Carbon-14 Spiked Worldwide Over 1200 Years Ago, And The Sun Is To Blame
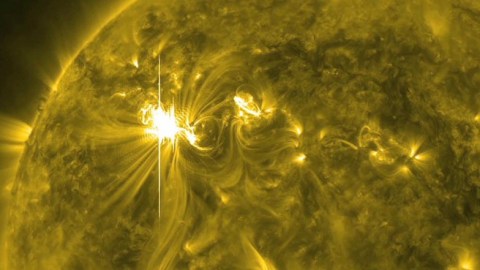
In 774/775, tree rings show a spike in carbon-14 unlike anything else. At last, scientists think they know why.
Every once in a while, science gives us a mystery that comes as a complete surprise. Typically, when we slice open a tree and examine its rings, we discover three different forms of carbon in each ring: carbon-12, carbon-13, and carbon-14. While the ratios of carbon-12 and carbon-13 don’t appear to change with time, carbon-14 is a different story. Its abundance slowly decays with a half-life of a little over 5,000 years, with a typical variation of about 0.06% from year-to-year in the rings.
But in 2012, a team of Japanese researchers were analyzing tree rings dating to the years 774/775, when they noticed an enormous surprise. Instead of the typical variations they were used to, they saw a spike that was 20 times larger than normal. After years of analysis, the unlikely culprit has finally been revealed: the Sun. Here’s the scientific story of how we know.
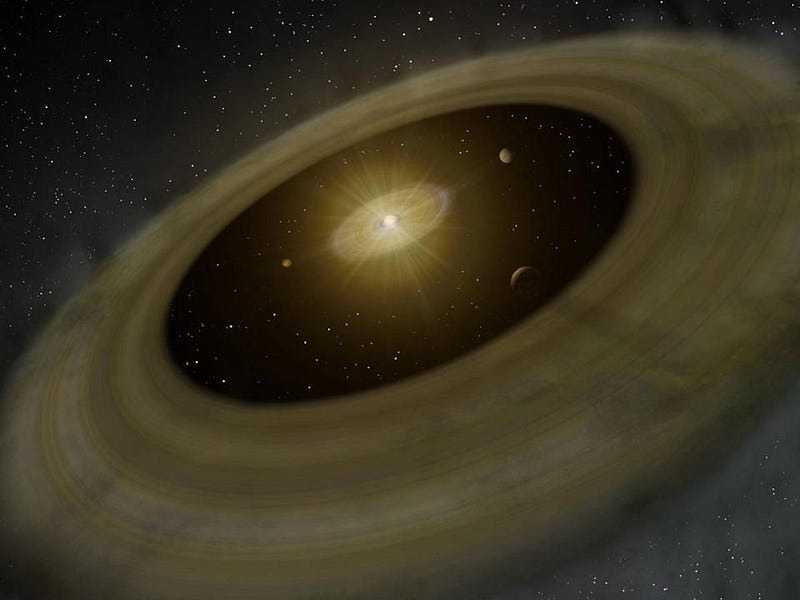
Long ago, our Solar System formed from a molecular cloud of gas. Embedded amidst the hydrogen and helium left over from the Big Bang were the full suite of heavy elements making up the remainder of the periodic table, returned to the interstellar medium from the corpses of previous generations of stars. Prominent among those elements was carbon, the fourth most common element in the entire Universe.
Most of the carbon that exists on Earth, formed from that long-ago event, is carbon-12, consisting of six protons and six neutrons in its nucleus. A tiny fraction of our carbon, about 1.1%, is in the form of carbon-13, with one extra neutron compared to its more common carbon-12 counterpart. But there’s another form of carbon that’s not only rare but unstable, carbon-14 (with two extra neutrons over carbon-12), that holds the key to unlocking this mystery.
