Ask Ethan #92: Is there a limit to temperature?
If you took all the energy out of something, you’d reach absolute zero, the coldest temperature of all. But is there a highest temperature?
“Nothing is lost… Everything is transformed.” –Michael Ende
At the end of every week here on Starts With A Bang, we take a look at the questions and suggestions that have been submitted for our weekly Ask Ethan column. As voted on by our Patreon supporters, this week’s honor goes to schoolteacher Cameron Peters, who asks:
I teach 8th grade science and my students have been learning about heat and temperature. As part of this we’ve looked at the concept of absolute zero, what it means and how it relates to the motion of atoms. My students want to know if there is a maximum temperature that can occur in nature, or is there no upper limit.
Let’s start off with what an 8th grader would know, and go up in temperature from there.
Take this classic experiment: dropping food coloring into water of different temperatures. What are you going to see? The hotter the water’s temperature is, the faster the food coloring is going to diffuse throughout the water.
Now, why does this happen? Because the temperature of the molecules is directly related to the kinetic motions — and speeds — of the particles involved. This means that the hotter water has the individual water molecules within it moving around at higher speeds, and also that the food coloring particles will get transported around more rapidly in hotter water than in colder water.

If you were to stop all of this motion entirely — to bring everything to perfect rest (even overcoming the nature of quantum physics to do so) — that would enable you to reach absolute zero: the coldest possible thermodynamic temperature.
But what about going in the other direction? If you heat a system of particles up, surely they’ll just start moving faster and faster. But is there a limit to how high you can heat them, and is there some sort of catastrophe you’ll run into that prevents you from getting any hotter than some limit? Let’s see!
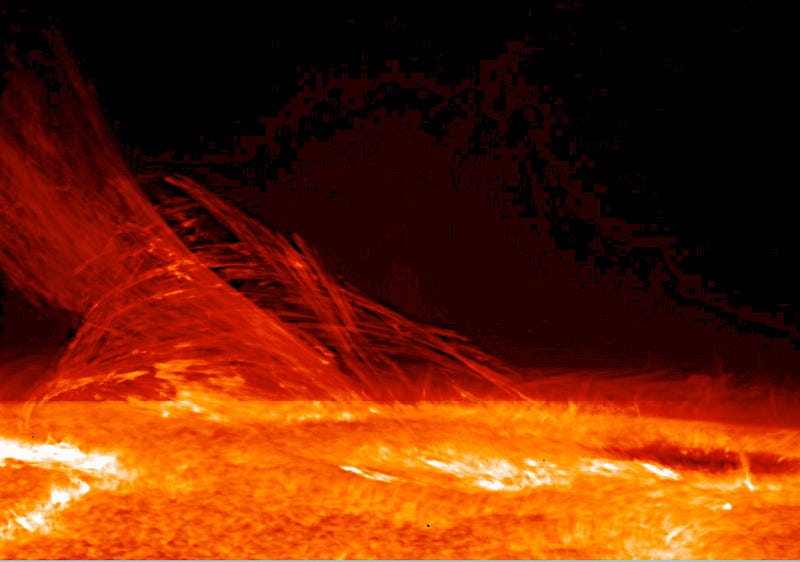
At temperatures of thousands of Kelvin, the heat you impart to your molecules will start to destroy the very bonds holding those molecules together, and if you continue to turn up the heat, will begin stripping the electrons off of the atoms themselves. You’ll wind up with an ionized plasma, something made exclusively out of electrons and atomic nuclei, with no neutral atoms at all.
But this is still just fine: the individual particles in there — the electrons and the positive ions — are perfectly content to bounce around at these high temperatures, obeying the same laws of physics as always. And you’re still free to turn up the heat, and see what happens next.
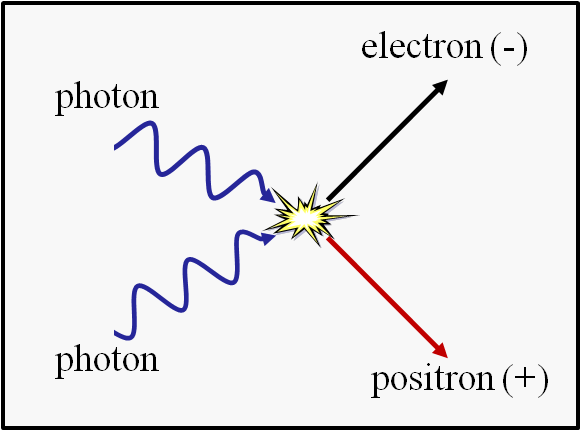
As you go up-and-up in temperature, the individual entities you think of as “particles” start to break down.
