Ask Ethan: Why can’t black holes be made of dark matter?
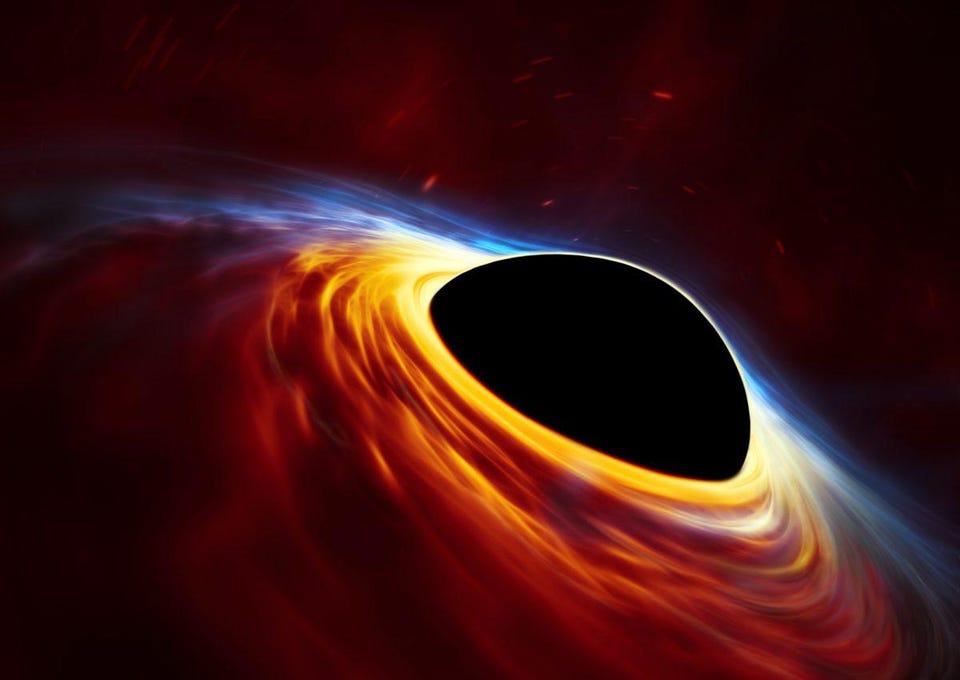
- If enough mass gathers together in one small volume of space, a black hole will inevitably form.
- Five-sixths of the mass in the Universe is made of dark matter, and just one-sixth is normal matter.
- And yet, we’re quite certain that all black holes in the Universe formed from normal matter, not dark matter. Here’s why.
In many ways, the most extreme objects in all the Universe are black holes. They pack more mass into a smaller volume of space than anything else, curving the fabric of space so severely that once anything enters a certain region, it can never escape, not even if it travels at the ultimate cosmic speed limit: the speed of light. There are likely millions of black holes distributed throughout each Milky Way-like galaxy in the Universe, with the most massive black holes reaching up into the millions or even billions of solar masses.
And yet, if we think about how all of these black holes formed, we’re quite certain that every single one was originally made from normal matter, and that none formed from dark matter, even though dark matter out-masses normal matter by a 5:1 ratio in the Universe. If they both gravitate equally, why is that? That’s the inquiry of N. D. Moller, who wants to know:
“If black holes are a result of the gravitational force, and if dark matter [also] responds to the gravitational force, what prevents the formation of a black hole [out of] dark matter?”
It’s a fantastic question and, thankfully, one we can answer. Here’s the cosmic story of why black holes can’t be made of dark matter.
