experiment at the antimatter factory is known as ALPHA: the Antihydrogen Laser PHysics Apparatus. Creating neutral antihydrogen atoms out of antiprotons and positrons and then confining them in a controlled environment, ALPHA scientists had previously measured the atomic spectrum of antihydrogen, confirming that the light it emitted and absorbed was identical to that of normal hydrogen.
In order to measure whether antimatter falls “up,” “down,” or in some entirely different fashion, ALPHA scientists needed to create a large number of antihydrogen atoms, sweep away all the fast-moving ones, and then let the slowest-moving ones fall, tracking their arrival down to the edges of the chamber.
After allowing the particles to fall and tracking their arrival times, ALPHA scientists then compared their results to simulations with:
- normal gravity,
- anti-gravity,
- and no gravity,
discovering, in a remarkable technical achievement, that the results were most consistent with antimatter experiencing normal gravity, and not any of the other options. In other words: antimatter doesn’t anti-gravitate, and with that, humanity’s best hope for achieving warp drive has just died.
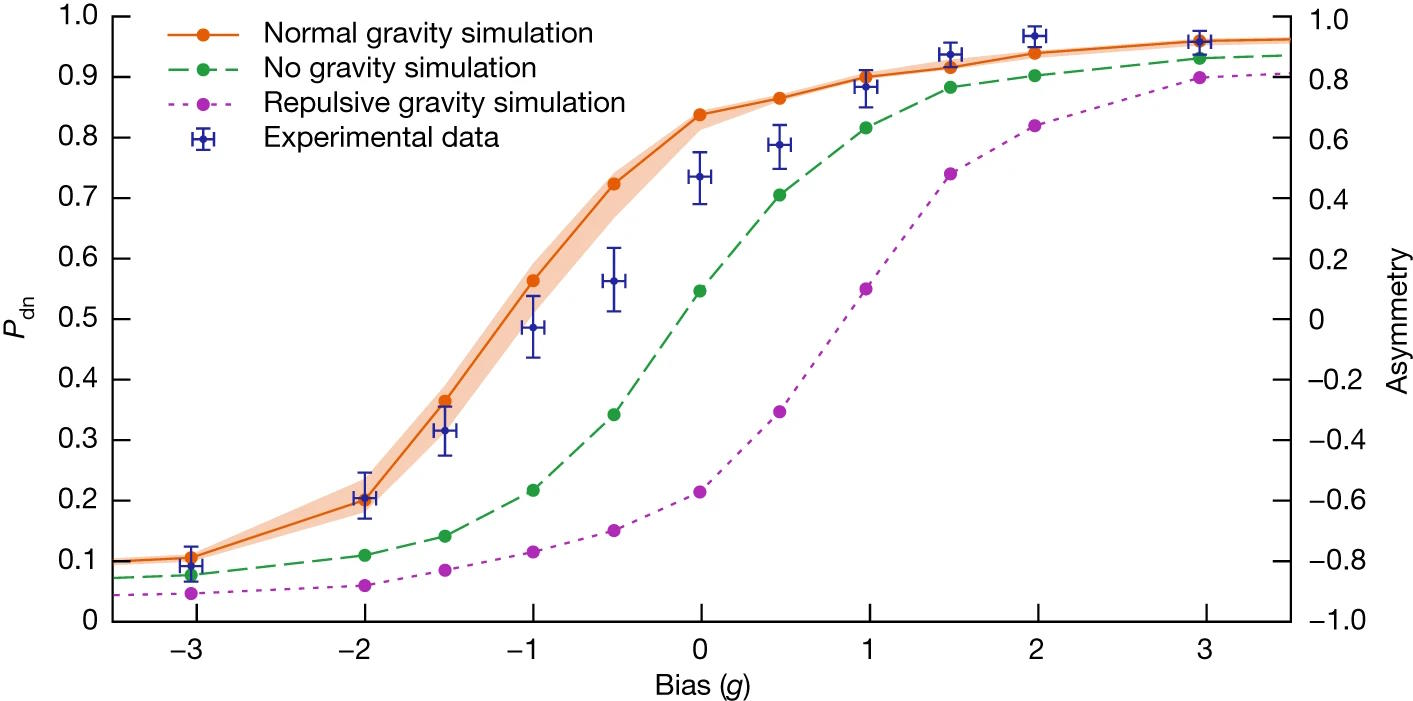
Also dying with this measurement is another sci-fi hope: artificial gravity that works without rotation or acceleration. If antimatter truly anti-gravitated, then simply by building a spacecraft’s floor out of normal matter and its ceiling out of antimatter, we could create a spacecraft that had its own built-in, automatic system for artificial gravity. But because antimatter doesn’t anti-gravitate but only gravitates normally, the only options for artificial gravity involve either constant linear acceleration or by having a rotating cylinder about its axis: as seen in 2001: A Space Odyssey or as envisioned by the science-fiction scenario of an O’Neill cylinder.
Although there are physicists who wonder “Why did we even need to do this experiment; we all knew that antimatter has positive mass,” that sentiment is absolutely foolish. We must remember — and I say this as a theoretical physicist myself — that physics is 100% an experimental science. We can be confident in our theory’s predictions only insofar as we can test and measure what it predicts; as soon as we step outside of the realm of what’s been validated by experiment, we run the risk of stepping outside the realm of where our theory is valid. We just learned that Einstein’s general relativity passed another test, the antimatter test, and with it, our greatest science-fiction hope for achieving warp drive has completely evaporated.
Author’s note: Ethan Siegel wrote the book on Star Trek’s technologies, and this scenario for a potentially realistic warp drive, outlined in the book, is now ruled out.