The Big Bang no longer means what it used to
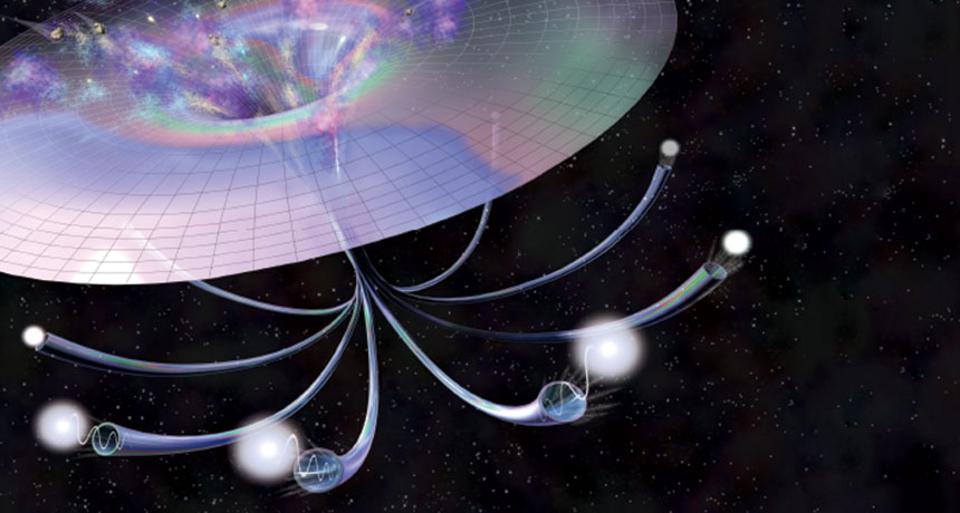
- The idea that the Universe had a beginning, or a “day without a yesterday” as it was originally known, goes all the way back to Georges LemaĆ®tre in 1927.
- Although it’s still a defensible position to state that the Universe likely had a beginning, that stage of our cosmic history has very little to do with the “hot Big Bang” that describes our early Universe.
- Although many laypersons (and even a minority of professionals) still cling to the idea that the Big Bang means “the very beginning of it all,” that definition is decades out of date. Here’s how to get caught up.
If there’s one hallmark inherent to science, it’s that our understanding of how the Universe works is always open to revision in the face of new evidence. Whenever our prevailing picture of reality — including the rules it plays by, the physical contents of a system, and how it evolved from its initial conditions to the present time — gets challenged by new experimental or observational data, we must open our minds to changing our conceptual picture of the cosmos. This has happened many times since the dawn of the 20th century, and the words we use to describe our Universe have shifted in meaning as our understanding has evolved.
Yet, there are always those who cling to the old definitions, much like linguistic prescriptivists, who refuse to acknowledge that these changes have occurred. But unlike the evolution of colloquial language, which is largely arbitrary, the evolution of scientific terms must reflect our current understanding of reality. Whenever we talk about the origin of our Universe, the term “the Big Bang” comes to mind, but our understanding of our cosmic origins have evolved tremendously since the idea that our Universe even had an origin, scientifically, was first put forth. Here’s how to resolve the confusion and bring you up to speed on what the Big Bang originally meant versus what it means today.