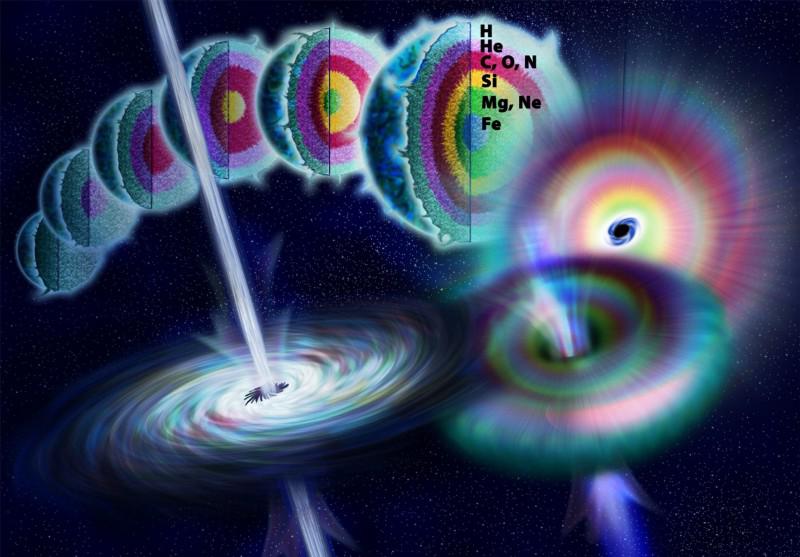
Credit: Nicolle Rager Fuller/NSF
In our modern Universe, there are only a few known ways to successfully make a black hole. Perhaps the most common method is from the core-collapse of an extremely massive star. The only thing holding stars up against gravitational collapse is the energy produced by nuclear fusion in their cores, where the outward radiation pressure balances the inward gravitational force. When the star’s core runs out of whatever fuel it’s burning, the radiation pressure starts to drop and the star’s core starts to contract under its own gravity.
When a star’s core contracts, it heats up. If it gets hot enough, it can start burning even heavier elements, using them as fuel. Once it’s done fusing elements like silicon, however, there’s nowhere else to go, and the core collapses in a type II supernova event. If the star’s core is massive enough, a black hole will form (instead of a neutron star) by virtue of collecting so much mass in such a tiny volume of space.
You can also form a black hole from the collision of two neutron stars, as long as the combined mass of the post-merger object is again greater than a certain threshold.
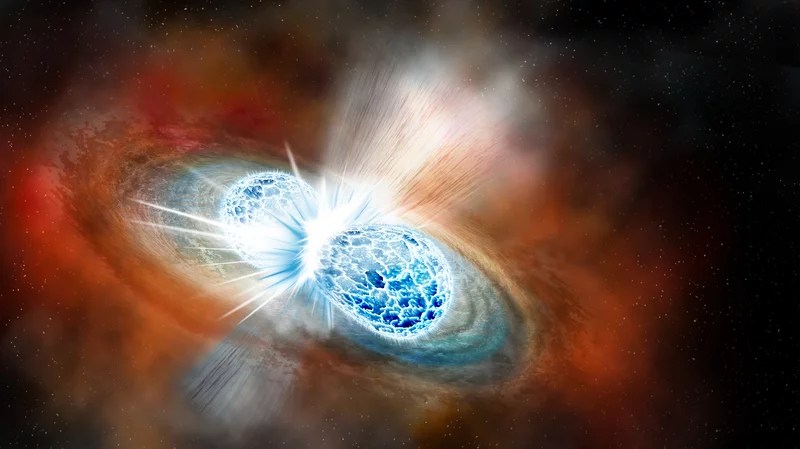
Credit: Robin Dienel/Carnegie Institution for Science
Finally, there’s also the opportunity to form a black hole from direct collapse, where either a contracting cloud of gas or a single, massive star simply gets too dense for light to escape from within a certain volume of space. Once you meet that condition, an event horizon forms, and anything that crosses it is guaranteed not only to never escape, but will swiftly grow the mass — and hence, the size — of your newly forming black hole. In short order, it can swallow the entire mass of whatever object it formed from, and then you’ve got a black hole on your hands.
To recap the three main, known ways to produce a black hole where there was none previously:
- from a core-collapse supernova
- from the collision and merger of two neutron stars
- from a direct collapse process
Although there might be other exotic ways to produce a black hole, these are the ones thought to be responsible for the overwhelming majority of black holes in our Universe.

