Cold Dark Matter Is Heated Up By Stars, Even Though It Cannot ‘Feel’ Them
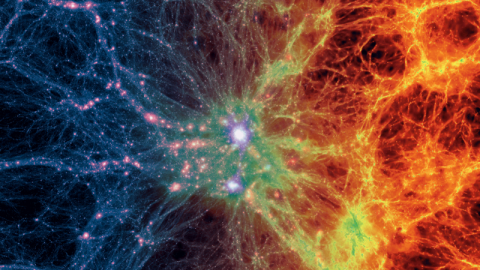
If dark matter doesn’t interact with normal matter or light, how can it be heated up?
One of the great cosmic mysteries of our time is the presence and existence of dark matter. Unlike normal matter, which is made of known particles that can emit, absorb, or otherwise interact with light and the other known particles, dark matter simply passes through both itself and everything else. It is completely invisible, as far as we can tell, except for one effect: it appears to have a gravitational mass. It affects the curvature of spacetime, and holds galaxies, clusters of galaxies, and the great cosmic web together.
When we run our simulations, however, we get very specific predictions for the structures that dark matter should form. The cosmic web lines up, but the smaller, galactic scales don’t. Long touted as the biggest problem for cold dark matter, scientists have uncovered the solution: dark matter gets heated up by stars. Here’s the story of how that happens.
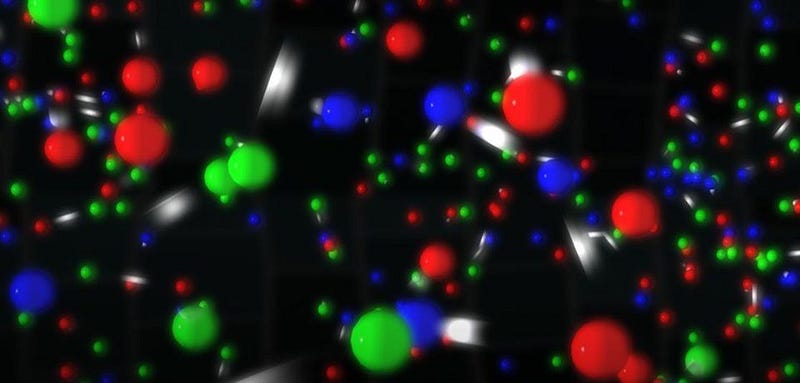
Imagine the Universe as it might have been in the earliest stages after the Big Bang. It’s hot, dense, and full of matter and radiation. Only, instead of the particles you might exclusively think of — the subatomic particles that make up atoms, for instance — there’s five times as much dark matter. At these early times, particles of normal matter smash into one another and into photons, but dark matter passes through everything, refusing to collide.
It’s as though dark matter is 100% permeable: normal matter passes through it, antimatter passes through it, photons pass through it, even other dark matter particles pass through it. It’s only because dark matter is cold, or moving very slowly compared to the speed of light, that it can eventually gather together into gravitational clumps. Over time, it does exactly that, pulling the normal matter into the gravitational wells it created at early times.

What we wind up with, then, is a Universe populated with regions of space containing spheroidal distributions of matter: both normal and dark. Over time, the normal matter will collide with other normal matter particles and stick together, forming molecules, clouds of gas, and releasing radiation. The normal, atom-based matter will sink to the center of each such region, where it will typically form a rotating, disk-like shape: what we know as a galaxy.
Meanwhile, the dark matter is incapable of doing any such thing. It remains in a large, diffuse halo surrounding the galaxy itself. This should be independent of galaxy size or scale, as simulations show. Regardless of how massive the overall galaxy is, there should be a halo of dark matter extending into space by a factor of ten or more over the disk itself. This is true for Milky Way-sized galaxies, larger ones, and even tiny dwarf galaxies.