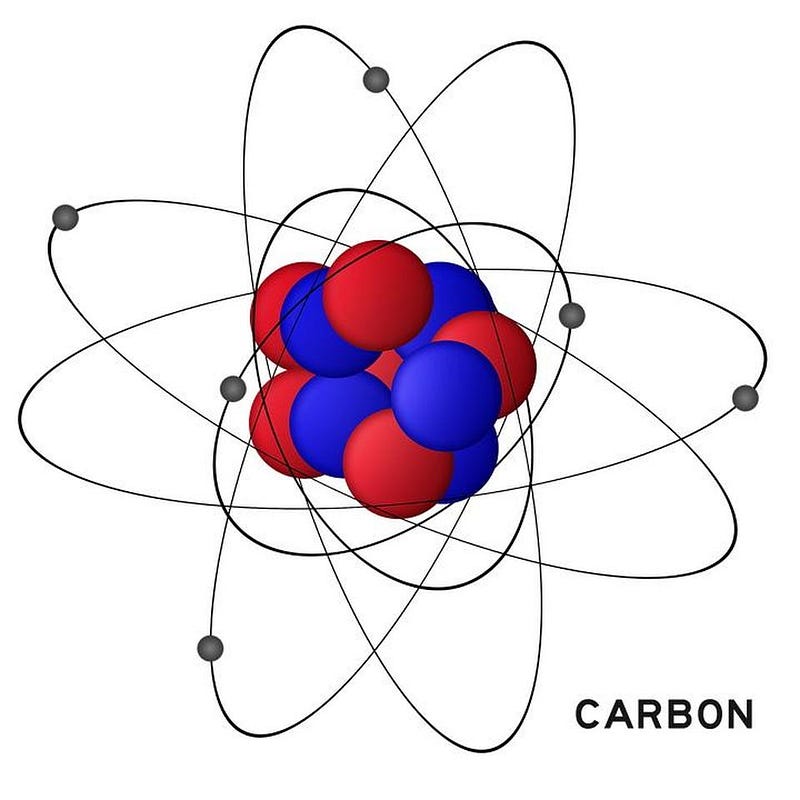
Unlike carbon-12 and carbon-13, carbon-14, with six protons but eight neutrons in its nucleus, is inherently unstable. With a half-life of a little more than 5,000 years, carbon-14 atoms will decay into nitrogen-14, emitting an electron and an anti-electron neutrino when the decay occurs. Any carbon-14 atoms that were created prior to the formation of Earth would have all decayed away long ago, leaving none of them behind.
But here on Earth, we do have carbon-14. Approximately 1 out of every one trillion carbon atoms have eight neutrons in them, indicating that there must be some way for those unstable isotopes to be produced on Earth. For a long time, we knew that carbon-14 existed, but we didn’t understand its origin. In the 20th century, though, we finally figured it out: carbon-14 comes from high-energy cosmic particles that collide with our world.
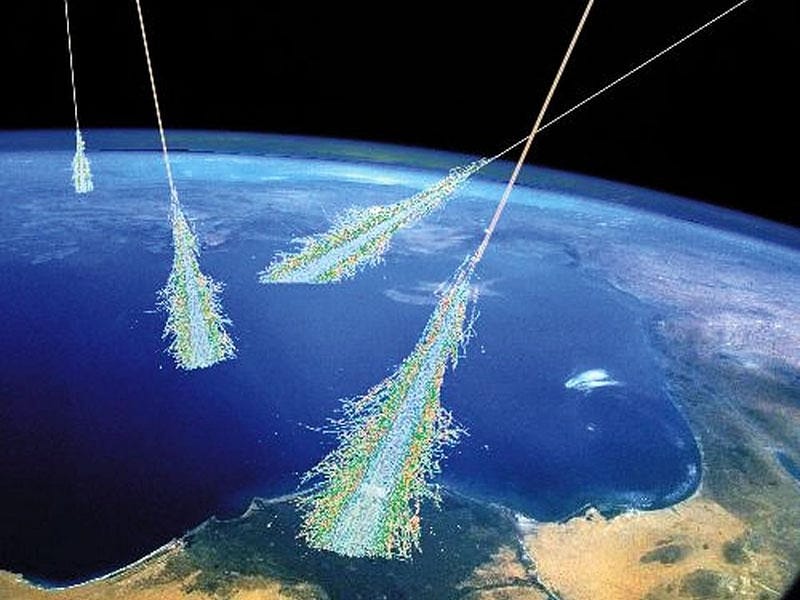
From sources such as the Sun, the stars, stellar corpses, black holes, and even galaxies outside the Milky Way, space is flooded with these high-energy particles known as cosmic rays. Most of them are simple protons, but some are heavier atomic nuclei, others are electrons, and a few are even positrons: the antimatter counterpart of electrons.
