Ask Ethan: Could The Universe’s Missing Antimatter Be Found Inside Black Holes?
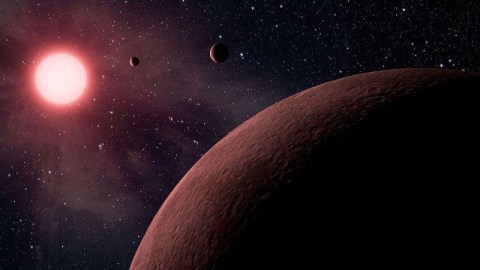
We see matter, but not antimatter, in our Universe. Could black holes be the answer?
One of the greatest cosmic puzzles in our entire Universe is why there’s so much more matter than antimatter. The laws of physics, as far as we can tell, only allow you to create or destroy matter and antimatter in equal amounts. Yet when we look out at the stars, galaxies, and large-scale structure of the Universe, we find that it’s all made of matter, with only trace amounts of antimatter to be found anywhere. This is a cosmic mystery, and has led many to speculate that perhaps there was an equal amount of antimatter somewhere that simply got segregated from the matter. Is this possible, and could black holes be that place? Anne Blankert wants to know, as she asks:
It is a mystery why we see matter without corresponding antimatter. Some remote and old super massive black holes evolved much faster than current theory is able to predict. Could the missing antimatter be hiding inside those primordial black holes? Does the total mass of super massive black holes come even close to the amount of missing anti matter?
It’s a fascinating thought. Let’s look deeply to try and figure it out.

Everywhere we look in the Universe, we see the same story: galaxies and stars, in all directions and all locations in space, at least on average. Sure, on small scales, galaxies clump and group together, but if you look on very large scales, the Universe has the same average properties (like density) everywhere. If, at any point, there were a galaxy made out of antimatter instead of matter, we would see a huge amount of matter/antimatter annihilation signatures and a dearth of matter in the intergalactic medium at the matter/antimatter interface. The fact that we don’t see this annihilation signature anywhere, not in individual galaxies, in clusters of galaxies, or in colliding clusters of galaxies, tells us that 99.999%+ of the Universe is definitely matter, like us, and not its antimatter counterpart.
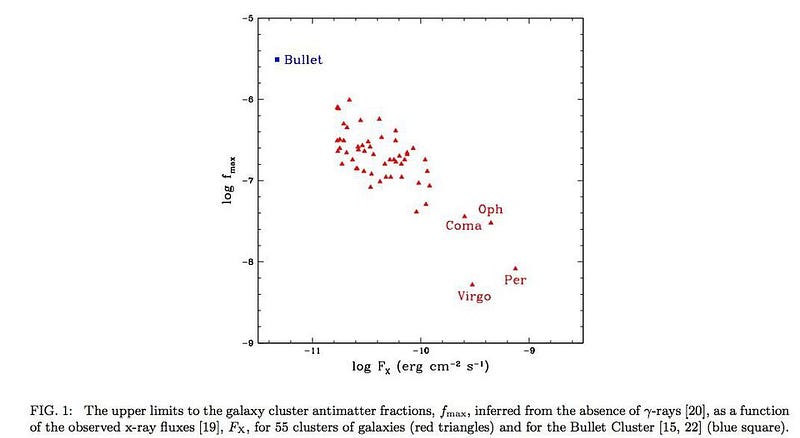
This is confusing, because we don’t know of any mechanisms, under the current laws of physics, that create more matter than antimatter. The symmetry between matter and antimatter, in terms of particle physics, is even more explicit than you might think. For example:
- every time we create a quark, we also create an antiquark,
- every time a quark is destroyed, an antiquark is also destroyed,
- every time we create-or-destroy a lepton, we also create-or-destroy an antilepton from the same lepton family, and
- every time a quark-or-lepton experiences an interaction, collision or decay, the total net number of quarks and leptons at the end of the reaction (quarks minus antiquarks, leptons minus antileptons) is the same at the end as it was at the beginning.
The only way we’ve ever made more (or less) matter in the Universe has been to also make more (or less) antimatter in an equal amount.