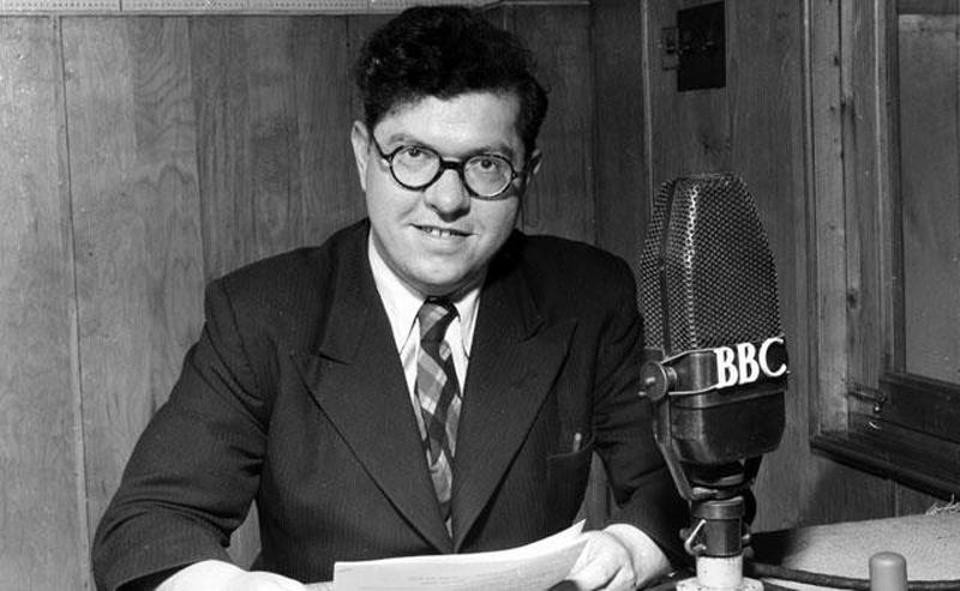
The first time the phrase “the Big Bang” was uttered was over 20 years after the idea was first described. In fact, the term itself comes from one of the theory’s greatest detractors: Fred Hoyle, who was a staunch advocate of the rival idea of a Steady-State cosmology. In 1949, he appeared on BBC radio and advocated for what he called the perfect cosmological principle: the notion that the Universe was homogeneous in both space and time, meaning that any observer not only anywhere but anywhen would perceive the Universe to be in the same cosmic state. He went on to deride the opposing notion as a “hypothesis that all matter of the universe was created in one Big Bang at a particular time in the remote past,” which he then called “irrational” and claimed to be “outside science.”
But the idea, in its original form, wasn’t simply that all of the Universe’s matter was created in one moment in the finite past. That notion, derided by Hoyle, had already evolved from its original meaning. Originally, the idea was that the Universe itself, not just the matter within it, had emerged from a state of non-being in the finite past. And that idea, as wild as it sounds, was an inevitable but difficult-to-accept consequence of the new theory of gravity put forth by Einstein back in 1915: General Relativity.
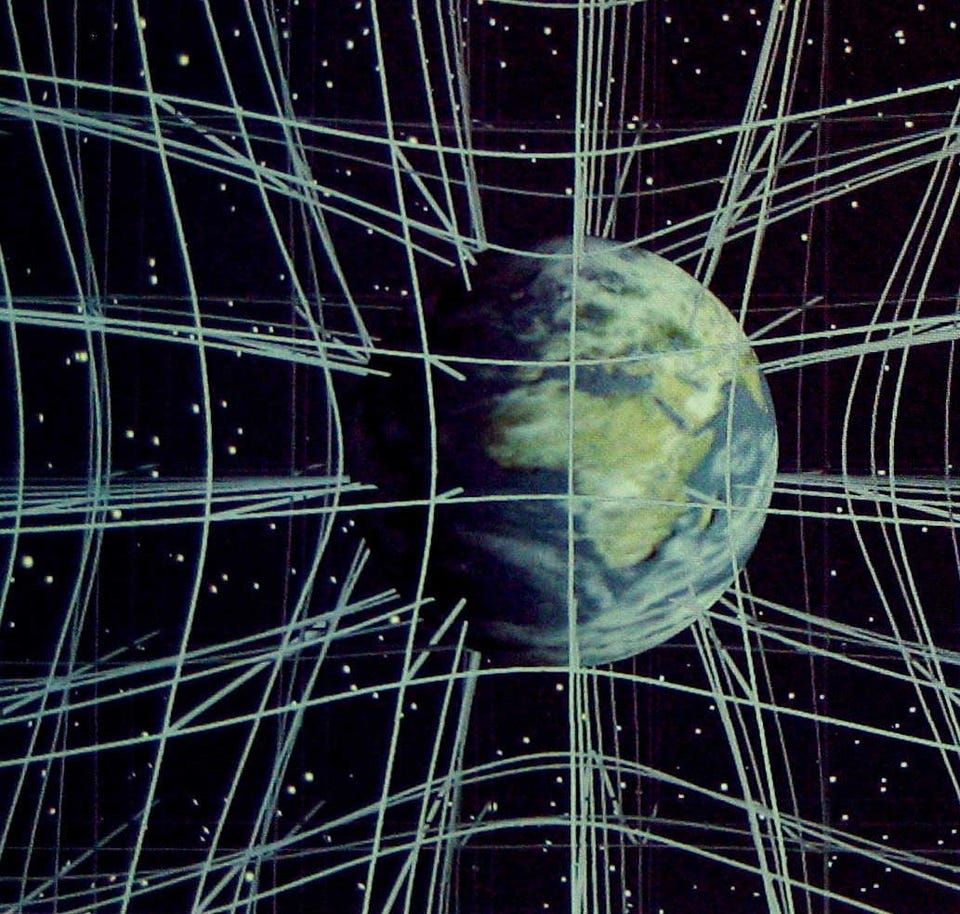
When Einstein first cooked up the general theory of relativity, our conception of gravity forever shifted from the prevailing notion of Newtonian gravity. Under Newton’s laws, the way that gravitation worked was that any and all masses in the Universe exerted a force on one another, instantaneously across space, in direct proportion to the product of their masses and inversely proportional to the square of the distance between them. But in the aftermath of his discovery of special relativity, Einstein and many others quickly recognized that there was no such thing as a universally applicable definition of what “distance” was or even what “instantaneously” meant with respect to two different locations.
