Evaporating exoplanet WASP-69b solves two planetary puzzles
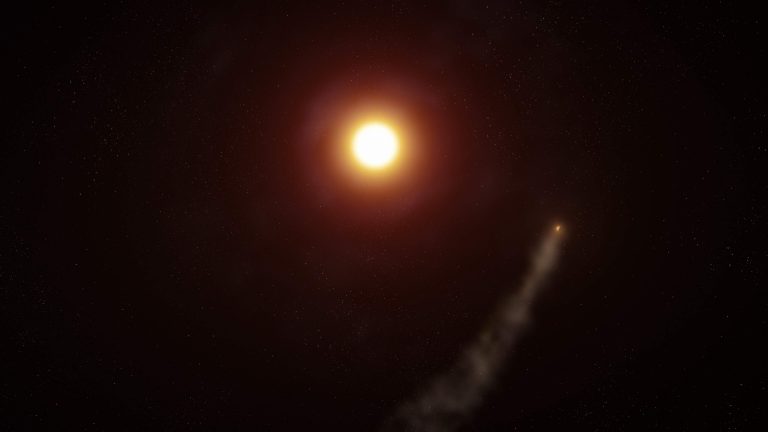
- With more than 5,000 exoplanets known, we can finally characterize them by properties, including mass, radius, and orbital period around their parent stars.
- A few surprising puzzles arise: there are hot Earths and hot Jupiters, but no hot Neptunes, and there are super-Earths and mini-Neptunes, but no worlds in between.
- This isn’t necessarily just the way it is; there’s a possible explanation for both. By observing exoplanet WASP-69b, we may have stumbled upon the solution.
It was only a little over 30 years ago, in the early 1990s, that humanity detected our first planets in orbit around stars other than the Sun: the exoplanets. The earliest ones discovered were a bit of a surprise: they were all massive, in tight orbits around their parent stars, and extremely hot: a class known as hot Jupiters. Since that time, we’ve discovered more than 5000 exoplanets, ranging from sub-Earth sized all the way up to super-Jupiters, with a huge variety found in between. However, two puzzles have arisen:
- the fact that there are “hot Earths” and “hot Jupiters,” but no “hot Neptunes” in between them,
- and the fact that there are plenty of Earth-like planets up to about 140% the radius of Earth, and plenty of Neptune-like planets down to about half the size of Neptune (about 200% the radius of Earth), but preciously few planets in that in-between range: a puzzle known as the radius gap.
Although there are suspects in the quest to solve these cosmic puzzles, the direct supporting evidence has been elusive up until now. However, a new paper that investigates the remarkable properties of evaporating exoplanet WASP-69b may provide the key clue that leads to a solution for both. Here’s how.
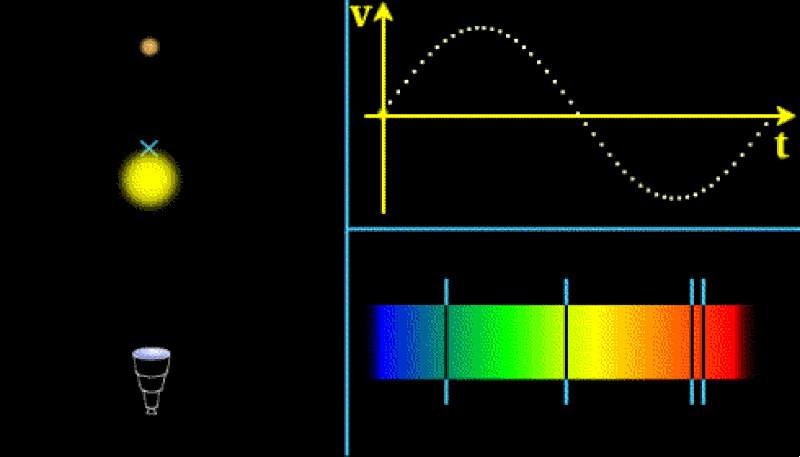
The first successful methods for finding exoplanets involved measuring the light from a parent star very exquisitely. If there are planets orbiting the star in question, the star isn’t only going to gravitationally pull on that planet, but the planet will gravitationally pull on the star, causing the star to move in an elliptical pattern around the mutual center-of-mass of the star-planet system. That causes the star to appear to “wobble” with respect to us, as it will periodically move toward-and-away from us leading to its light redshifting and blueshifting in a periodic fashion. This detection method, known as either the radial velocity or stellar wobble method, is most sensitive to high-mass planets in close orbits to their parent stars.