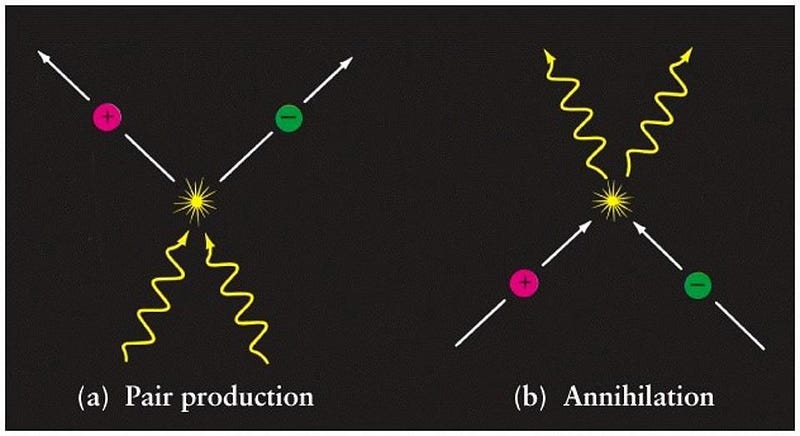
The standard interpretation of these facts is that, even though we aren’t entirely sure how, we must have created more matter than antimatter in the Universe’s past. In the standard picture of the hot Big Bang, when the Universe was in its very early stages, particle-antiparticle pairs of all the known (and even any yet-to-be-discovered) particles were created in tremendous abundance. This is because, at high temperatures and densities, you can spontaneously produce new particle-antiparticle pairs from pure energy, via Einstein’s E = mc². In equal amounts, these pairs annihilate, producing pure energy (photons) again. As the Universe cools, you run out of energy to make new pairs, and the annihilation dominates.
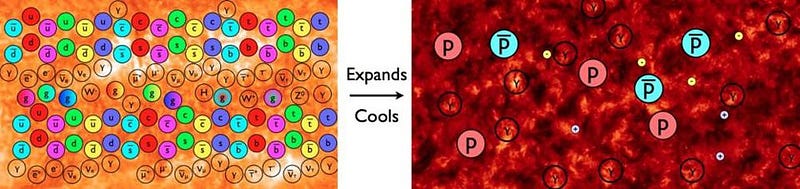
If we didn’t have any matter/antimatter asymmetry, we would have wound up with a Universe that has an incredible 10²⁰ photons for every proton, and one antiproton for every proton as well. There would be approximately the same number of electrons and positrons as there are protons and antiprotons, and that would be it. Instead, however, what we see is a Universe with “only” about 1-or-2 billion photons for every proton. Conventionally, we assume that there was some asymmetric process that happened in the early Universe to give rise to this asymmetry. A simple example would be the creation of a new set of particles and antiparticles with different preferences for decay channels from one another, which could lead to a Universe with a slight preference for matter over antimatter.
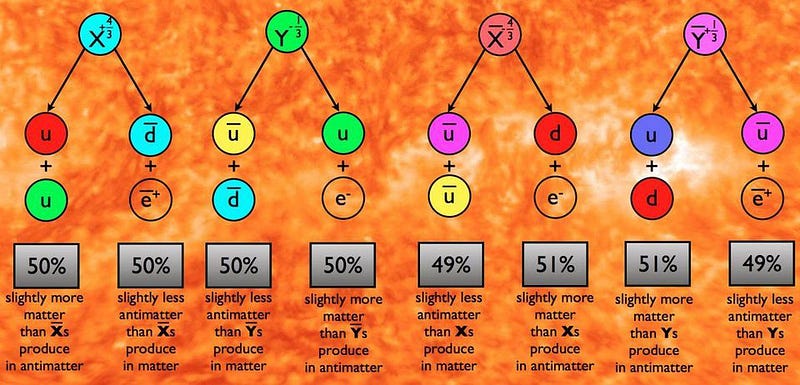
But what about this new idea? What if, at some early point, something compelled the antimatter to collapse into black holes, while leaving the normal matter behind? After all, we do see very early, abundant, supermassive black holes! Making them, however, isn’t necessarily a problem, or a good motivator for such a wild idea. Whatever can be explained without invoking any new physics should be, and for supermassive black holes, we think we can do that with the idea of direct collapse. Some black holes don’t need stars to burn and go supernova; they simply collapse, which could provide seeds large enough, fast enough, to grow into the young quasars we see today.