With the introduction of Einsteinian relativity — the notion that observers in different frames of reference would all have their own unique, equally valid perspectives on what distances between objects were and how the passage of time worked — it was only almost immediate that the previously absolute concepts of “space” and “time” were woven together into a single fabric: spacetime. All objects in the Universe moved through this fabric, and the task for a novel theory of gravity would be to explain how not just masses, but all forms of energy, shaped this fabric that underpinned the Universe itself.
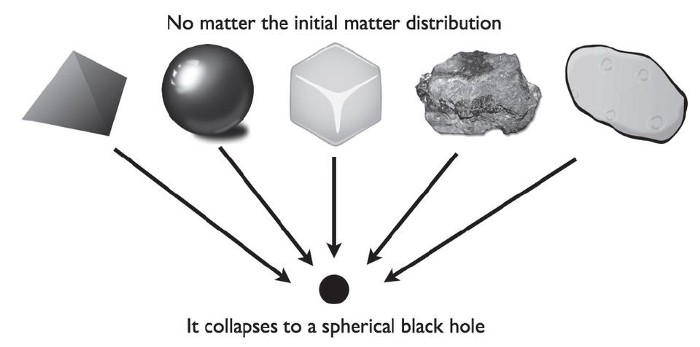
Although the laws that governed how gravitation worked in our Universe were put forth in 1915, the critical information about how our Universe was structured had not yet come in. While some astronomers favored the notion that many objects in the sky were actually “island Universes” that were located well outside the Milky Way galaxy, most astronomers at the time thought that the Milky Way galaxy represented the full extent of the Universe. Einstein sided with this latter view, and — thinking the Universe was static and eternal — added a special type of fudge factor into his equations: a cosmological constant.
Although it was mathematically permissible to make this addition, the reason Einstein did so was because without one, the laws of General Relativity would ensure that a Universe that was evenly, uniformly distributed with matter (which ours seemed to be) would be unstable against gravitational collapse. In fact, it was very easy to demonstrate that any initially uniform distribution of motionless matter, regardless of shape or size, would inevitably collapse into a singular state under its own gravitational pull. By introducing this extra term of a cosmological constant, Einstein could tune it so that it would balance out the inward pull of gravity by proverbially pushing the Universe out with an equal and opposing action.