Credit: NASA/ESA/C. Kochanek (OSU)
As you’ve likely noticed, all of these rely on normal matter: matter that’s made out of atoms and their constituent components. This might be a bit of a puzzle to you, when you consider the following facts.
- Normal matter makes up just one-sixth of the total mass in the Universe, with dark matter constituting the remaining five-sixths.
- Normal matter and dark matter both experience the gravitational force equally, obeying Newton’s and Einstein’s laws of gravitation in precisely the same fashion.
- In every environment where there’s a copious amount of normal matter, such as a galaxy like the Milky Way, there’s significantly more dark matter overall, with ratios of 5:1 in favor of dark matter, at minimum.
Why, then, would normal matter be so effective at forming black holes, while dark matter wouldn’t be? The key lies not in the gravitational force, but in the other dissipative forces: things like friction and collisions, which rely on the electromagnetic interaction. Normal matter experiences electromagnetic interactions. Dark matter doesn’t. Astrophysically, this makes a tremendous difference in what happens to each one.
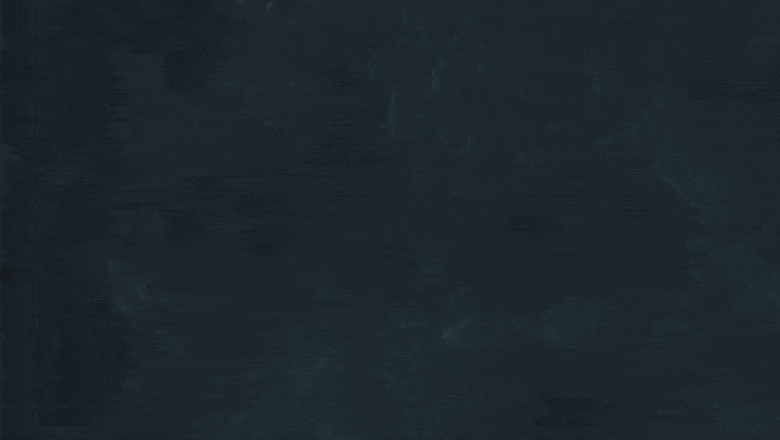
Credit: Ralf Kaehler and Tom Abel (KIPAC)/Oliver Hahn
When a galaxy forms, dark matter and normal matter both typically flow into it in the same cosmic amounts: that 5:1 ratio that exists on average throughout the cosmos. Just like all particles under the influence of gravity, they’re attracted to the center of the gravitational potential, and that’s the direction in which they accelerate.
However, that’s where the similarities end. When dark matter takes the plunge through the existing galaxy, it has no collisions, no friction, experiences no heating, no interactions with electromagnetic radiation, and no way to exchange energy or momentum with the other particles — both normal and dark particles — that exist within the galaxy. It begins, and will remain, on a slow, high-angular-momentum orbit, taking perhaps a billion years or so to make a single, full ellipse.
Normal matter, on the other hand, has all of those things that dark matter lacks. It collides with other normal matter particles, which can exchange energy and momentum. Particles can stick together, resulting in a dissipative loss of kinetic energy and angular momentum. They experience kinetic and thermal friction, heating up when they interact with other normal matter particles. And they respond to radiation, possessing a significant cross-section.