- At approximately 8 × 10^9 Kelvin (8 billion K), you start to spontaneously produce matter-and-antimatter pairs — electrons and positrons — from the raw energies of particle collisions with one another.
- At around 2 × 10^10 Kelvin (20 billion K), atomic nuclei are spontaneously blasted apart into individual protons and neutrons.
- At around 2 × 10^12 Kelvin (2 trillion K), protons and neutrons cease to exist, and instead the fundamental particles that make them up — quarks and gluons — start to bang around, unbound at these high energies.
- And at approximately 2 × 10^15 Kelvin (2 quadrillion K), you start producing all the known particles and antiparticles in vast quantities
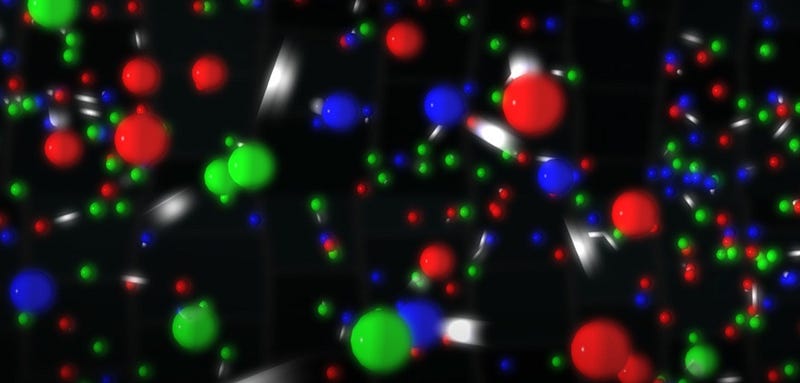
This, still, isn’t an upper limit, not by a long shot. Right around this 2 × 10^15 Kelvin (2 quadrillion K) threshold, something else interesting happens. You see, this is right around the energy you need to produce the Higgs boson, and hence is also right around the energy you need to restore one of the most fundamental symmetries in the Universe: the symmetry that gives particles their rest mass.
In other words, once you heated up your system to higher than this energy threshold, you’d discover that all of your particles were now massless, and flew around at the speed of light. Instead of what you think of as a mix of matter, antimatter and radiation, everything would behave as though it were radiation, whether it were actually matter, antimatter or none-of-the-above.
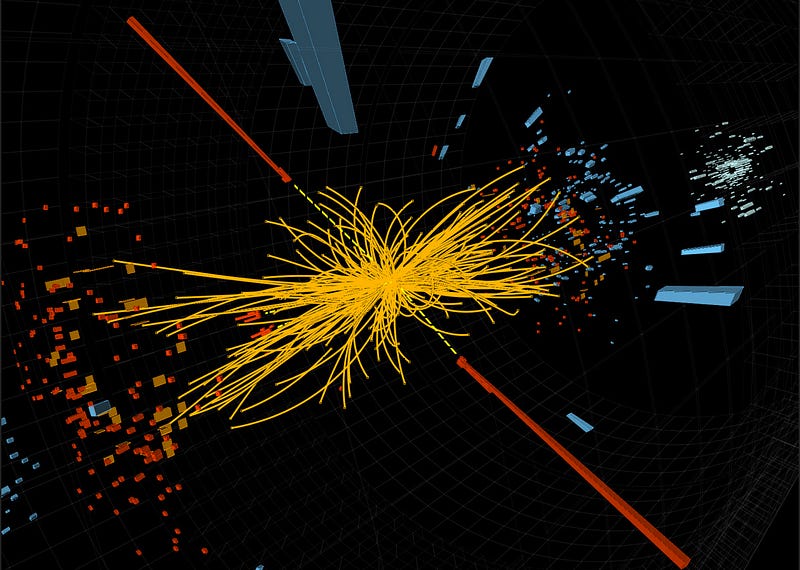
But we’re not done. You can keep heating up your system to higher and higher temperatures, and even though everything within it won’t move any faster, it will become more energetic, the same way that radio waves, microwaves, visible light and X-rays are all forms of light (and move at the speed of light) even though they have vastly different energies.