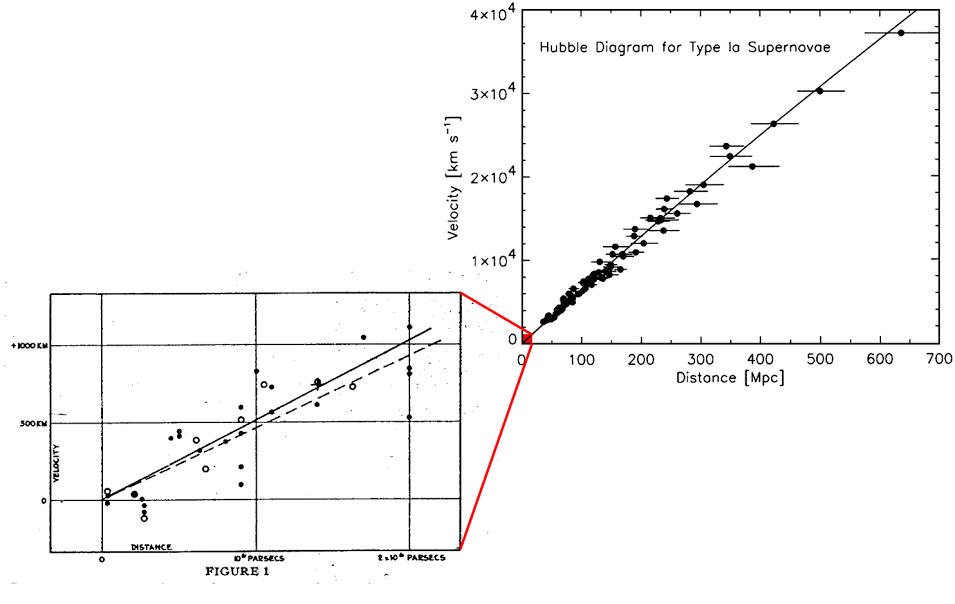
Two developments — one theoretical and one observational — would quickly change this early story that Einstein and others had told themselves.
- In 1922, Alexander Friedmann worked out, fully, the equations that governed a Universe that was isotropically (the same in all directions) and homogeneously (the same in all locations) filled with any type of matter, radiation, or other form of energy. He found that such a Universe would never remain static, not even in the presence of a cosmological constant, and that it must either expand or contract, dependent on the specifics of its initial conditions.
- In 1923, Edwin Hubble became the first to determine that the spiral nebulae in our skies were not contained within the Milky Way, but rather were located many times farther away than any of the objects that comprised our home galaxy. The spirals and ellipticals found throughout the Universe were, in fact, their own “island Universes,” now known as galaxies, and that moreover — as had previously been observed by Vesto Slipher — the vast majority of them appeared to be moving away from us at remarkably rapid speeds.
In 1927, Georges Lemaître became the very first person to put these pieces of information together, recognizing that the Universe today is expanding, and that if things are getting farther apart and less dense today, then they must have been closer together and denser in the past. Extrapolating this back all the way to its logical conclusion, he deduced that the Universe must have expanded to its present state from a single point-of-origin, which he called either the “cosmic egg” or the “primeval atom.”
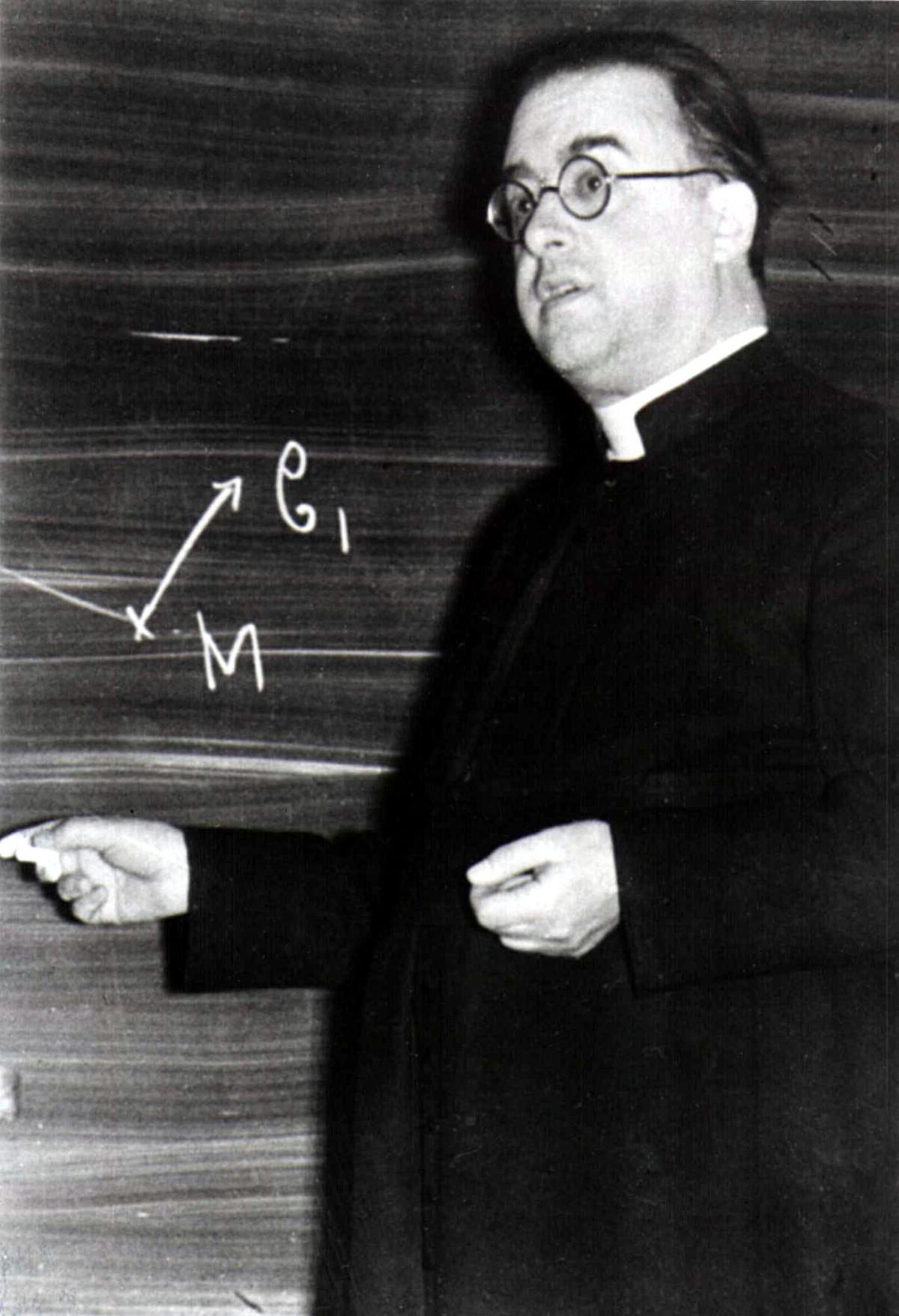
This was the original notion of what would grow into the modern theory of the Big Bang: the idea that the Universe had a beginning, or a “day without yesterday.” It was not, however, generally accepted for some time. Lemaître originally sent his ideas to Einstein, who infamously dismissed Lemaître’s work by responding, “Your calculations are correct, but your physics is abominable.”