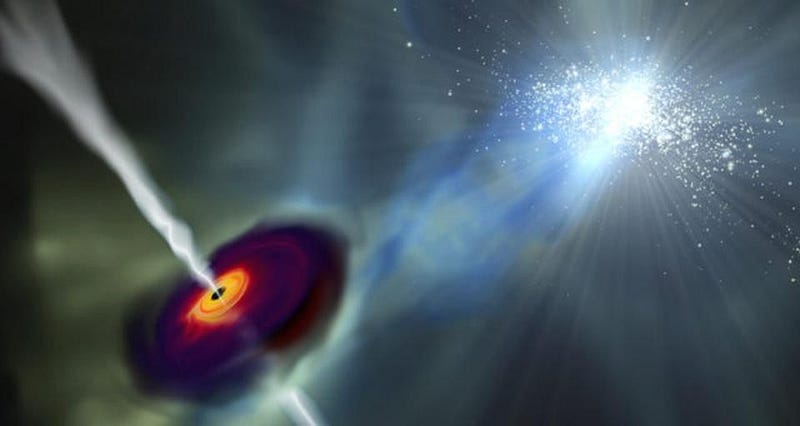
So don’t look to supermassive black holes. There’s also the idea of primordial black holes, which are revived periodically as a candidate for dark matter. They can’t be too light, or they would have decayed; they can’t be too heavy, or they would have been seen. Most of the possible mass ranges where primordial black holes could be the missing matter in the Universe are already ruled out or tightly constrained. To make a primordial black hole, you need a density fluctuation (a departure from the average density) that’s about 68% denser than average, but in the young Universe, the largest fluctuation was only about 0.006% denser than average. In fact, the only allowable mass range where primordial black holes could make up a significant fraction of the dark matter is already ruled out by LIGO , where the observed merger rate tells us that the total mass in these 10-to-100 solar mass ranges of black holes is less than about 0.000017% of the critical density.
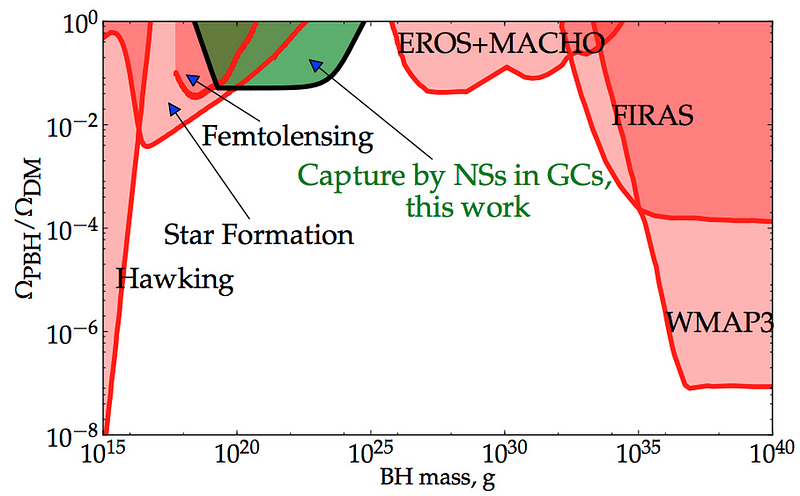
Going even further, we’ve managed to make estimates of the total mass of black holes in the Universe, and it comes out to about 0.007% of the total energy in the Universe. Given that there is about 700 times as much normal matter as there are black holes, this can’t be where the antimatter is hiding; antimatter didn’t form black holes.
But we had another way of knowing that: the laws of physics have symmetries between the way matter and antimatter are allowed to behave. One of those symmetries extends to the forces and interactions they experience, meaning that whatever forces matter particles experience, there must be the same magnitude forces (they can have the opposite sign) acting on antimatter. But this works both ways, and there can’t be any additional forces that only hit antimatter. If you want something to affect the antimatter in the Universe, it must also affect matter.
