Black holes are common. So where are the white holes?
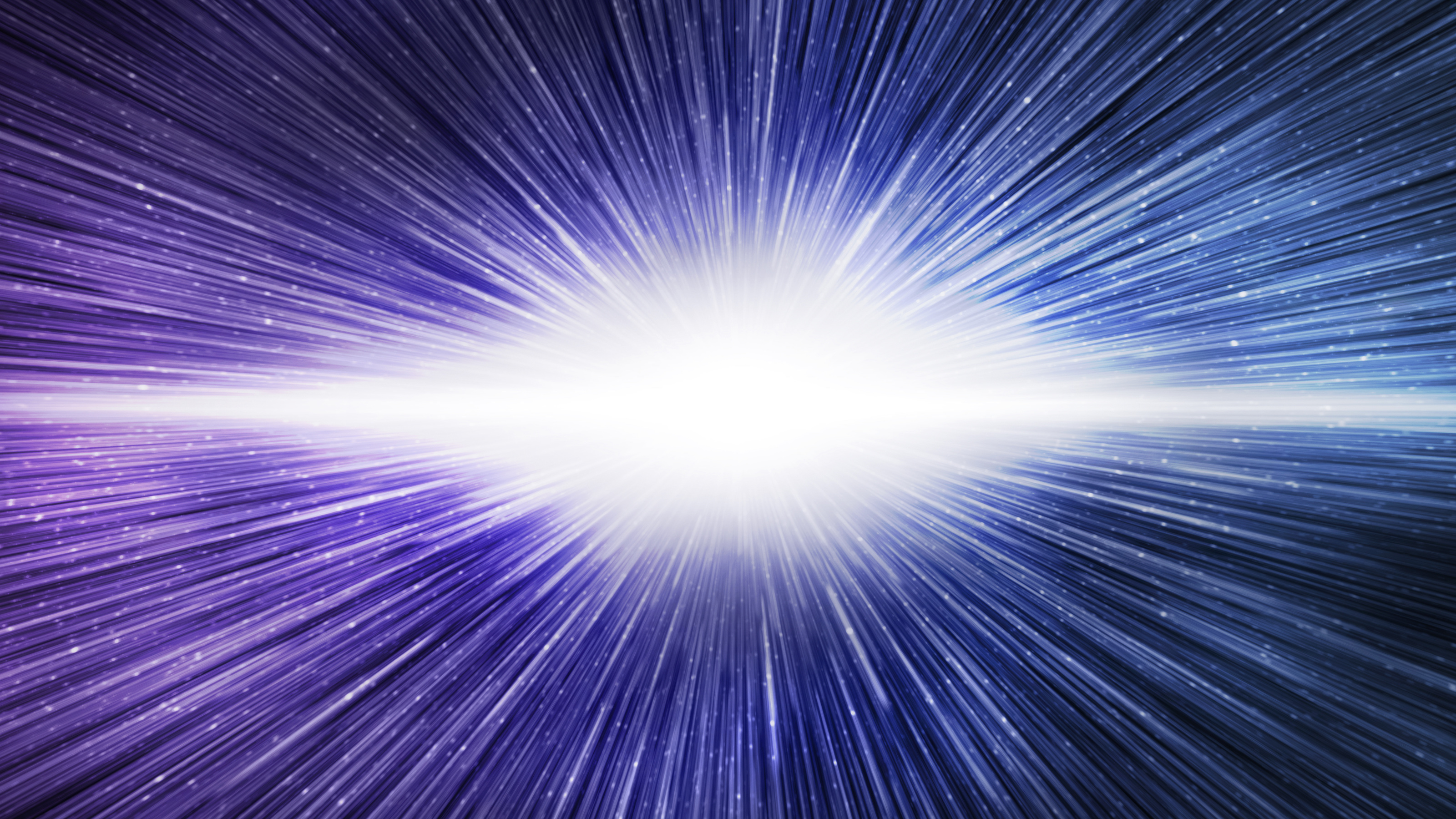
- Many of the laws of physics, including all of general relativity, are symmetric in both time and space: it doesn’t matter whether your clocks run forward or backward.
- We know that black holes, mathematically predicted to exist by general relativity, are absolutely real, and we’ve even detected and measured a large number of them directly.
- Could white holes, the time-reversed equivalent of a black hole, be just as real? And what would that mean for our Universe if so?