There may be as-of-yet-unknown new particles that get created, or new laws (or symmetries) of nature that come into play. You might think you can just go all the way up — hotter and hotter — up to infinite energies.
There are three reasons why this is impossible, however.
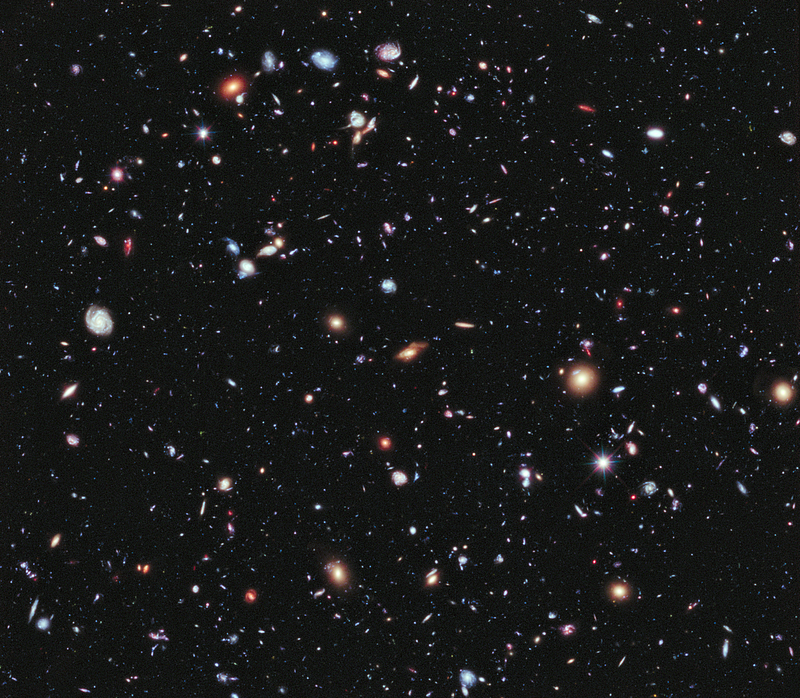
1.) There’s only a finite amount of energy present in the entire observable Universe. Take everything that exists in our spacetime: all the matter, antimatter, radiation, neutrinos, dark matter, even the energy inherent to space itself, and it’s huge. There are some 10^80 particles of normal matter, about 10^89 neutrinos and antineutrinos, slightly more photons, plus all the energy in dark matter and dark energy that’s spread out over the 46 billion light year radius of the observable Universe that’s centered on our position.
But even if you were to turn all of it into pure energy (via E = mc^2), and even if you were to use all of that energy to heat up your system, you won’t have an infinite amount of energy to play with. If you put all of it into a single system, you’d get a huge amount of energy, corresponding to a temperature of around 10^103 Kelvin, but that’s still not infinite. So there is an upper limit. But before you ever got up to that point, something else would stop you…

2.) If you put too much amount of energy together in any confined region of space, you’ll create a black hole! You normally think of black holes as being huge, massive, dense objects, capable of swallowing hordes of entire planets the same way cookie monster might swallow an entire box of cookies: sloppily, easily, and thoughtlessly.

The thing is, if you gave an individual quantum particle enough energy — even if it was just a massless particle moving at the speed of light — it would turn into a black hole! There’s a scale at which simply having something with a certain amount of energy in it will mean that it can’t interact as particles normally do, and that if you had particles reach this energy, the equivalent of 22 micrograms by E = mc^2, you’d only be able to get up to 10^19 GeV of energy or so before your system refused to get any hotter. You’d spontaneously produce these black holes which would immediately decay into a state of lower-energy, thermal radiation. So it seems that this energy scale — the Planck Scale — is the upper limit for our Universe, and that “only” corresponds to a temperature of approximately 10^32 Kelvin.
So that’s a lot lower than the previous limit, because not only is the Universe finite, but black holes are limiting factors. But there’s something else that’s a limiting factor, and it’s the big thing I would worry about if I had the ability to raise temperatures to arbitrary scales.