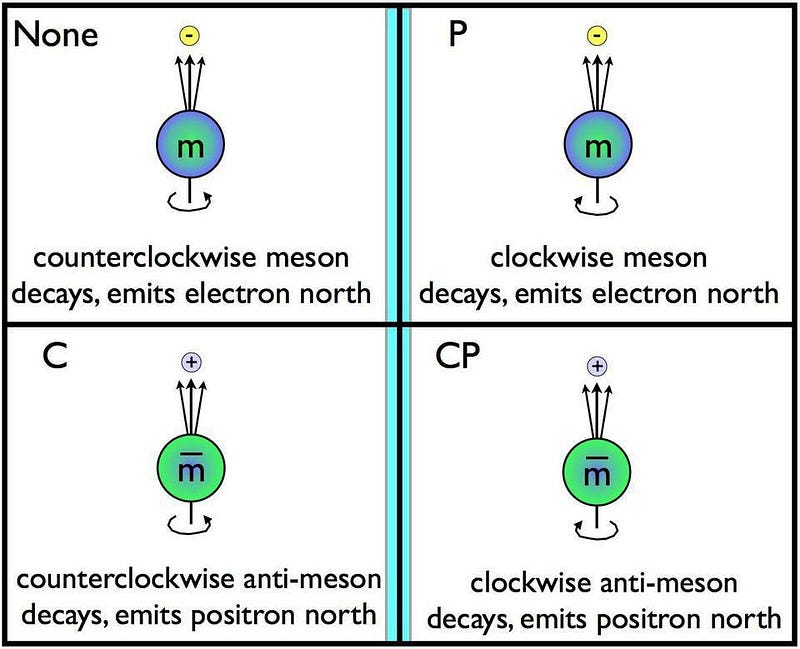
That’s why, given the laws of physics that we have, we’re certain that the antimatter couldn’t have all collapsed to black holes, leaving the normal matter behind. If there were equal amounts of dark matter and normal matter, that might be a compelling line-of-thought, but the combined facts that:
- we don’t need exotic physics to make the supermassive black holes in the early Universe,
- primordial black holes are ill-motivated (from structure formation) and largely ruled out as existing in any great abundance,
- and antimatter is forbidden from having interactions that would cause it to make black holes while having matter not make black holes,
is enough to lead us back to the standard picture. Somehow, the Universe made more matter than antimatter at some point in the very distant past, and that’s why we were able to come into existence in the first place. How, exactly, that happened remains one of the greatest unsolved problems in physics today.
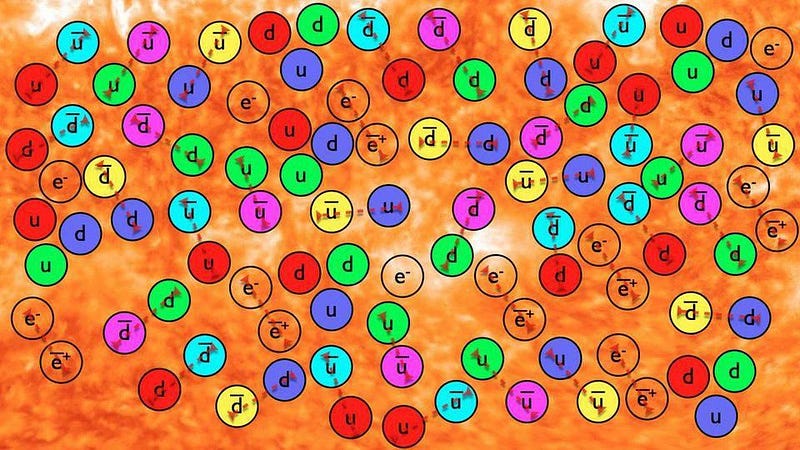
Send in your Ask Ethan questions to startswithabang at gmail dot com!
Ethan Siegel is the author of Beyond the Galaxy and Treknology. You can pre-order his third book, currently in development: the Encyclopaedia Cosmologica.