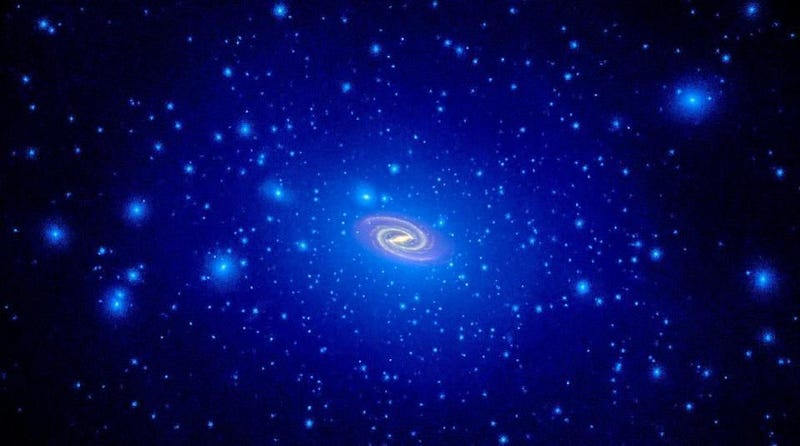
This is the standard picture: one that’s been a cornerstone of modern astrophysics for more than 20 years. But recently, observations of dwarf galaxies — galaxies between 0.1% and 1% as massive as our own galaxy — have shown that this idea of a universal dark matter profile does not fit the data very well. In particular, many of these galaxies show evidence that there’s less dark matter in the very interiors of these galaxies, or at their central cores, than these simulations predict.
If we run our simulations of a galaxy with dark matter alone, this cannot be the case. But if we take what we already know:
- that dark matter doesn’t interact with itself or normal matter or radiation,
- that normal matter can interact with itself and with radiation, but not dark matter,
- and that normal matter and dark matter can communicate through the gravitational force,
a possible solution appears to emerge.
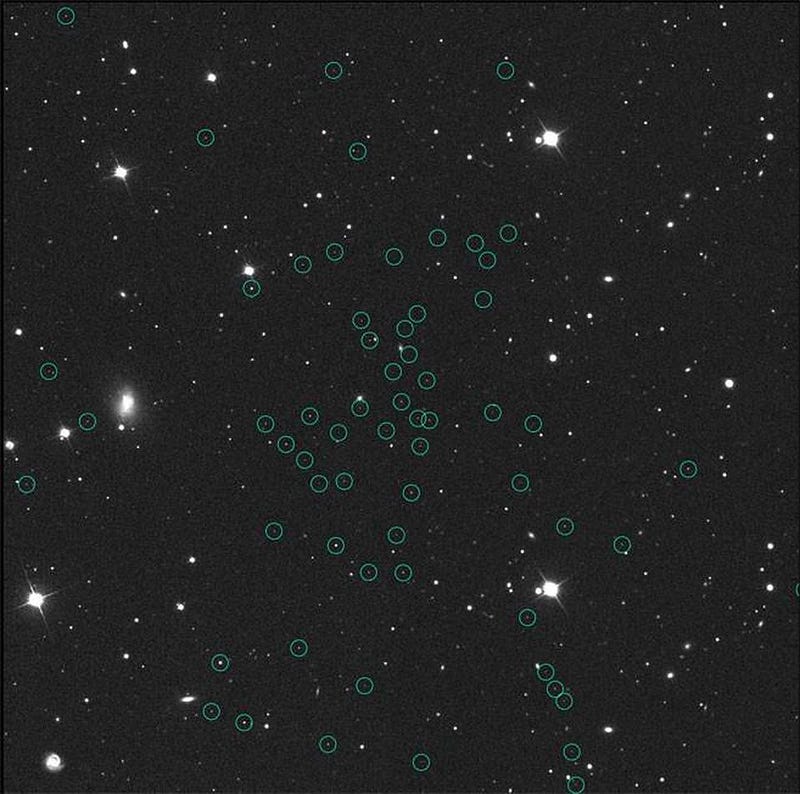
The way to think about it is to visualize what’s going on with the normal matter in the center of this galaxy when it forms a large number of new stars. The gas present contracts down, creates new stars of a variety of masses, and starts to experience radiation emanating from the young stars that have recently formed there.
