Ask Ethan #85: Hubble vs. The Big Bang
How do we know that the fluctuations in the cosmic microwave background aren’t polluted by everything Hubble reveals?
“Silently, one by one, in the infinite meadows of heaven,
Blossomed the lovely stars, the forget-me-nots of the angels.” –Longfellow
With today marking the 25th anniversary of the launch of the Hubble Space Telescope, it’s only fitting that — as I dive into the questions and suggestions you’ve sent in — I pulled out one from Gerard that looks at two things you might not thing are related, but that turn out to be. He asks the following:
Scientists talk about near perfect uniformity of the CMB. How do they know the measured differences in uniformity [aren’t] just due to the error in not making perfect corrections for the galaxies in the field of view of the measuring telescopes?
At first, you might not think this is related to Hubble, but it very much is. Let’s go back to the very beginning and see how the story unfolds.

The hot Big Bang starts off with just a hot, dense soup of particles, antiparticles and radiation at incredible temperatures. It’s almost perfectly smooth and uniform, but not quite. Inflation — the phenomenon that preceded and set up the hot Big Bang — stretched the tiny quantum fluctuations that always occur everywhere in space across the entire Universe, creating a set of overdense and underdense regions.
In addition to all of that, this hot, dense Universe is also expanding. As gravitation works to pull everything together, attracting more and more matter-and-energy to the overdense regions and trying to recollapse the Universe on all scales. While this struggle between gravitation and expansion occurs, the Universe cools, since the expanding Universe not only causes the amount of stuff-per-unit-volume to dilute, it also stretches the wavelength of any light present.

After the Universe cools enough so that symmetries break and particles obtain mass, excess particle-antiparticle pairs annihilate away, and protons and neutrons form into stable atomic nuclei, finally you can form stable, neutral atoms for the first time, as the leftover radiation is too low in energy to ionize those atoms once again. At this point, the leftover glow from the Big Bang — all of those photons — are free to travel in a straight line unimpeded, as the free electrons that had been causing them to scatter are finally taken out of the equation.

This radiation itself at this time is pretty much perfectly uniform. And the radiation as we’d see it is almost perfectly uniform, but not quite. Not only did inflation create slightly overdense and underdense regions, but on certain scales (preferentially smaller ones), gravitation will have worked to grow (or wash out, on other scales, along with the interplay of radiation) the magnitudes of these overdense and underdense regions.
So how come the radiation itself is perfectly uniform, but we won’t see it that way?
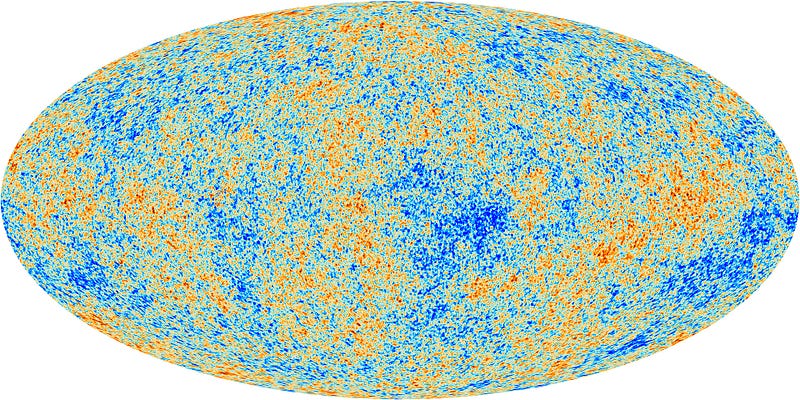
Remember the most important concept that Einstein’s general relativity introduced: the idea that space is curved by the presence of matter and energy. If you have an overdense region of space — more matter and more energy — space is curved more severely at that location, meaning that any light that falls into that region gets blueshifted, and any light that climbs out of that region gets redshifted.
So if all the light is actually the same temperature, but some regions are more (or less) dense than average, what does that mean for the light once it’s completely climbed out of that region and on its way to our eyes?