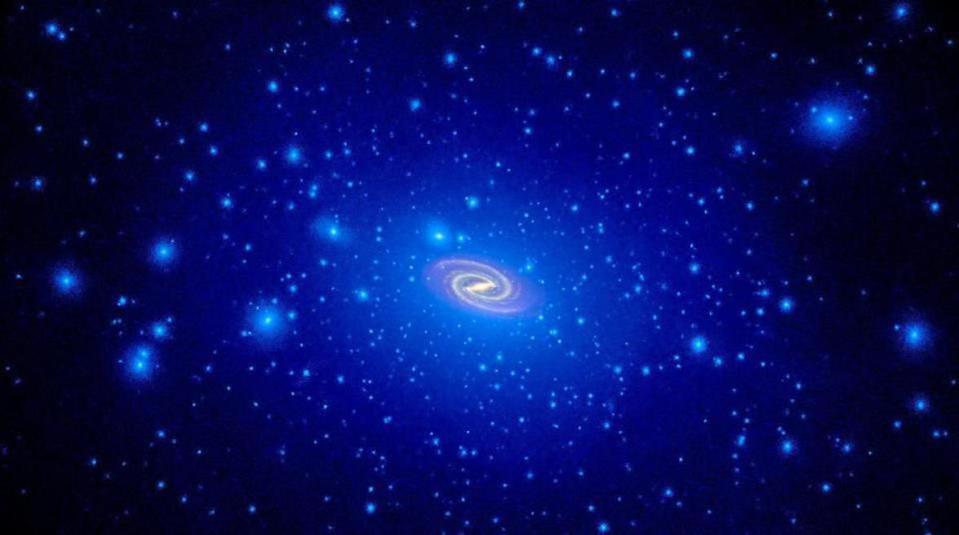
Credit: NASA, ESA, and T. Brown and J. Tumlinson (STScI)
Together, all of these phenomena result in a major difference that can be summed up as follows: normal matter sheds momentum and angular momentum and sinks to the core of the galaxy, where it clumps together, while dark matter always remains diffusely distributed in an enormous halo around the galaxy, unable to shed linear or angular momentum. Normal matter forms individual, dense, small-scale clumps. Dark matter can only form sparse, diffuse clumps, mostly on very large scales.
Without a mechanism by which it can shed angular momentum, dark matter can never even approach the densities necessary to create an event horizon and hence, a black hole. There is no such thing as a dark matter star, so you can’t have dark matter undergo core collapse. There are no regions that ever collect enough dark matter to pull light within that region back on itself, meaning that you can’t have dark matter undergo direct collapse. And there are no stellar remnants or other comparably dense objects made out of dark matter, meaning that there’s no way to have a collision between dark matter-rich entities that lead to a black hole. All the mechanisms by which normal matter can wind up creating a black hole fail when applied to dark matter.
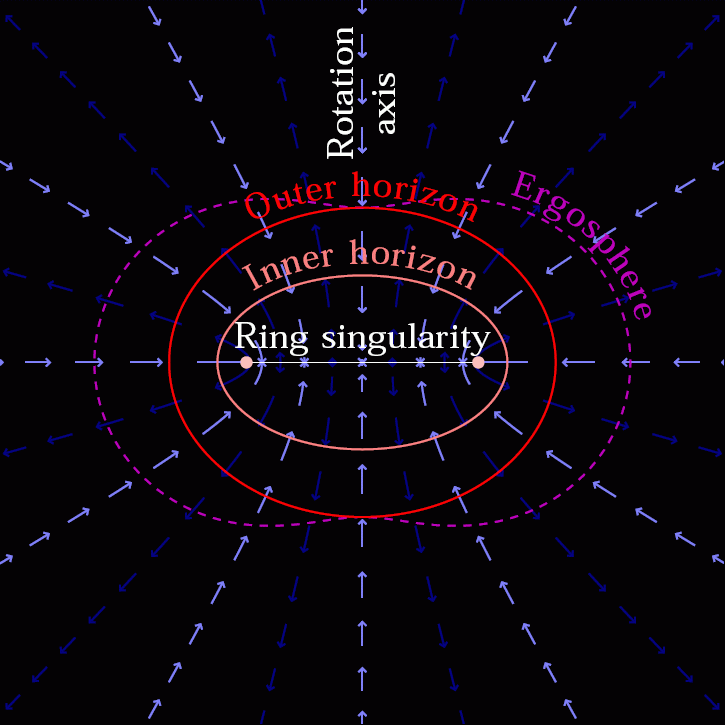
Credit: Andrew Hamilton/JILA/University of Colorado
Now, once you make a black hole from normal matter, there’s no reason dark matter particles can’t cross over the event horizon and add to the mass of the already-existing black hole. Dark matter is no different than any other form of matter or radiation in this regard: if you cross over the edge of the event horizon, you will inevitably fall to the central singularity and wind up adding to the overall mass of the black hole. But because of the clumpy nature of normal matter and the diffuse nature of dark matter, there are no realistic scenarios in which even 1% of a black hole’s total mass could come from dark matter. For black holes, it’s normal matter or nothing.