It’s the hottest, most massive stars that emit the most radiation, and those stars also emit matter particles. These stellar winds work to push gas and dust away from the center of the galaxy, giving it a boost of kinetic energy. All of that normal matter had concentrated in the core of the galaxy, and this new, important burst of star formation has worked to push it away. The galaxy’s center now has less matter — normal matter, that is — than it had before.
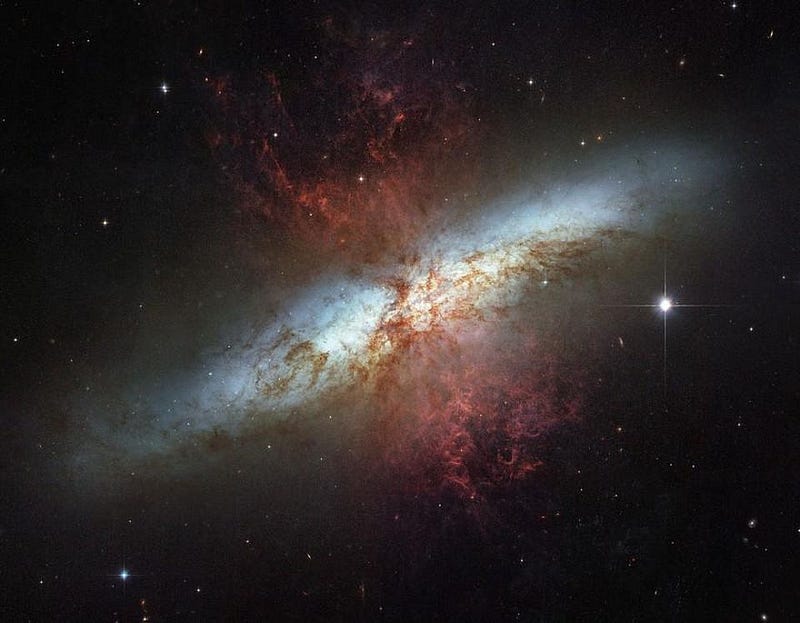
What happens next?
Well, think about what would happen to the planets in the Solar System if you removed a large amount of mass from the Sun. It’s that large, central mass that keeps them in their stable, nearly-circular orbits. If the mass increased, they would spiral inwards; if the mass decreased, they would spiral outwards.
When galaxies form stars, it’s as though the central region loses mass, which causes all the matter around it to feel a lessened gravitational pull. Yes, the normal matter gets expelled because of the radiation, winds, and pressure. Once that’s gone from the center, though, all the matter present — both normal and dark — has less of a gravitational pull to keep it in place. The only recourse is to move to a higher, less-tightly-bound orbit.
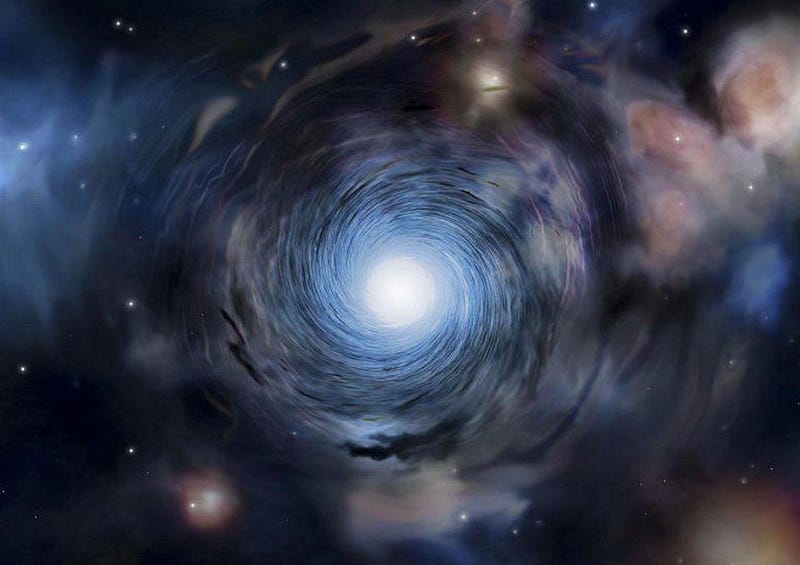
This effect is what’s known as “dark matter heating.” It isn’t that any of the radiation from the stars or any of the heat from the normal matter is getting transferred to the dark matter itself; it doesn’t involve temperature or energy transfer directly.