Regardless of their composition, the first thing a cosmic ray will collide with when it encounters the Earth is our atmosphere, which leads to a cascading chain reaction of interactions. A variety of new particles will be produced, including photons, electrons, positrons, unstable light particles like mesons and muons, and more familiar particles like protons and neutrons. In particular, the neutrons are incredibly important for the production of carbon-14.
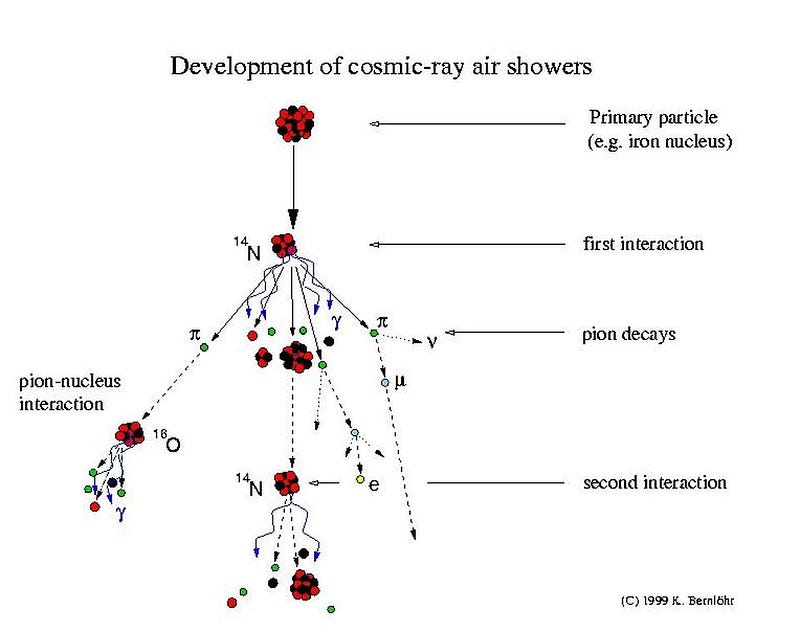
Most of Earth’s atmosphere — about 78% — is made up of nitrogen gas, which itself is a diatomic molecule made of two nitrogen atoms. Each time a neutron collides with a nitrogen nucleus, which consists of 7 protons and 7 neutrons, there’s a finite probability that it will react with that nucleus, replacing one of the protons. As a result, a nitrogen-14 atom (and a neutron) transforms into a carbon-14 atom (and a proton).
Once you produce that carbon-14, it behaves just like any other atom of carbon. It readily forms carbon dioxide in our atmosphere, and mixes throughout the atmosphere and the oceans. It gets incorporated into plants, consumed by animals, and readily makes its way into living organisms until it reaches equilibrium concentrations. When an organism dies (or a tree ring is fully formed), no new carbon-14 enters it, and so all the existing carbon-14 slowly but steadily decays away.