In fact, based on what we understand about dark matter and the Universe, there’s only one possibility for ever creating a black hole made of dark matter. That will occur only if the very early Universe is born with a large-enough-magnitude fluctuation that — rather than either getting washed out by outflowing radiation or slowly growing as the seeds of large-scale structure — it will itself collapse in rapid fashion, leading to the formation of a black hole well prior to the formation of any stars at all. If this occurred, it could have created one or more primordial black holes: a set of black holes that existed irrespective of stars. This is one instance where it’s only the mass that matters, not whether it’s normal or dark.
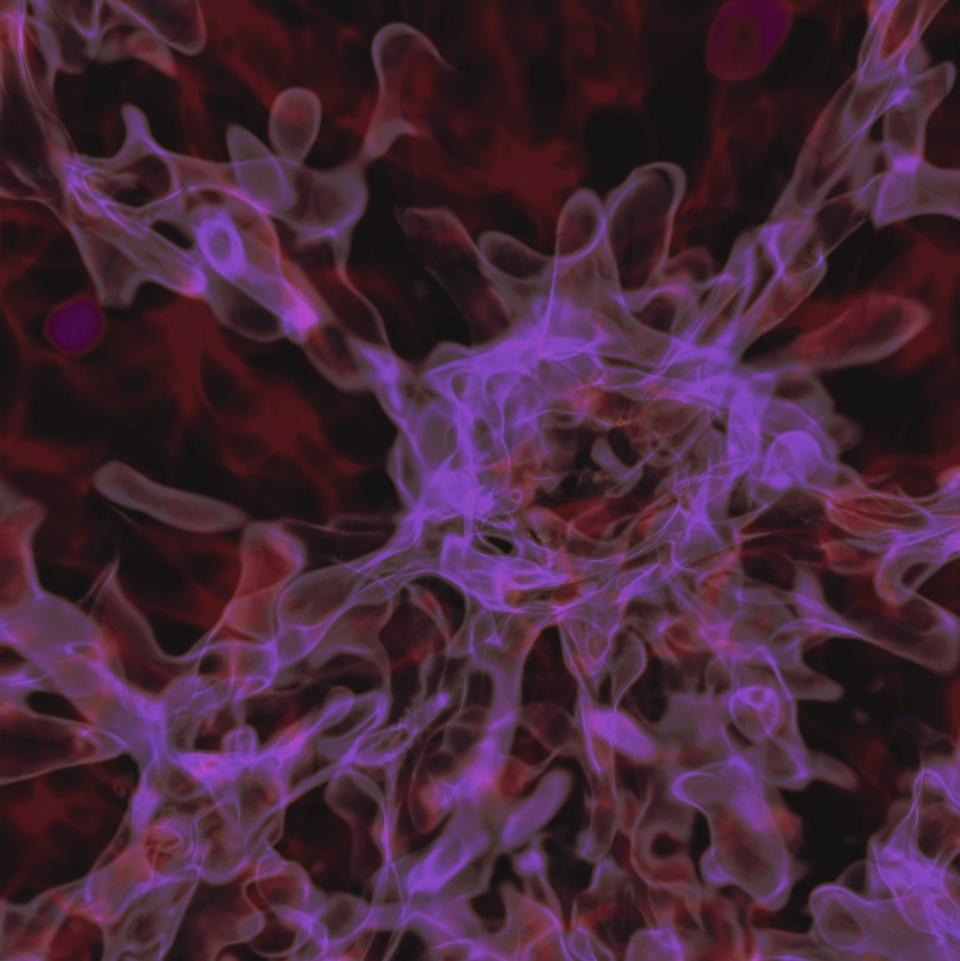
Because it’s solely based off of the physics of gravitation and the way that structure forms in an expanding Universe, it’s a straightforward calculation to determine how large of an overdensity you need to result in a primordial black hole. No matter the average density, if a Universe is born with a region that’s locally 68% greater than that average density, it will lead to gravitational collapse and the formation of a primordial black hole. This is irrespective of mass or size, and only depends on the magnitude of the overdensity.