In our Universe, the laws of physics tell us all the possibilities for what’s allowed to conceivably exist, but only by actually observing, measuring, and experimenting with our Universe itself can we determine what’s truly real. In Einstein’s general relativity, one of the very first possibilities that was ever discovered was for a black hole: a region of space with so much matter-and-energy in one place that from within that volume, nothing, not even light, could ever escape. The flip side of that is an equally possible mathematical solution that’s the reverse of a black hole: a white hole, from which matter and energy will spontaneously emerge.
Black holes have been demonstrated, through many different types of observations, to not only be physically real, but to be quite abundant all throughout the Universe. What about white holes? What are they, and are they physically real, too? That’s what Kristin Houser wants to know, as she asks:
“[I] came across a random blog post about white holes and wondered if you’d ever written about them? […] I bet anything you wrote would be far better than what’s showing up on page 1 of Google.”
It’s one of the most fascinating possibilities ever conceived of. Let’s take a deep look at all we know.
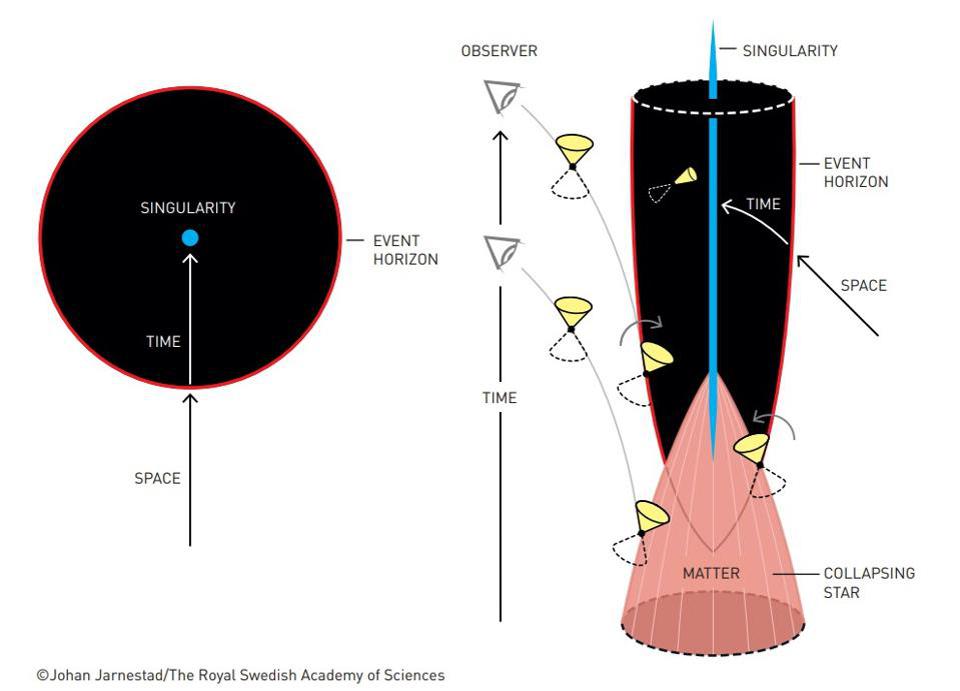
The idea of white holes makes a lot more sense if you begin with its much more familiar counterpart: the black hole. First thought up in the 18th century by John Michell who referred to them as “dark stars,” it was realized that just as all masses in the Universe have an “escape velocity” from their surface — i.e., there’s a certain speed that one must reach to escape completely from its gravitational pull — that if enough mass is gathered in a small enough volume, that escape velocity would reach or exceed the speed of light. Since nothing can move faster than that speed, these objects would only absorb light-and-matter, but would never emit any from within a certain distance: its event horizon.
The original idea was put forth within the context of Newtonian gravity, but in 1915, Einstein’s general theory of relativity was released, superseding Newton’s and replacing it with a more comprehensive law of gravity. Nevertheless, black holes persisted: they were shown to arise within Einstein’s theory as early as 1916, and versions of black holes with electric charges and angular momentum (i.e., spin) as well as mass were also discovered. Once again, with enough mass in one region of space, the creation of a black hole would be all but inevitable.