Despite the resistance to his ideas, however, Lemaître would be vindicated by further observations of the Universe. Many more galaxies would have their distances and redshifts measured, leading to the overwhelming conclusion the Universe was and still is expanding, equally and uniformly in all directions on large cosmic scales. In the 1930s, Einstein conceded, referring to his introduction of the cosmological constant in an attempt to keep the Universe static as his “greatest blunder.”
However, the next great development in formulating what we know of as the Big Bang wouldn’t come until the 1940s, when George Gamow — perhaps not so coincidentally, an advisee of Alexander Friedmann — came along. In a remarkable leap forward, he recognized that the Universe was not only full of matter, but also radiation, and that radiation evolved somewhat differently from matter in an expanding Universe. This would be of little consequence today, but in the early stages of the Universe, it mattered tremendously.

Matter, Gamow realized, was made up of particles, and as the Universe expanded and the volume that these particles occupied increased, the number density of matter particles would drop in direct proportion to how the volume grew.
But radiation, while also made up of a fixed number particles in the form of photons, had an additional property: the energy inherent to each photon is determined by the photon’s wavelength. As the Universe expands, the wavelength of each photon gets lengthened by the expansion, meaning that the amount of energy present in the form of radiation decreases faster than the amount of energy present in the form of matter in the expanding Universe.
But in the past, when the Universe was smaller, the opposite would have been true. If we were to extrapolate backward in time, the Universe would have been in a hotter, denser, more radiation-dominated state. Gamow leveraged this fact to make three great, generic predictions about the young Universe.
- At some point, the Universe’s radiation was hot enough so that every neutral atom would have been ionized by a quantum of radiation, and that this leftover bath of radiation should still persist today at only a few degrees above absolute zero.
- At some even earlier point, it would have been too hot to even form stable atomic nuclei, and so an early stage of nuclear fusion should have occurred, where an initial mix of protons-and-neutrons should have fused together to create an initial set of atomic nuclei: an abundance of elements that predates the formation of atoms.