Instead, what’s happening is that the additional energy imparted to the normal matter is expelling it from where it was previously the most concentrated: in the galactic center. Once that normal matter is removed from the galactic center, there’s less mass there to hold the dark matter in place, and it, too, has to move to a higher, less-tightly-bound orbit. Because the dark matter gets pushed out and bumped to a higher, more energetic orbit, it has the same effects as though the dark matter was given an extra burst of energy. It’s not actually hotter than it was previously, but the effects are identical.
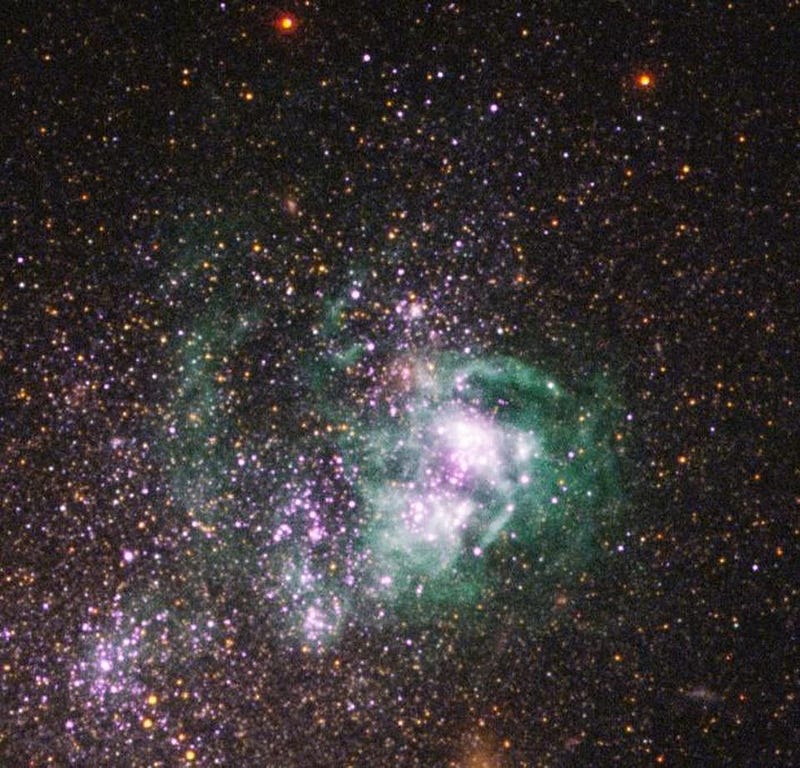
Over their lifetimes, galaxies of all types experience multiple cycles of gas flowing in and out of the central regions. When gas concentrations reach a very high level, it can trigger new star formation; when gas concentrations reach a low level, new star formation is impossible.
So what does this mean for the dwarf galaxies you’d actually find, if this idea is correct?
It means that if a galaxy had only a few small bursts of central star formation, the dark matter in the core wouldn’t have gotten heated by very much. Most of it would still be present. You’d expect a relatively high value of dark matter at the centers of dwarf galaxies that had very little history of star formation in their centers.