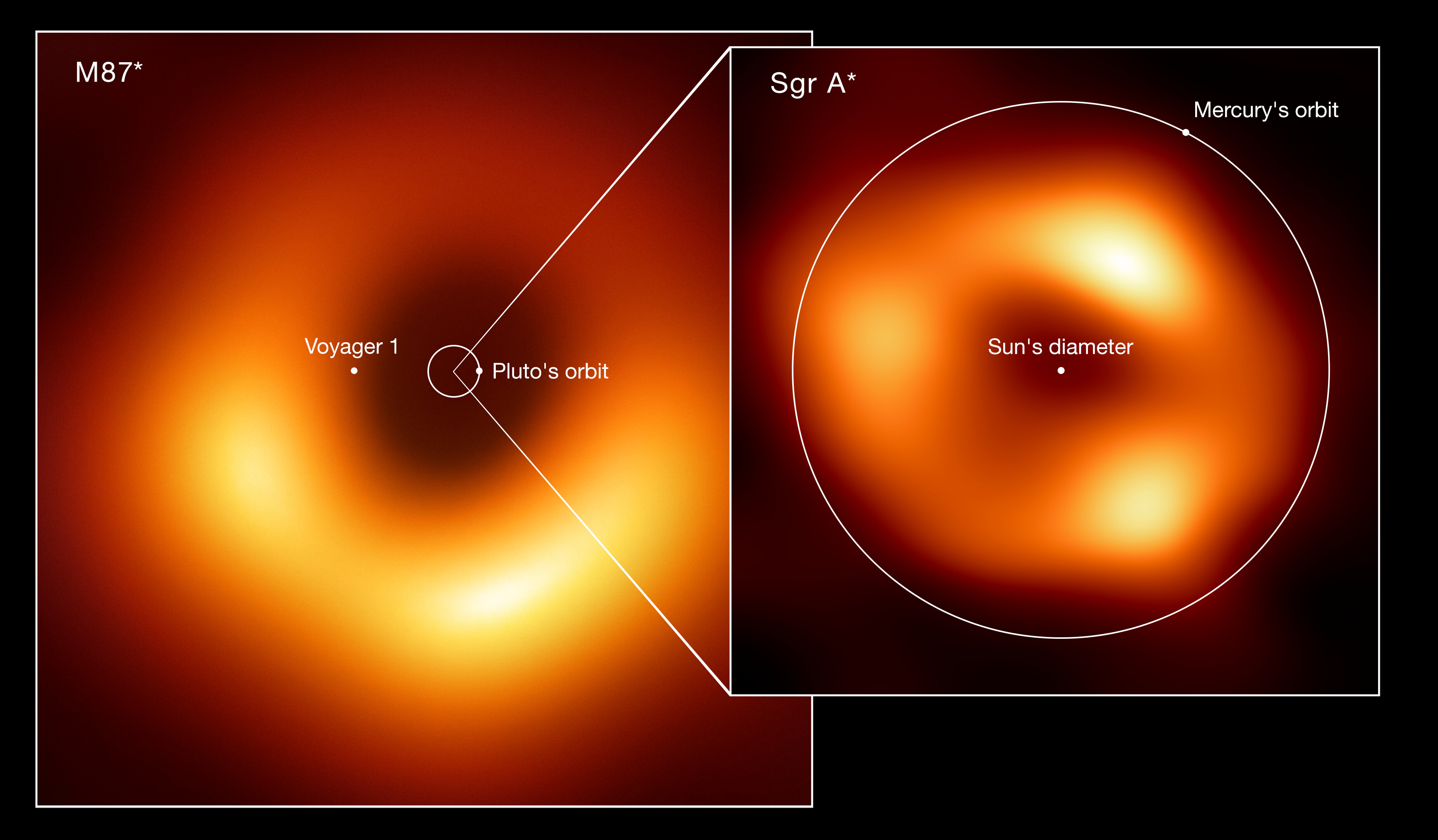
One of the fascinating things that must happen inside of a black hole’s event horizon, according to the laws of relativity, is the formation of a singularity. A singularity — sometimes jokingly referred to as a place where “God divided by zero” — is where the laws of physics break down. In the case of a black hole, it’s where the rules that describe space and time are no longer applicable; it’s as though at that location, you get nothing but nonsense for answers to any physical question you can ask of the system.
Regardless of what configuration of initial matter and energy you had prior to the formation of a black hole, once that material collapses and forms an event horizon, the creation of a singularity cannot be avoided. If you only have mass to your black hole, that singularity will be a point, surrounded by a spherical event horizon. If your black hole also has angular momentum (i.e., if it spins), then that singularity gets smeared out into a one-dimensional ring: and still, the laws of physics break down everywhere along that ring, again giving nonsense answers for any questions involving time or space.