Similarly, a planet that transits across the face of the star as seen along our line-of-sight to it will block a fraction of that star’s light, causing a periodic flux dip in the light seen from the star over time. This is again most sensitive to planets that block a large fraction of their parent star’s light with a higher frequency. These two methods, now being joined by others such as direct imaging and microlensing, have revealed the overwhelming majority of the more-than-5000 exoplanets presently known, and already, the exoplanet population shows a few surprises.
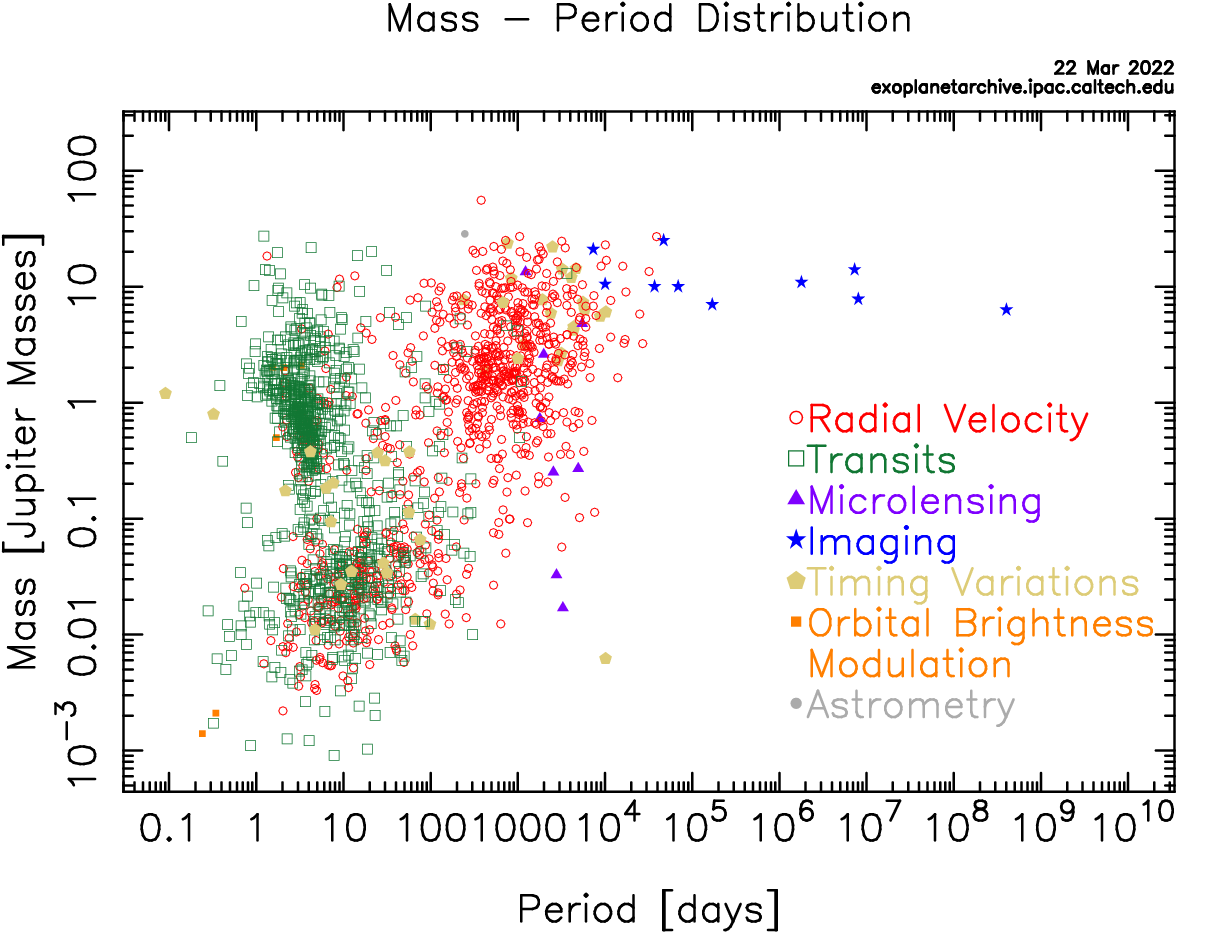
In particular, there are two puzzles that appear when we examine the data in detail.