- And finally, this means that there would be some point in the Universe’s history, after atoms had formed, where gravitation pulled this matter together into clumps, leading to the formation of stars and galaxies for the first time.

These three major points, along with the already-observed expansion of the Universe, form what we know today as the four cornerstones of the Big Bang. Although one was still free to extrapolate the Universe back to an arbitrarily small, dense state — even to a singularity, if you’re daring enough to do so — that was no longer the part of the Big Bang theory that had any predictive power to it. Instead, it was the emergence of the Universe from a hot, dense state that led to our concrete predictions about the Universe.
Over the 1960s and 1970s, as well as ever since, a combination of observational and theoretical advances unequivocally demonstrated the success of the Big Bang in describing our Universe and predicting its properties.
- The discovery of the cosmic microwave background and the subsequent measurement of its temperature and the blackbody nature of its spectrum eliminated alternative theories like the Steady State model.
- The measured abundances of the light elements throughout the Universe verified the predictions of Big Bang nucleosynthesis, while also demonstrating the need for fusion in stars to provide the heavy elements in our cosmos.
- And the farther away we look in space, the less grown-up and evolved galaxies and stellar populations appear to be, while the largest-scale structures like galaxy groups and clusters are less rich and abundant the farther back we look.
The Big Bang, as verified by our observations, accurately and precisely describes the emergence of our Universe, as we see it, from a hot, dense, almost-perfectly uniform early stage.
But what about the “beginning of time?” What about the original idea of a singularity, and an arbitrarily hot, dense state from which space and time themselves could have first emerged?

That’s a different conversation, today, than it was back in the 1970s and earlier. Back then, we knew that we could extrapolate the hot Big Bang back in time: back to the first fraction-of-a-second of the observable Universe’s history. Between what we could learn from particle colliders and what we could observe in the deepest depths of space, we had lots of evidence that this picture accurately described our Universe.
But at the absolute earliest times, this picture breaks down. There was a new idea — proposed and developed in the 1980s — known as cosmological inflation, that made a slew of predictions that contrasted with those that arose from the idea of a singularity at the start of the hot Big Bang. In particular, inflation predicted:
- A curvature for the Universe that was indistinguishable from flat, to the level of between 99.99% and 99.9999%; comparably, a singularly hot Universe made no prediction at all.
- Equal temperatures and properties for the Universe even in causally disconnected regions; a Universe with a singular beginning made no such prediction.
- A Universe devoid of exotic high-energy relics like magnetic monopoles; an arbitrarily hot Universe would possess them.
- A Universe seeded with small-magnitude fluctuations that were almost, but not perfectly, scale invariant; a non-inflationary Universe produces large-magnitude fluctuations that conflict with observations.
- A Universe where 100% of the fluctuations are adiabatic and 0% are isocurvature; a non-inflationary Universe has no preference.
- A Universe with fluctuations on scales larger than the cosmic horizon; a Universe originating solely from a hot Big Bang cannot have them.
- And a Universe that reached a finite maximum temperature that’s well below the Planck scale; as opposed to one whose maximum temperature reached all the way up to that energy scale.
The first three were post-dictions of inflation; the latter four were predictions that had not yet been observed when they were made. On all of these accounts, the inflationary picture has succeeded in ways that the hot Big Bang, without inflation, has not.