It means that the denser regions appear colder, due to a higher-than-average gravitational redshift, while the less dense regions will appear hotter, thanks to a below-average gravitational redshift. This is known as the Sachs-Wolfe effect.
When we look at the best “baby picture” of the Universe, or the fluctuations in the cosmic microwave background (CMB), that’s exactly what we expect it is that we’re seeing: the cold spots will correspond to overdense regions that will someday grow — thanks to gravity — into richer-than-average areas of stars, galaxies and groups-and-clusters of galaxies. And on the flipside, hot spots are the underdense regions that will give up, on average, a greater amount of their matter to the surrounding regions that are more dense, and so will wind up with fewer-than-average stars, galaxies and clusters.
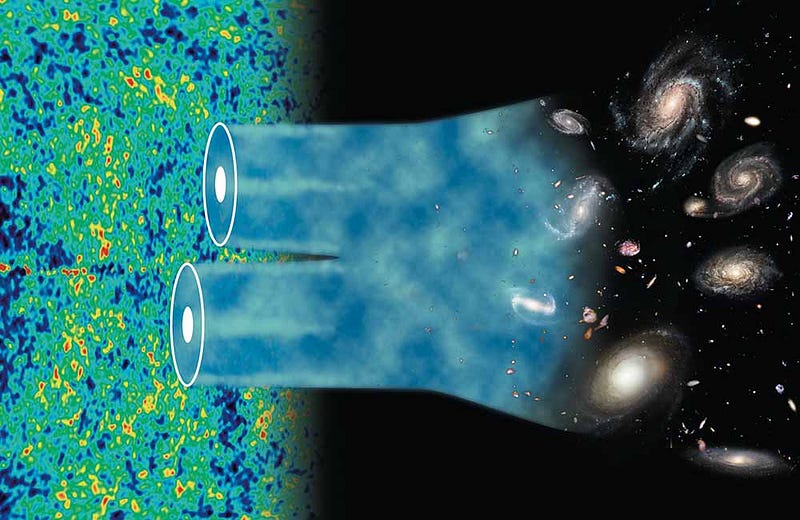
But what about all the stars, galaxies and clusters that are out there? Surely, they cause these same effects: gravitational redshifts when these primordial amounts of radiation climb out of those wells. After all — as Hubble has taught us — the Universe is full of galaxies, even in regions of space where we can’t see them without super-long exposures.


But that won’t cause any trouble by itself. You see, the photon was blueshifted by a certain amount when it first fell into the galaxy, and was only afterwards redshifted by the same amount when it climbed back out!
There are two main effects that can change a photon’s energy when such an event happens, though, and both of them actually do affect the CMB:
- The gas in the galaxies/clusters, both due to its temperature and to its motion, can cause a shift in the temperature of the CMB. This is known as the Sunyaev-Zel’dovich effect (both the thermal and kinematic components, respectively), and has been both predicted and detected.
- The gravitational potentials of these objects — whether they’re overdense or underdense — can grow-or-shrink during the time it takes a photon to fall in and then escape, changing its energy over time. This is known as the Integrated Sachs-Wolfe effect, and it actually plays a role on large scale fluctuations, particularly at late times.

In fact, one of the things that was difficult to explain for some time was the existence of a large-scale spot in the Universe that was too cold for what theoretically should have been there; a spot that large and that cold should not have existed if the Universe formed the way I just described to you.
But after an intense galaxy survey of the area, we determined that there were about 20% fewer galaxies than average in this huge region, meaning that this is a great cosmic void, changing its gravitational potential due to the Integrated Sachs-Wolfe effect and causing the CMB light that passes through this to be extra redshifted, or colder than average.

When you take this into account, you wind up finding that the cold spot originating from the CMB is just a normal cold spot, and this “supervoid” that caused the extra chilling of this region of space was simply a run-of-the-mill underdense region on a large scale. Two completely normal things just happened to line up, making it appear that the CMB was behaving bizarrely. But in reality, Gerard, it’s actually the opposite situation from what you feared: by correlating the galaxy maps with the CMB, we can actually come to better understand what our Universe looked like when it was born, before any gravitational or astrophysical effects played a role!

Yet another spectacular achievement for astronomy and astrophysics, and every telescope that’s ever observed the night sky — including Hubble — helped contribute to our understanding of this.
Thanks for a great question and for another fantastic week. If you’ve got a question or suggestion for the next Ask Ethan, go for it, and maybe you’ll be featured right here, on Starts With A Bang!
Leave your comments at the Starts With A Bang forum on Scienceblogs!