- There are plenty of hot Earth-sized planets, and also plenty of hot Jupiter-sized planets, with hot Earth-sized planets common around low-mass stars and hot Jupiter-sized planets common around higher mass stars. However, there are pretty much no classes of stars that show the existence of hot Neptune-sized planets around them. It’s almost as though there’s a process at play that forbids the existence of lower-mass, gas-rich worlds, and we don’t know exactly why.
- There are also lots of Earths and super-Earths found in the exoplanet data, including all radii from all types of stars (at least, as far as we can measure them), as well as lots of Neptunes and mini-Neptunes that exist, particularly at distances that lead to the planet not being “too hot,” or too close to their parent star. However, there aren’t very many of the “in between” sized planets, which is known as either the radius gap or the Fulton gap in the literature.
There are a few ideas floating around out there that attempt to explain this puzzle, but ideas in science aren’t worth very much unless we can test and confront them with actual, meaningful data.
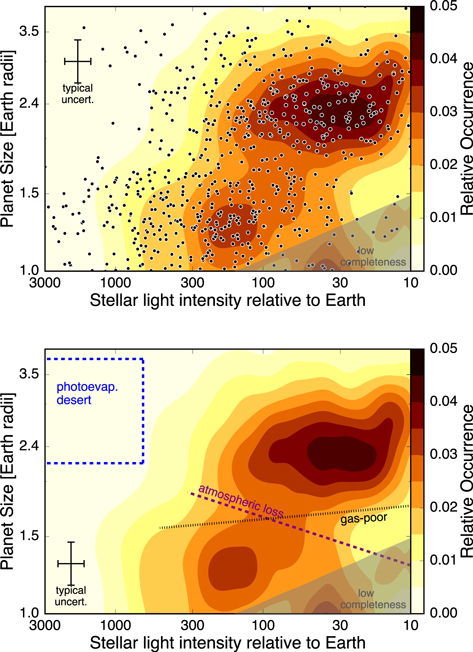
One interesting hypothesis, however, could explain both of these phenomena at once: the process of photoevaporation. Photoevaporation is a fancy way to say:
- there are a lot of energetic emissions that come from stars, including ultraviolet light and energetic particles,
- and those energetic emissions are going to not only heat any planets they encounter, but will collide with any particles that exist within those planetary atmospheres,
- and that if those atmospheric particles get energized beyond a certain threshold, they’re going to get kicked up to speeds that exceed the escape velocity of that world,
- and hence, the atmosphere will begin to be stripped away.
There could be a critical threshold in both cases. For the hottest planets, either they’ll simply become stripped planetary cores, incapable of holding onto any substantial atmosphere, or they’ll be massive enough that they can hang onto large volatile (hydrogen and helium rich) envelopes, but not in between. And for less hot planets, either you aren’t massive enough to hold onto a volatile envelope, in which case you’re a rocky, Earth-like planet, or you are massive enough, in which case you’re an inflated, Neptune-like planet. If you’re even a little less massive than the Neptune-like worlds, your volatiles are photoevaporated away, and you’d transition into an Earth-like state.
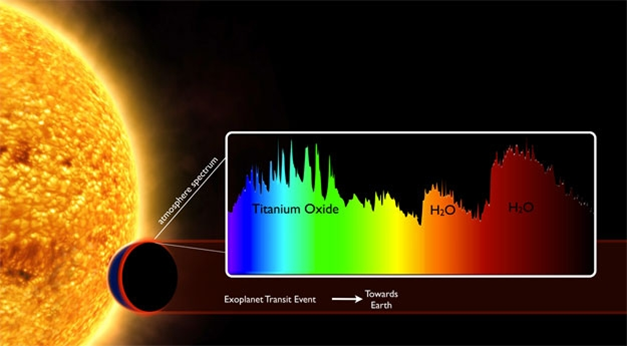
It’s a challenge to observe these types of planets during the stages where they’re still forming, as the observational difficulties have not yet been overcome. But there is a very reasonable way to investigate the issue: by looking at the least massive hot Jupiter planets found around relatively young, massive stars, and to try and measure and quantify the effects of photoevaporation. If we can understand how these worlds, the least massive ones that still have volatiles, lose their atmospheric mass owing to the influence of their parent stars, perhaps we can learn lessons about photoevaporation and how it plays (or doesn’t play) a role in explaining these two big planetary puzzles.