Now, we have a Universe that was born with a spectrum of fluctuations, and those seed fluctuations gave rise to the structures we see everywhere in the Universe. We see the effects of these fluctuations in:
- the temperature imperfections in the cosmic microwave background
- polarization signatures in the cosmic microwave background
- features like correlation functions and the power spectrum of the large-scale structure of the Universe
With these in mind, we can reconstruct what sort of fluctuations the Universe must have been born with.
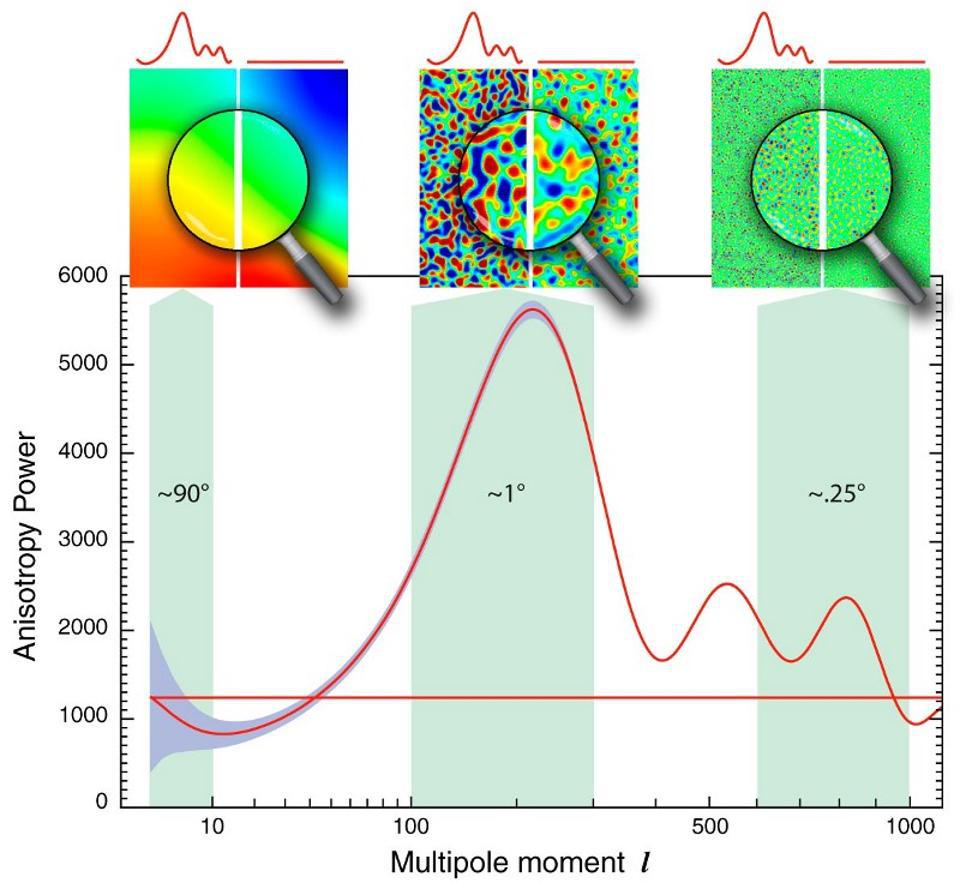
Credit: NASA/WMAP science team
What we find when we observe the Universe, consistent with the predictions of cosmic inflation, is one that must have been born with slightly larger fluctuations (by about 3%) on the largest cosmic scales than the smallest measurable cosmic scales, and whose fluctuations, in magnitude, are about 0.003% of the average value. In other words, if we look for a very rare fluctuation — a 5-σ fluctuation, which occurs for about 1 in every 3.5 million such fluctuations — it will correspond to a region that’s only 0.015% greater or lower than the average density.