Even though they don’t emit any light themselves, however, their effects on matter — from binary companion stars to infalling gas and material to photons that get bent and distorted by the black hole’s gravity — have revealed their presence for many decades, culminating a few years ago with the direct imaging of the light bent around a black hole’s event horizon itself.

So if that’s a black hole, then what is a white hole?
There are two ways to look at it. One is to simply recognize that general relativity is a time-symmetric theory: if you observe a system of matter-and-energy in motion through the fabric of space over time, you cannot tell whether the clock is running forward or backward. general relativity’s predictions are symmetric in time, meaning that objects move, accelerate, and interact according to the same laws in both cases.
This applies to even bizarre cases. Two black holes, orbiting one another in a decaying fashion and emitting gravitational waves, obey the same physical rules as two black holes that orbit one another and absorb gravitational waves from their surroundings, getting farther and farther apart over time. A cloud of contracting matter that fragments into clumps that will eventually form stars obeys the same rules as a series of expanding clumps of matter that move apart from their points-of-origin and diffuse into a large, fluffy cloud.
And matter that collapses to form an event horizon and then a singularity, i.e., a black hole, obeys exactly the same rules as a singularity from which matter-and-energy, as well as space-and-time, emerge. Considering the case of a time-reversed black hole is one powerful way to conceive of a white hole.
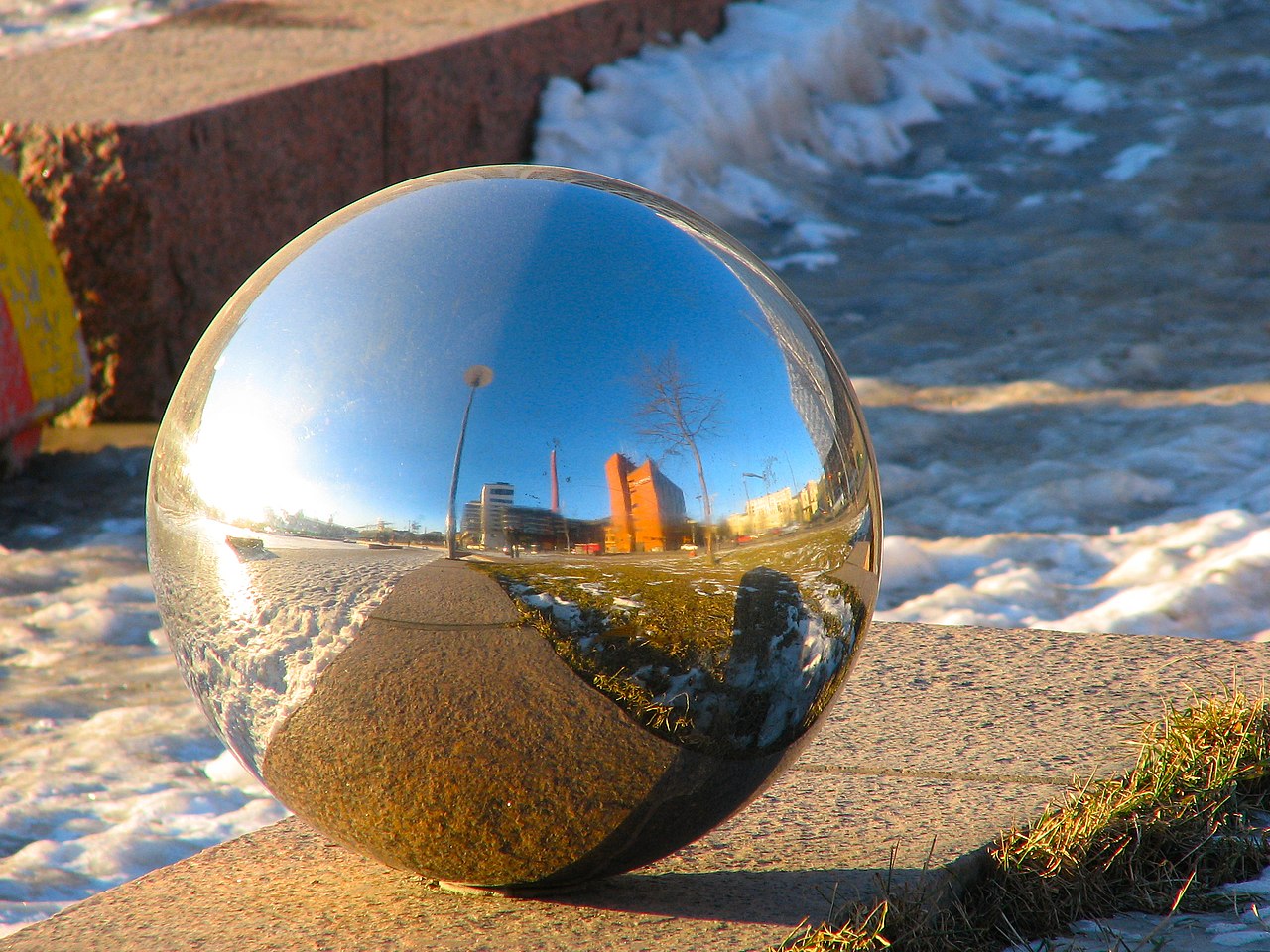
Another way to think about a white hole isn’t to reverse the arrow of time, but rather to think about what happens if you treat space as reversible. Before you scratch your head wondering how such a thing is possible, be aware that we have an analogue of that in the real world: a spherical, mirrored orb. If you were to put a spherical mirror in space, you’d be able to see a reflection of the entire exterior Universe in it, simply by looking at the mirror from the right perspective.