In other words, what we want to do is to study systems that are experiencing photoevaporation, in real-time and in situ, to learn how efficiently parent stars can strip their orbiting planets’ volatile atmospheres away. One intriguing system to study is the WASP-69 system. WASP-69 is a K-class star, a little bit smaller and less massive than the Sun, but a little bit younger: at just 2 billion years of age. WASP-69 also has an exoplanet that orbits it: WASP-69b, roughly a Saturn-mass, slightly larger-than-Jupiter in radius exoplanet, that’s close enough to its parent star, and hence hot enough, to experience photoevaporation.
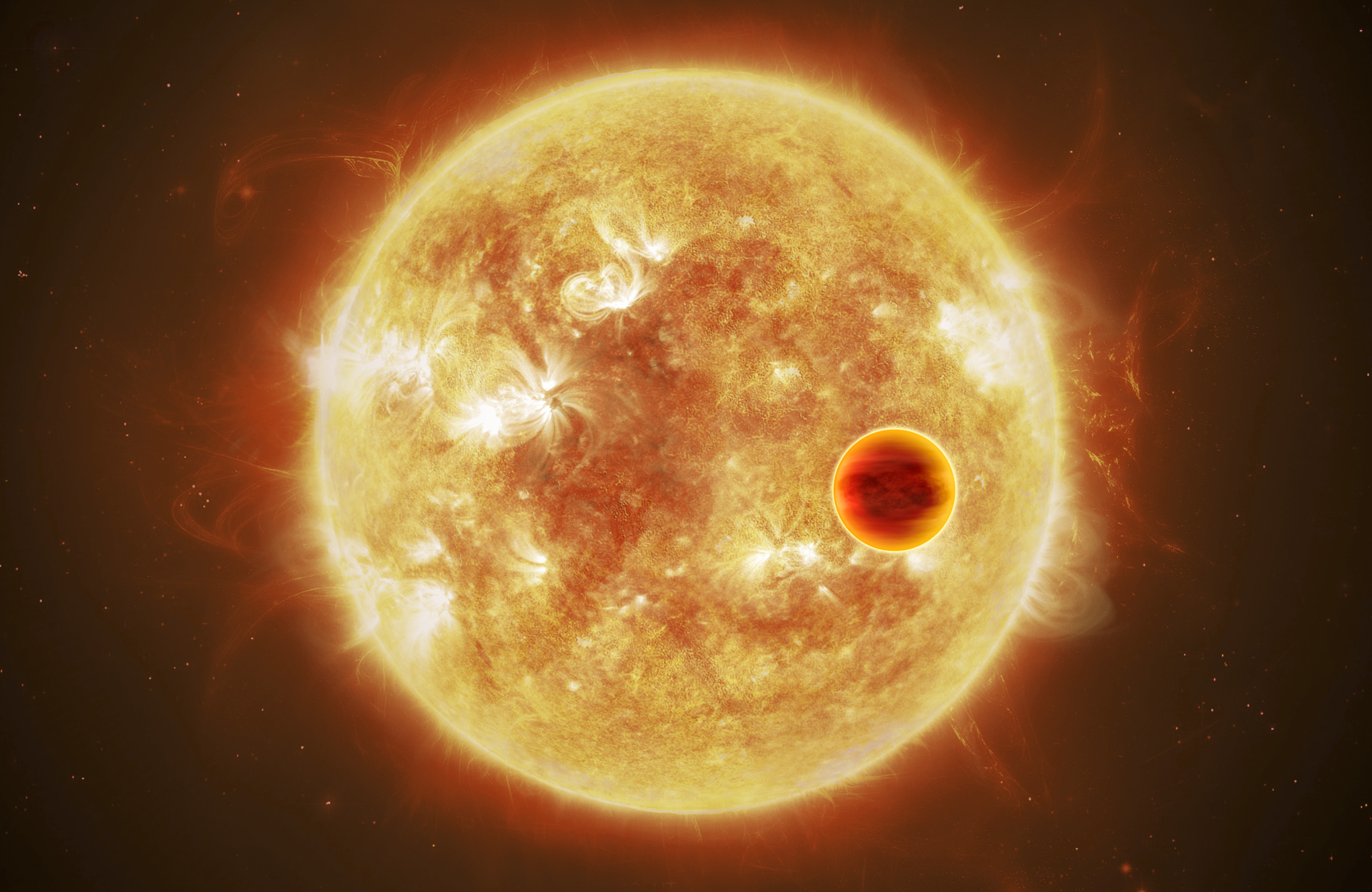
Why would a planet only about the mass of Saturn actually be larger than Jupiter? The answer comes from the parent star itself: because when you heat gas up, even in an exoplanet’s atmosphere, it expands, causing the exoplanet to become “puffier” in terms of its radius. The puffier your atmosphere is, the farther away the most tenuously-held atmospheric particles are from the planet’s center-of-mass, making its escape velocity lower. The hotter your planet is and the lower the escape velocity from it is, the greater the rate of photoevaporation will be.