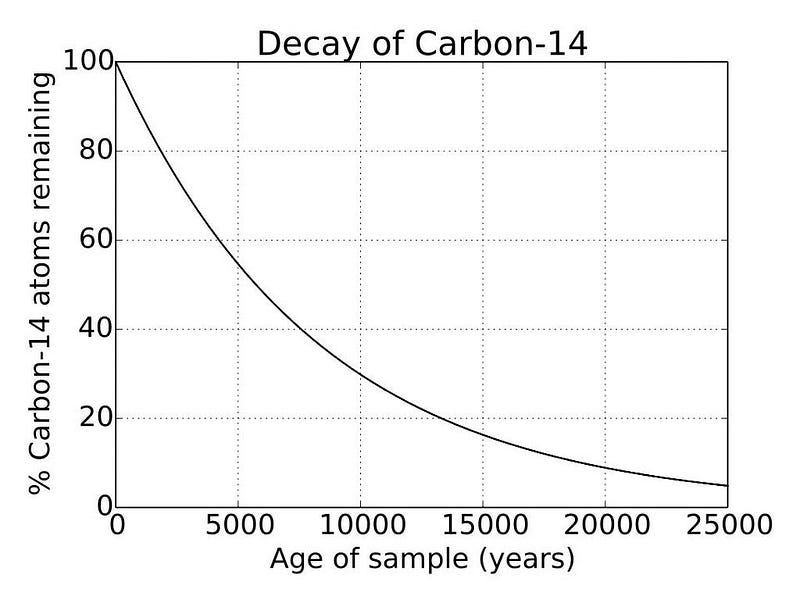
When you hear the term “carbon dating,” this is what scientists are referring to: measuring the carbon-14 to carbon-12 ratio. If we know what the initial carbon-14 to carbon-12 ratio was when an organism was alive (because it only varies by ~0.06% from year-to-year, typically), and we measure what the carbon-14 to carbon-12 ratio is today (where some of it has decayed away due to its unstable, radioactive nature), we can infer how long it’s been since that organism stopped uptaking carbon-14.
As far as we can tell, the levels of carbon-14 remained roughly constant throughout the world throughout the past few millennia. The only known fluctuation in this pattern, at least as of the early 2010s, was from the detonation of nuclear weapons in the open air. And yet, in 2012, we got a scientific shock: in approximately the year 774/775, two independent cedar trees in Japan were analyzed for carbon-14 in their rings, and saw a tremendous spike that was some 20 times larger than natural variations could explain.
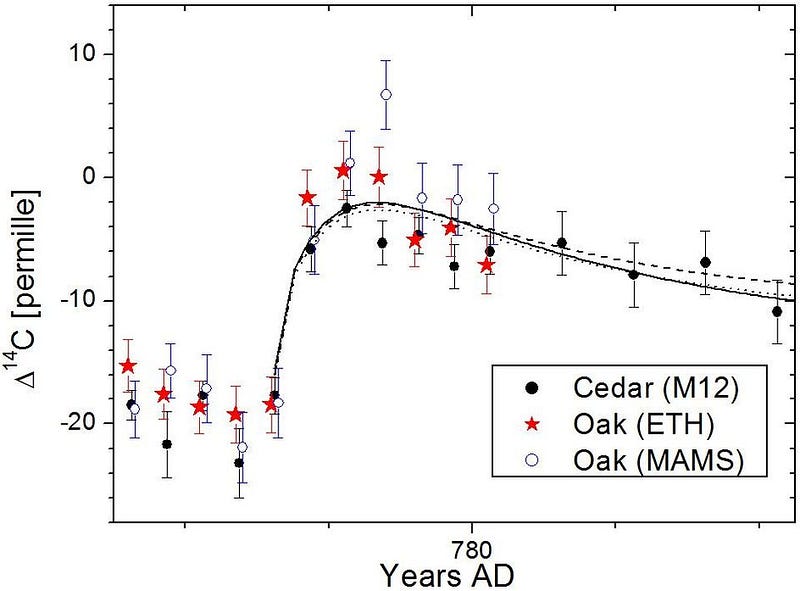
The only natural explanation that makes any sense is if, right around that time, Earth experienced an excessive bombardment of these cosmic rays, creating a spike in the amount of carbon-14 that gets created. Even though it’s a small excess in absolute terms — just 1.2% more carbon-14 than normal — it’s far above any natural variation that we’ve ever seen.