Well, the spacetime inside and outside of a black hole’s event horizons behave very analogously to exactly that situation. If you consider a black hole that’s defined only by a point mass — i.e., a Schwarzschild black hole — then for whatever value of mass/energy the black hole has, we can also define a specific radius (we call it “R“) for the black hole’s event horizon.
You can ask all sorts of questions about “how space behaves” at any distance from that black hole, and we can call that distance “r” instead. There are now three cases:
- r > R, which places us outside the event horizon.
- r = R, which places us at the event horizon.
- And r < R, which places us inside the event horizon.
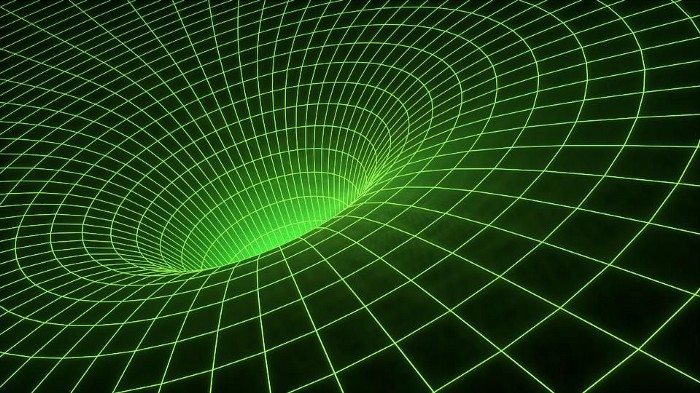
Now, here’s the tricky part: reversing space. All we have to do is replace r, everywhere we see it, with its inverse relative to the event horizon: ℛ, which we can define as ℛ = R²/r.
Remarkably, we now have those same three cases, but everything is reversed!
- ℛ > R, which places us inside the event horizon,
- ℛ = R, which places us at the event horizon,
- and ℛ < R, which places us outside the event horizon.
Despite the fact that this is now the opposite set of conditions for a black hole, the equations that describe space and time are identical for both cases.
What this means, then, is that if we pretend a black hole is “flipped” inside out — so that every point from the inside of a black hole’s event horizon (including its singularity at r = 0) now corresponds to a point outside of a black hole’s event horizon (where the singularity now goes everywhere at r = ∞), and vice versa — we recover the identical behavior. The only difference is what was outside is now inside and what was inside is now outside; it’s just reversed. Instead of a black hole, this “flipped” object can now be thought of as a white hole.
