Black Holes Must Have Singularities, Says Einstein’s Relativity
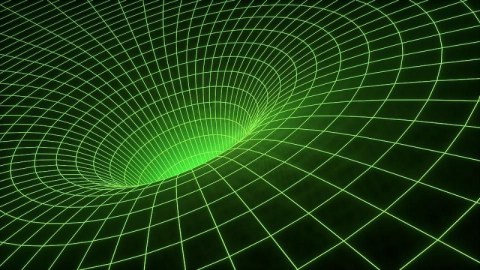
Unless you can make a force that travels faster than the speed of light, a singularity is inevitable.
The more mass you place into a small volume of space, the stronger the gravitational pull gets. According to Einstein’s general theory of relativity, there’s an astrophysical limit to how dense something can get and still remain a macroscopic, three-dimensional object. Exceed that critical value, and you’re destined to become a black hole: a region of space where gravitation is so strong that you create an event horizon, and a region from within which nothing can escape. No matter how fast you move, how quickly you accelerate, or even if you move at the ultimate speed limit of the Universe — the speed of light — you can’t get out. People have often wondered whether there might be a stable form of ultra-dense matter inside that event horizon that will hold up against gravitational collapse, and whether a singularity is truly inevitable. But if you apply the laws of physics as we know them today, you cannot avoid a singularity. Here’s the science behind why.
Imagine the densest object you can make that isn’t yet a black hole. When massive stars go supernova, they can make either a black hole (if they’re above a critical threshold), but more commonly will see their cores collapse to form a neutron star. A neutron star is basically an enormous atomic nucleus: a bound-together collection of neutrons more massive than the Sun, but contained in a region of space just a few kilometers across. It’s conceivable that if you exceed the allowed density at the core of a neutron star, it might move on to an even more concentrated state of matter: a quark-gluon plasma, where densities are so great that it no longer makes sense to consider the matter in there as individual, bound structures.
Why can we have matter at all, though, inside the core of such a dense object? Because something must be exerting an outward force, holding the center up against gravitational collapse. For a low-density object like Earth, the electromagnetic force is enough to do it. The atoms that we have are made of nuclei and electrons, and the electron shells push against each other. Because we have the quantum rule of the Pauli Exclusion Principle, which prevents any two identical fermions (like electrons) from occupying the same quantum state. This holds for matter as dense as a white dwarf star, where a stellar-mass object can exist in a volume no greater than the size of Earth.
If you place too much mass on a white dwarf star, however, the individual nuclei themselves will undergo a runaway fusion reaction; there’s a limit to how massive a white dwarf star can get. In a neutron star, there are no atoms at the core, but rather one enormous atomic nucleus, made almost exclusively of neutrons. Neutrons also act as fermions — despite being composite particles — and quantum forces also work to hold them up against gravitational collapse. It’s possible, beyond that, to imagine another, even denser state: a quark star, where individual quarks (and free gluons) interact with each other, obeying the rule that no two identical quantum particles can occupy the same quantum state.
But there’s a key realization in the mechanism that prevents matter from collapsing down to a singularity: forces must be exchanged. What this means, if you attempt to visualize it, is that force carrying particles (like photons, gluons, etc.) must be exchanged between the various fermions in the interior of the object.
The thing is, there’s a speed limit to how fast these force-carriers can go: the speed of light. If you want an interaction to work by having an interior particle exert an outward force on an exterior particle, there needs to be some way for a particle to travel along that outward path. If the spacetime containing your particles is below the density threshold necessary to create a black hole, that’s no problem: moving at the speed of light will allow you to take that outward trajectory.
But what if your spacetime crosses that threshold? What if you create an event horizon, and have a region of space where gravity is so intense that even if you moved at the speed of light, you couldn’t escape?
All of a sudden, there’s no path at all that will work! The gravitational force will work to pull that exterior particle inwards, but under these conditions the force-carrying particle coming from the interior particle simply cannot move outwards. Inside a dense-enough region, even massless particles have nowhere to go except towards the most interior points possible; they cannot influence exterior points. So the exterior particles have no choice but to fall in, closer to the central region. No matter how you set it up, every single particle inside the event horizon inevitably winds up at a singular location: the singularity at the black hole’s center.
So long as particles — including force-carrying particles — are limited by the speed of light, there’s no way to have a stable, non-singular structure inside a black hole. If you can invent a tachyonic force, which is to say a force mediated by particles that move faster-than-light, you might be able to create one, but so far no real, tachyon-like particles have been shown to physically exist. Without that, the best you can do is “smear out” your singularity into a one-dimensional, ring-like object (due to angular momentum), but that still won’t get you a three-dimensional structure. So long as your particles are either massive or massless, and obey the rules of physics we know, a singularity is an inevitability. There can be no real particles, structures, or composite entities that survive a journey into a black hole. Within seconds, all you cam ever have is a singularity.
Ethan Siegel is the author of Beyond the Galaxy and Treknology. You can pre-order his third book, currently in development: the Encyclopaedia Cosmologica.