Moreover, it’s a spike that’s subsequently been confirmed to exist in tree rings around the world, from Germany to Russia to New Zealand to the United States. The results agree across countries, and could be explained by anything from increased solar activity to a cosmic flare to a direct hit from a distant gamma-ray burst. But the carbon-14 evidence has subsequently been joined by a few other historical and scientific peculiarities, and with the latter enabling us to solve the mystery.
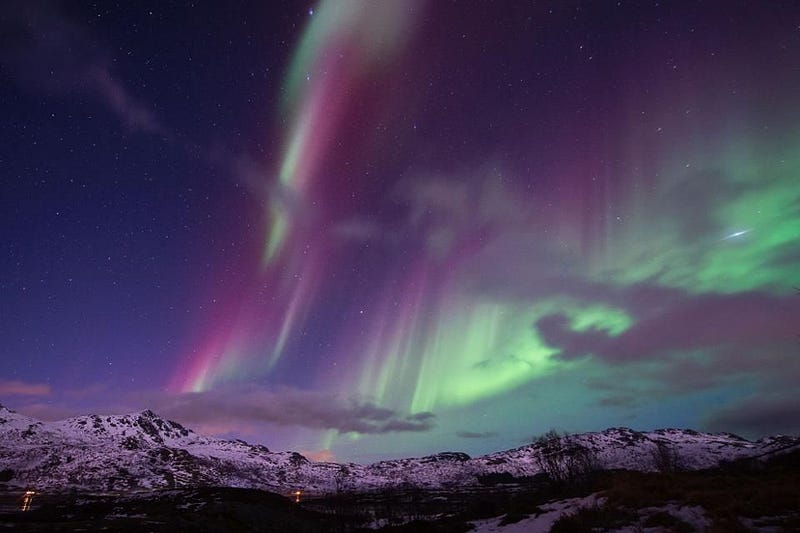
Historically, a “red crucifix in the heavens” was recorded in the Anglo-Saxon Chronicle of 774, which could correspond to either a supernova (no remnant has ever been found) or to an auroral event. In China, an anomalous “thunderstorm” was recorded in 775, so notable that it was the only such event recorded.
But scientifically, the tree-ring data has been joined by ice core data from Antarctica. While the tree-rings show a spike in carbon-14 in 774/775, the ice core data shows a corresponding spike in radioactive beryllium-10 and chlorine-36, which suggest an association with a strong, energetic event of solar particles. An event such as this would have perhaps been on par with the now-famous Carrington event of 1859, which is the largest recorded solar storm in recent history, with the historical data remaining consistent with this explanation as well.
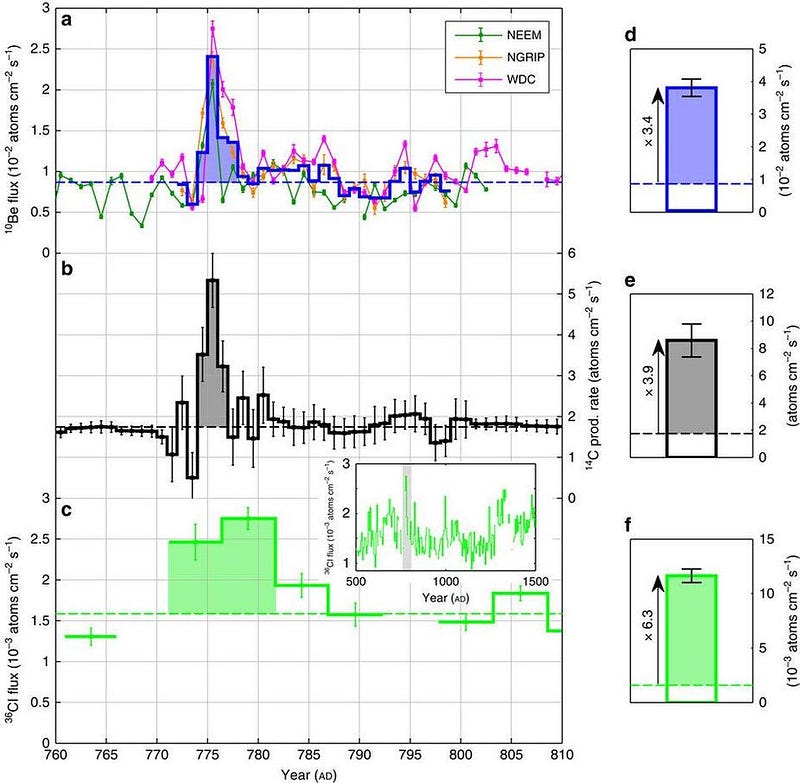
Two other events have subsequently been unearthed that could show similar spikes in these isotopes: a slightly weaker outburst in 993/4 and an even earlier one dating back to ~660 BCE. The combined data from all three events point to a common origin that necessarily involves a large flux of protons in a specific energy range.