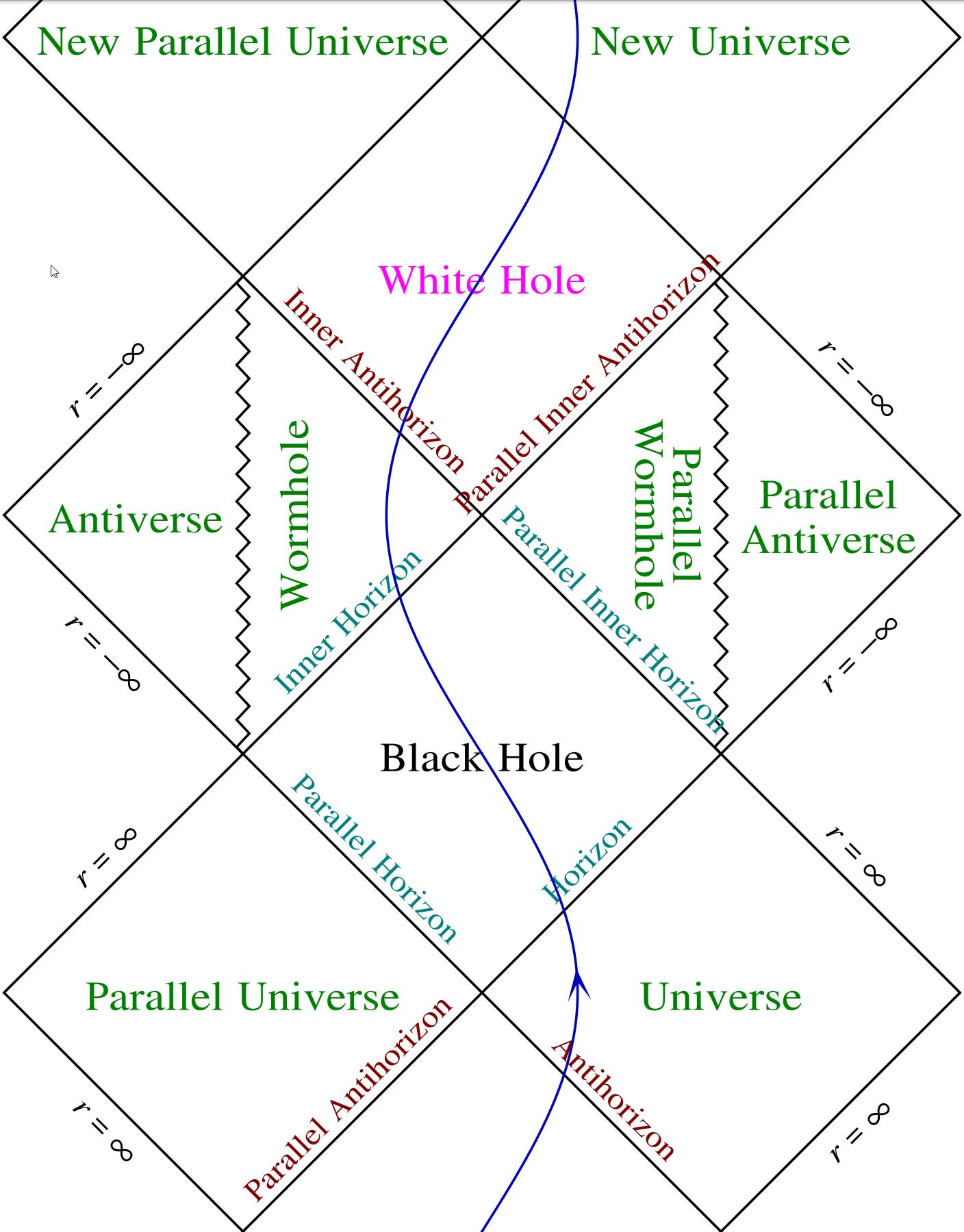
One of the questions physicists often wonder about is this: when something crosses over to the other side (i.e., the inside) of a black hole’s event horizon, where does it go? Sure, you can simply state, “It goes into the black hole’s central singularity,” but that’s a dissatisfying answer, especially because we know the laws of physics break down at that singularity.
One possibility that is often considered is that the singularity might not be just a point where things “go to” after falling into an event horizon, but might equally be a point where things “emerge from” as well. Rather than simply being “the end” of the story for matter, it could instead be “the beginning” of a new, different story.
In other words, it’s completely plausible that there will be events that correspond to large amounts of matter-and-energy emerging at a specific place-and-time that appear to correspond to a singularity as well. Not only might our Universe have black holes, but there could be white holes as well: places where things appear to begin from an initial singularity. It is not lost on physicists that, in many ways, that appears to correspond to a remarkable event that occurred 13.8 billion years ago: the hot Big Bang.