That’s an enormous gap: from 0.015% to 68%, and the only way to achieve it would be to invoke some sort of new, novel physics on a specific, small scale. This new physics must have successfully hid, so far, all evidence of its existence, and would need to be invoked solely to create a population of dark matter-based primordial black holes: a population for which there is no evidence. In fact, when we look at the actual evidence, we have only constraints (in many cases, quite good constraints) on the possible abundances of primordial black holes, and what fraction of the dark matter they could possibly be. Although we can’t exactly rule out that these objects exist below the currently observed threshold, there’s no physical reason or evidence to assume that such entities exist.
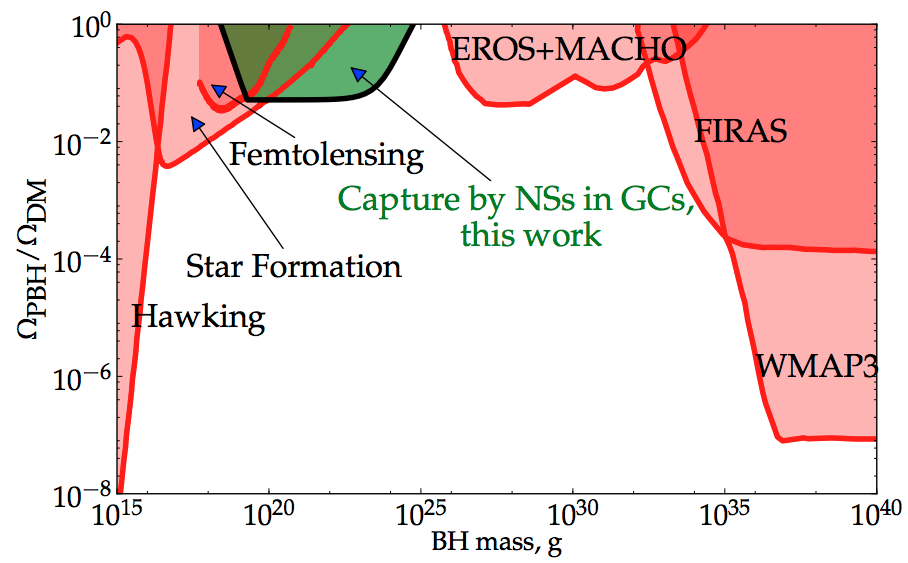
When it comes to drawing scientific conclusions, it’s important to ask the right questions, and not to be drawn in by the allure of wishful thinking. Rather than ask, “can I concoct a scenario that evades current constraints?” which gives you, at best, an option for “can I believe that this might be true?” we should be looking at the evidence and asking, with minimal additional assumptions, “what does the Universe tell us about itself that we can conclude actually is true?”
That key question — what is true? — is at the heart of all matters scientific. If there’s no evidence in favor of a hypothesis’s truth, it is scientifically irresponsible to conclude that the hypothesis itself is true. When it comes to dark matter as we understand it, we can fully expect that there will be no black holes in the Universe, today or ever, made either primarily or exclusively of dark matter. There are no stars, supernovae, stellar remnants, or direct collapse scenarios that should have led to a black hole made of dark matter, and no way for dark matter to be dense enough to form a black hole once the Universe has gotten underway.
Unless we invoke new, yet unobserved new physics to force the creation of a particular spectrum of primordial black holes, all made from dark matter, we can safely say that it’s the normal matter, not the dark matter, that’s responsible for the black holes we observe within our Universe.
Send in your Ask Ethan questions to startswithabang at gmail dot com!