Mystery of ultra-fast solar flares solved by plasma physics
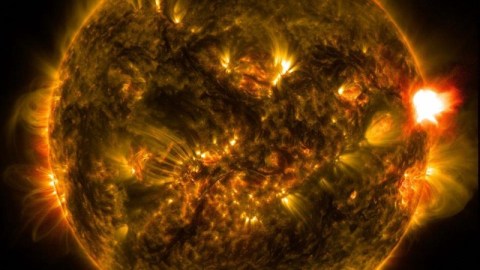
Magnetic reconnection this fast shouldn’t be possible, yet we see it everywhere we look. Finally, we know why.
“We are inside this plasma,
and plasma is inside everything. It is incandescent
in the sun, and I am curious to know if you
are able to stop orbiting yourself around it even for a second.”
–Marieta Maglas
Just like Earth and many other worlds, the Sun has a magnetic field that permeates throughout its interior and emerges far beyond its surface. The field is irregular over the surface, and oftentimes loops and other intricate structure can be seen. Plasma — the ionized matter found at the Sun’s edge and all throughout — often traces these magnetic structures. But every once in a while, these almost-always-tied-together field lines snap and rapidly reconnect, causing particles to stream outwards at incredible velocities. The reconnection speed has always been a mystery, occurring far more quickly than the equations would predict. Explanations have come and gone through the years; none has ever been satisfactory. But a new theoretical development, the science of plasmoid instability, appears have solved the puzzle at last.
Magnetic reconnection doesn’t just happen on the Sun, but in a wide variety of astrophysical and terrestrial phenomena. When charged particles fly from the Sun towards our world and then flow down the Earth’s magnetic field to create aurorae, that’s due to magnetic reconnection. When turbulent plasmas exist in interstellar space,magnetic reconnection causes electron heating, and the same mechanism may even power gamma-ray bursts. And right here on Earth, we can perform laboratory-based experiments to not only study the phenomenon itself, but its consequences, such as causing the hot, central plasma to mix with the cooler, outer plasma closer to the wall in magnetic fusion reactors.
The physics is pretty simple:
- Envision the magnetic field created by any number of bar magnets.
- Move those magnets around to different configurations relative to one another.
- Watch the lines disconnect from certain locations and reconnect in others as the fields change.
That’s it! That’s magnetic reconnection. Thanks to a series of space-based explorers, we’ve been able to observe and confirm the phenomenon of magnetic reconnection quite robustly, both in the emission of solar flares and in the auroral phenomenon here on Earth.
But, as with a great many things, the devil is in the details here.
In astrophysics, one of the most important details of plasmas is electric currents. Because plasmas are made up of ionized atoms and free electrons, including bare atomic nuclei, electric and magnetic fields can separate, move and accelerate these particles at incredible speeds. Moving charged particles make electric currents, and in one of these magnetized environments, those currents get compressed into thin layers — or sheets — that wind up getting expelled from the plasma entirely. The largest such current in our Solar System arises from the Sun and is known as the heliospheric current sheet. At around 10,000 kilometers in thickness, it extends past the orbit of Pluto in all directions.
It was thought, for a long time, that these thin current sheets needed to highly constrain the speed at which the magnetic field lines can split apart and reconnect; that’s what theoretical calculations predict. But physics is an experimental, measurable science for a reason, and our observations indicate unambiguously that the splitting and reconnection happens faster than that predicted speed. A team of physicists at the Princeton Plasma Physics Laboratory led by Luca Comisso performed a series of laboratory experiments that indicated the sought after solution was right in front of our faces the whole time: the plasma sheet isn’t a continuous, uniform entity, but rather can be broken up into small islands with their own unique magnetic properties. That’s what the “plasmoid instability” idea is.
The idea has been around for a few years, but the huge advance of Comisso’s team is that they were able — for the first time — to correctly determine the quantitative properties of the plasmoid instability that lead to fast magnetic reconnection in real situations. Ironically, it relied on one of the oldest physical principles of all, dating all the way back to Fermat (of Fermat’s last theorem) in the 1600s and the principle of least time. Here’s how this breaks down.
- A large current sheet behaves as the old, naïve model predicts: as a continuous, uniform entity where the magnetic field is mostly confined. In many ways, it’s like forming a thin sheet of plywood.
- Slight deviations from uniformity emerge, and plasmoid instabilities begin forming and growing at a uniform, linear rate. It’s like applying a small force to the plywood and watching it bend in response.
- As the outside magnetic properties continue to change — the Sun rotates, the Earth-Sun system switches from night to day, the field’s configuration shifts, etc. — the instabilities change less than they did before. It’s like increasing the force to the plywood and watching it bend less than you’d expect, as instead it just holds that tension in its material structure. This is an example of stored, potential energy.
- Finally, the magnetic properties have changed so much that the instabilities would be much more stably configured if the field lines rapidly shifted and reconnected. It’s here that the field lines break apart and reconnect, faster than any other model had predicted and in line with the observations. This is akin to the plywood simply snapping in two, and releasing that stored energy.
The beauty of this research is twofold: in its newfound predictive power and in the surprising lessons that were learned. The predictions that can now be made? How long “phase 2” above lasts, how many plasmoid instabilities will form, and what their growth rate and ultimate size will be. Coming up with a model that physically reproduces what experiments and observations bear out is a tremendous advance. But the team has also uncovered some surprising lessons. There are four quantities that grow/change over time (like the number of plasmoids and how long they take to reach the critical, reconnection phase) and three quantities that they depend on (like the sizes of the initial imperfections). Unlike most physical laws, which are power laws (i.e., xis proportional to y to some power), these dependences aren’t! As the authors say:
It is common in all realms of science to seek the existence of power laws, despite the fact that they are, sometimes, intrinsically simplistic. In contrast, we find that the scaling relations of the plasmoid instability are not true power laws — a result that has never been derived or predicted before.
If you ever wondered where solar flares came from before and how they get ejected so quickly, the answer lies in magnetic reconnection. For the first time, we finally understand and can predict exactly how this phenomenon works, in not just a qualitative but a quantitative fashion.
Reference: “General Theory of the Plasmoid Instability”, L. Comisso, M. Lingam, Y.-M. Huang, and A. Bhattacharjee, Phys. Plasmas 23, 100702 (2016). Preprint available at Arxiv.org.
This post first appeared at Forbes, and is brought to you ad-free by our Patreon supporters. Comment on our forum, & buy our first book: Beyond The Galaxy!