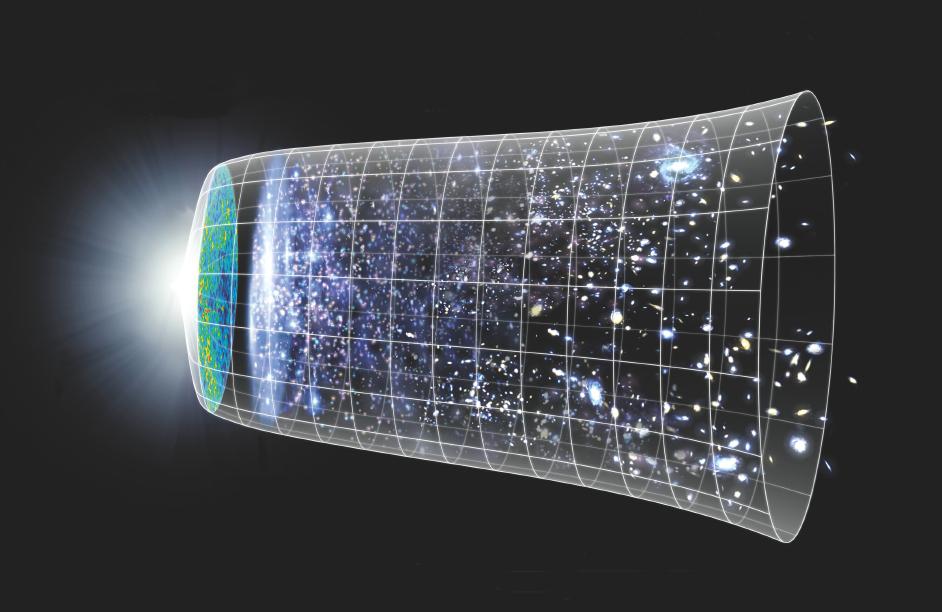
This brings up the fascinating possibility that there’s a connection between black holes and the emergence of a new Universe. Every time our Universe forms a new black hole, is there a baby Universe that emerges, analogous to a white hole, somewhere on the other side of a singularity?
Does this also imply that our Universe, and our own hot Big Bang, emerged from a state not so different from a white hole, and was that possibly caused by a prior Universe forming a black hole, from which our emergence was the aftermath?
There’s a fun calculation one can do with only a little effort that suggests this idea might be one to take seriously. If you were to add up all the matter and radiation within the observable Universe — all the atoms, all the black holes, all the dark matter, all the photons and all the neutrinos — you’d get a value for the effective “mass” of the observable Universe. (After all, if Einstein’s most famous equation tells us that E = mc², then it’s also true that m = E/c², so we can come up with a mass-equivalent value for all things that possess energy.) And if you were to then imagine that all of that mass went into creating a black hole, you could calculate what the expected radius for a black hole with an event horizon with a mass-equivalent of what’s inside our observable Universe.
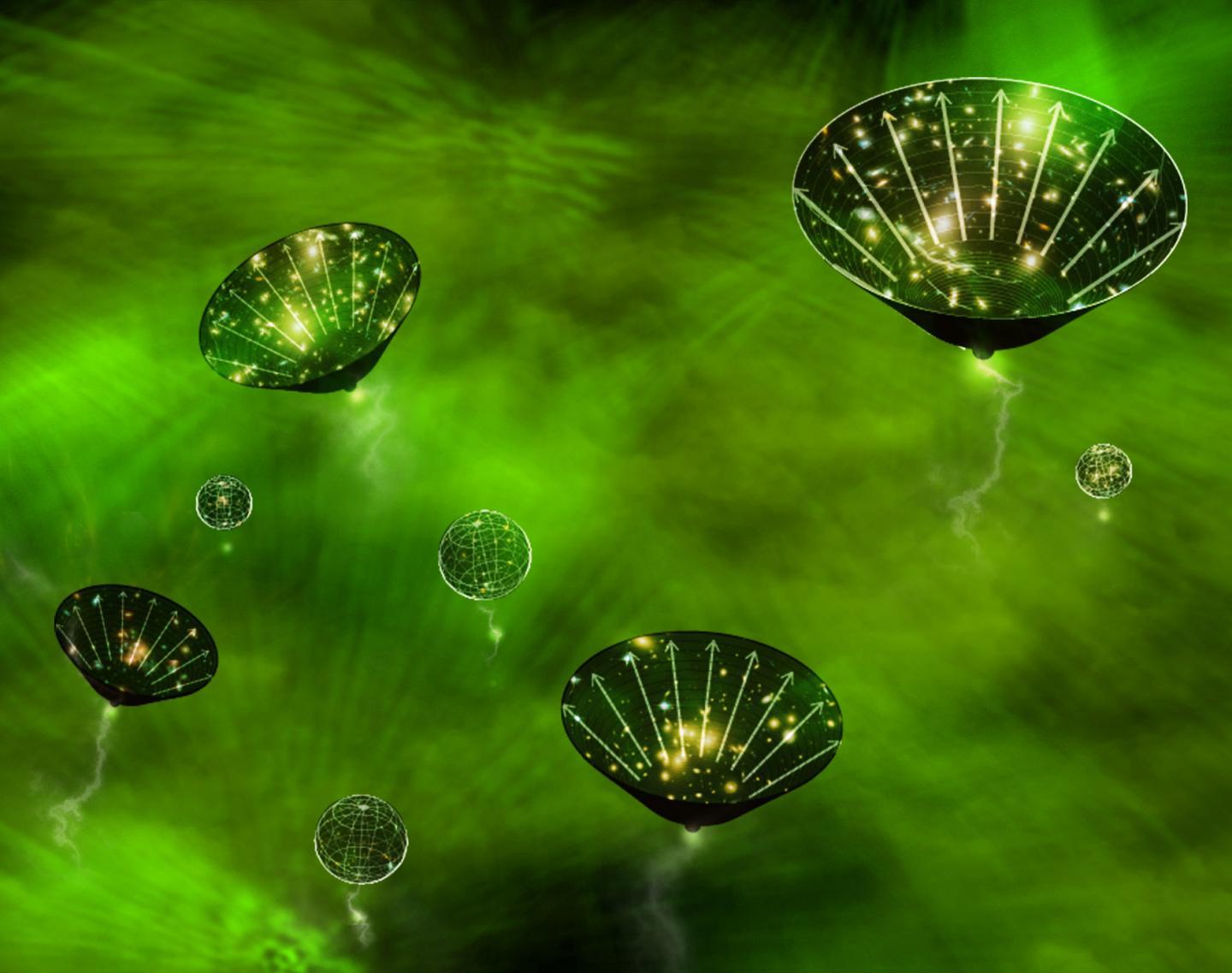
The answer you get for “How big would the event horizon be of a black hole with the mass-equivalent of all the matter-and-radiation within the observable Universe?” is a remarkable number: about 16.5 billion light-years. This is about a third of the actual radius to the edge of the observable Universe: 46.1 billion light-years. In fact, if it weren’t for the presence of dark energy — if we had more normal matter, dark matter, neutrinos, or photons in place of dark energy — these two values would actually equal one another.