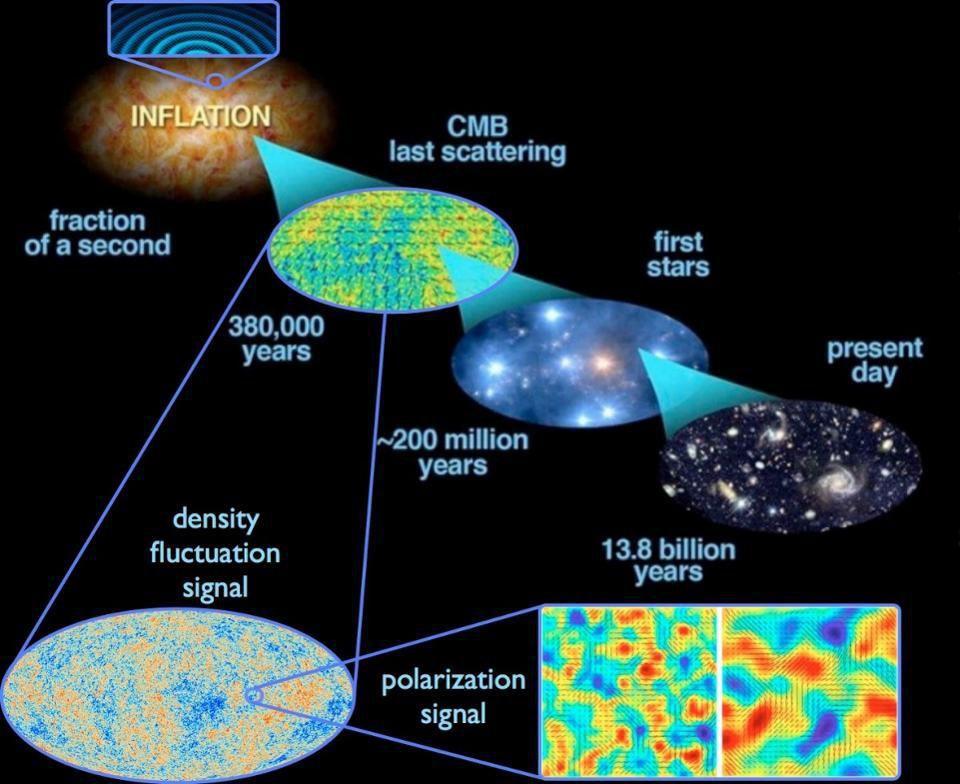
During inflation, the Universe must have been devoid of matter-and-radiation and instead contained some sort of energy — whether inherent to space or as part of a field — that didn’t dilute as the Universe expanded. This means that inflationary expansion, unlike matter-and-radiation, didn’t follow a power law that leads back to a singularity but rather is exponential in character. One of the fascinating aspects about this is that something that increases exponentially, even if you extrapolate it back to arbitrarily early times, even to a time where t → -∞, it never reaches a singular beginning.
Now, there are many reasons to believe that the inflationary state wasn’t one that was eternal to the past, that there might have been a pre-inflationary state that gave rise to inflation, and that, whatever that pre-inflationary state was, perhaps it did have a beginning. There are theorems that have been proven and loopholes discovered to those theorems, some of which have been closed and some of which remain open, and this remains an active and exciting area of research.
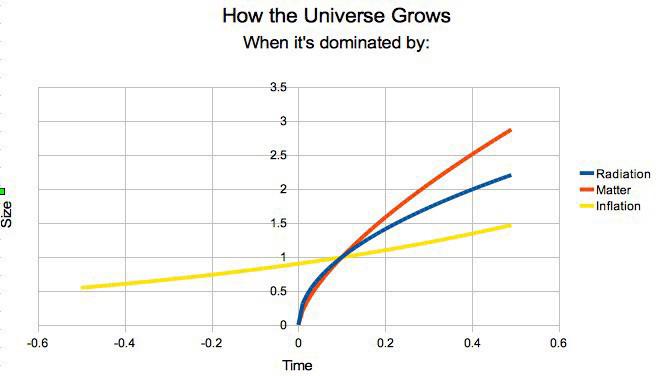
But one thing is for certain.
Whether there was a singular, ultimate beginning to all of existence or not, it no longer has anything to do with the hot Big Bang that describes our Universe from the moment that:
- inflation ended,
- the hot Big Bang occurred,
- the Universe became filled with matter and radiation and more,
- and it began expanding, cooling, and gravitating,
eventually leading to the present day. There are still a minority of astronomers, astrophysicists and cosmologists who use “the Big Bang” to refer to this theorized beginning and emergence of time-and-space, but not only is that not a foregone conclusion anymore, but it doesn’t have anything to do with the hot Big Bang that gave rise to our Universe. The original definition of the Big Bang has now changed, just as our understanding of the Universe has changed. If you’re still behind, that’s ok; the best time to catch up is always right now.
Additional recommended reading:
- Ask Ethan: Do we know why the Big Bang really happened? (evidence for cosmic inflation)
- Surprise: the Big Bang isn’t the beginning of the universe anymore (why a “singularity” is no longer necessarily a given)