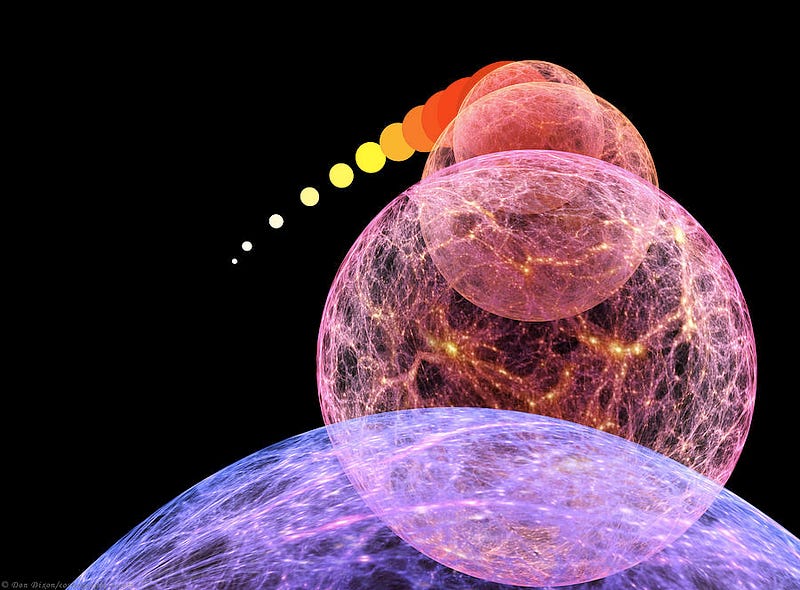
3.) At some high temperature, you will restore the potential that caused our Universe to inflate, cosmically. Back before the Big Bang, the Universe was undergoing a state of exponential expansion, where space itself was inflating like a cosmic balloon, but at an exponential rate. All the particles, antiparticles and radiation within it were rapidly separated from every other quantum bit of matter and energy, and when inflation came to an end, the Big Bang began.
If you managed to reach temperatures sufficient to bring this field back into its inflating state, you would effectively hit the “reset” button on the Universe, and cause inflation to resume, resulting in the Big Bang starting all over again.
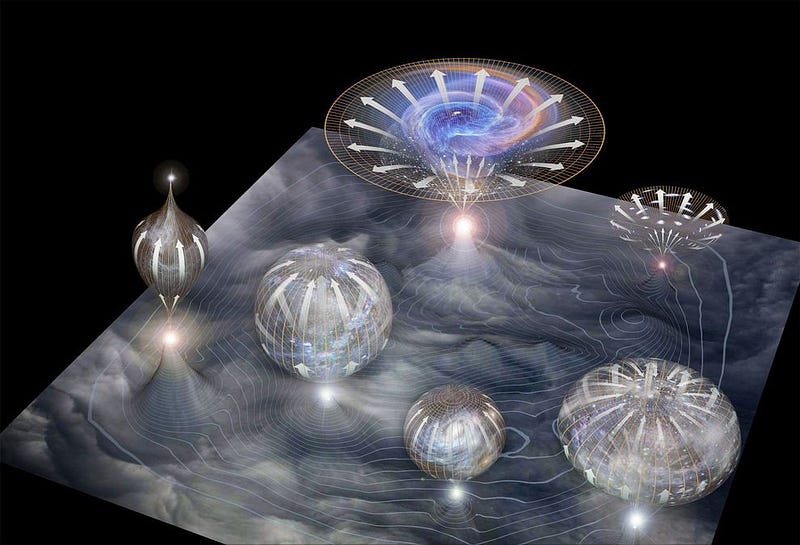
If that’s too technical for you, take this away: if you managed to get up to the temperature necessary to cause this effect, you would not survive. This is theorized to occur at temperatures of around 10^28–10^29 K, although there is a fair bit of wiggle room there, dependent upon what the actual scale of inflation happens to be.
So you can easily get up to very, very high temperatures. While the physical phenomena you’re used to will be very different in detail, you’ll still be able to make it rise, higher and higher, but only to a point before you destroy absolutely everything you ever held dear. So be careful, students of Mr. Peters, but don’t be fearful of the LHC. Even at the most powerful particle accelerator on Earth, we’re still a factor of at least 100 billion in energy away from risking this ill effect.
Submit your questions for Ask Ethan here, and I’ll see you back for more next week!
Leave your comments at our forum, and support Starts With A Bang on Patreon!