Even though we don’t observe any evidence for white holes within our Universe, the fact that we had a Big Bang and the fact that we have black holes within our Universe is quite consistent with the idea that there’s a “white hole” on the other end of every black hole that’s ever been created.
In fact, going very deeply into the weeds, if you ask what happens when you fall past the outer event horizon of a rotating black hole, it turns out that what you experience looks very much like what we believe our Universe experienced just prior to the onset of the hot Big Bang: a period of exponential expansion, very much akin to what we today know as cosmic inflation.
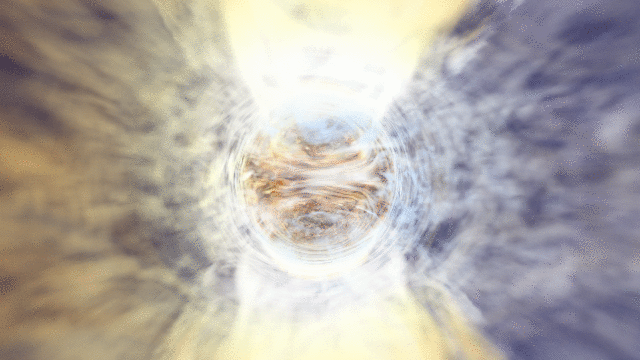
But do white holes actually exist? The truth is, we’ve never seen one, and we don’t expect to ever find one within our Universe. Event horizons, unfortunately, are very good at “hiding” whatever else occurs on the other side of them. There may be something very interesting at the central locations inside each black hole in our Universe, but we’ll never be able to access them. There may have been something very interesting occurring in whatever birthed our Universe before the onset of cosmic inflation and its aftermath: the hot Big Bang, but we have no way of obtaining any information about that time.
The sober truth, as much as we might detest it, is that the amount of information present in the Universe is finite, and renders us unable to reconstruct what’s going on (or what went on) on the “other side” of these events. It’s worth keeping in mind that general relativity admits white holes as an equal possibility to black holes, but that only the observational evidence for black holes has been found within our Universe. While mathematics can tell you the possibilities for what could occur, only observations, measurements, and experiments can tell you what does occur within the Universe. White holes remain an intriguing possibility, but their existence, at this point in time, can only be said to be speculative, at best.
Ethan is on medical leave until May 6th. Please enjoy a republication of this article from the Starts With A Bang archives!