This is consistent with a relatively common event seen in the Sun: the ejection of solar protons. However, it’s not consistent with the gamma-ray burst scenario, which cannot produce the necessary proton flux to explain the beryllium-10 simultaneously. The same Japanese team that initially suggested the gamma-ray burst explanation for the 774/5 tree-ring data, upon conducting their own measurements of the 993/4 event, concluded:
it is highly possible that these events have the same origin. Considering the occurrence rate of [carbon-14] increase events, solar activity is a plausible cause of [these] events.
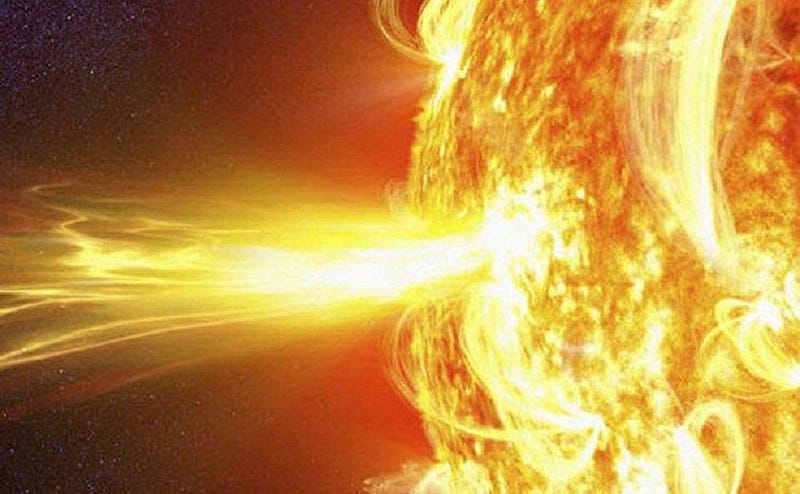
Every so often, the Sun does eject energetic particles right in the direction of Earth. Sometimes the Earth’s magnetic field deflects them away, other times it funnels these particles down into our atmosphere. When they arrive, they can create aurorae, disrupt our local magnetic fields, and — if we’re technologically advanced — they can induce all sorts of current in our electrical grids and devices, potentially causing trillions of dollars worth of infrastructure damage.
We now know there are a variety of solar events that impact Earth, and that the largest-magnitude events we’ve experienced occur more than once-per-millennium. We cannot predict when the next one will arrive, but it’s certain that the consequences for human society will be greater than they’ve ever been when it comes. Carbon-14 levels will certainly spike again in the future, but when that happens, a lot more than tree rings and ice cores will be affected. It’s up to us, collectively, to decide how we’ll prepare.
Ethan Siegel is the author of Beyond the Galaxy and Treknology. You can pre-order his third book, currently in development: the Encyclopaedia Cosmologica.