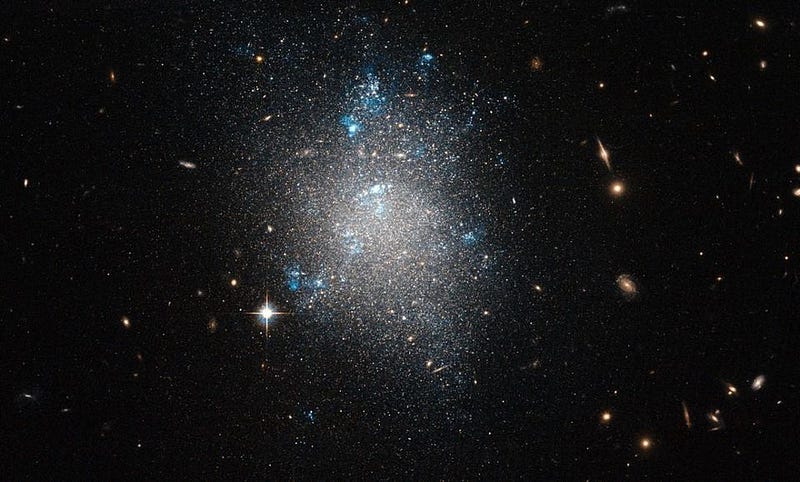
But if a galaxy had formed large amounts of stars over its history, you’d instead expect that the gas and matter near the center of the galaxy would be largely expelled, which pushes the dark matter to higher orbits, and changes the inferred mass profile of the galaxy. Practically all galaxies had starbursting phases during the first few billion years, but the least active ones have been quiet for the billions of years subsequent to that. In other words, a rich history of recent star formation should lead to a low-mass dark matter core in dwarf galaxies, while solely ancient star formation should lead to higher-mass cores.
That’s exactly what a team led by Justin Read found in a new study released in January. According to Dr. Read:
We found a truly remarkable relationship between the amount of dark matter at the centres of these tiny dwarfs, and the amount of star formation they have experienced over their lives. The dark matter at the centres of the star-forming dwarfs appears to have been ‘heated up’ and pushed out.
It’s a spectacular case of a more sophisticated simulation explaining a phenomenon that previous simulations, making more naive assumptions, could not account for.
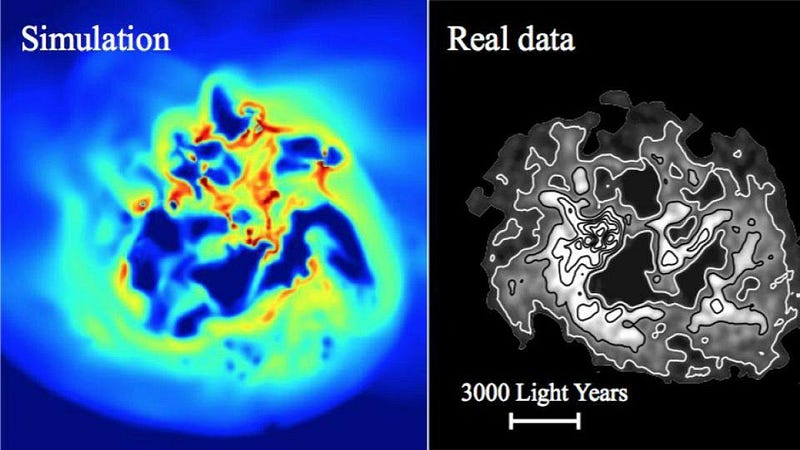
Traditionally, dark matter has been the go-to explanation for the phenomena we’ve observed on large cosmic scales. It explains the fluctuations in the cosmic microwave background, the large-scale structure of the Universe, and the behavior of clusters and groups of galaxies in a way that no alternative can. However, the smallest galactic scales have proven problematic for dark matter simulations, leading many to question its validity.
This new discovery is a fascinating case where theory and observation are lining up perfectly once better calculations are done. It may finally resolve one of the greatest problems for dark matter: explaining the behavior of the smallest galaxies in the Universe. Even with no direct energy transfer, dark matter is affected by the gravity of everything around it. If star formation moves mass around, dark matter will move around, too. Cold dark matter, indirectly, gets heated up by stars. At long last, we’re finally understanding how.
Ethan Siegel is the author of Beyond the Galaxy and Treknology. You can pre-order his third book, currently in development: the Encyclopaedia Cosmologica.