If we can measure the photoevaporation rate directly, that can provide us with valuable information that teaches us which sorts of planets (and planetary atmospheres) can persist within exoplanetary systems in a stable fashion, and which sorts of planets (and planetary atmospheres) will be inherently unstable, and won’t persist for billions of years.
Because WASP-69b is an exoplanet that transits in front of its parent star, you can not only measure the properties of the exo-atmosphere during the transit, but whether there are any features that appear either before or after the transit itself if you can obtain good enough data. And fortunately, Dakotah Tyler and his team were able to get Keck telescope time to take exactly this type of data for themselves.
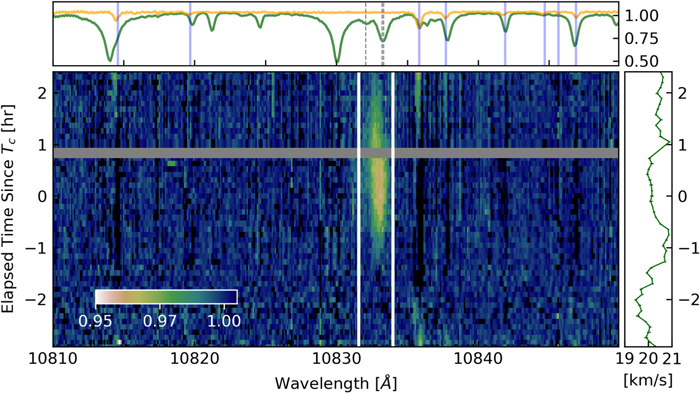
What did they see when they actually took this valuable data?
Importantly, they took not only photometric (raw light) data, but spectroscopic data, where the light from the parent star is broken up into its individual wavelengths. As the planet first makes contact with the limb of the star, relative to our line-of-sight, some of the starlight is not only blocked by the disk of the planet, but some of it filters through the atmosphere of the planet itself. When there’s neutral gas, such as hydrogen or helium, in between the star and our line-of-sight, a characteristic absorption signal – observable at an element-specific wavelength – will appear in the data.
When Tyler and his team acquired the data, they found something fascinating that had only been suggested, never robustly seen, by previous studies.
- Prior to the transit, no absorption signal was seen, just as expected.
- During the transit, an absorption profile consistent with plenty of neutral helium in this atmosphere is shown to exist: confirming that this is a volatile rich gas envelope.
- But even after the transit has completed, a significant amount of neutral helium in a signal still persists for quite a long time, confirming the existence of copious amounts of neutral gas well-separated from the planet itself.
The best way to interpret this data? The atmosphere of WASP-69b is being photoevaporated, and that photoevaporation process is creating an enormous, massive, comet-like tail emerging from this hot exoplanet.
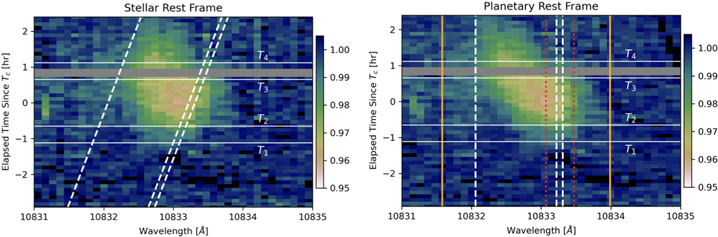
The neutral helium signal seen is incredibly informative. In detail, it tells us a number of fascinating facts.
- First off, material is being lost spectacularly fast from this exoplanet: at the rate of about one Earth-mass of atmosphere every ~1 billion years.
- Second off, that mass is departing this planet in a giant wake, extending for more than seven times the radius of the planet, or for a distance in excess of 350,000 miles (around 580,000 km) behind the planet itself.
- And third, because this is spectroscopic data, they can determine that this helium is being stripped away with a relative velocity of 23 km/s – around 50,000 miles-per-hour (80,000 km/hr) – which is consistent with photoevaporated material that then interacts with the star’s outgoing winds.
This data is enough to draw some fascinating inferences. It tells us that hot Jupiters and even hot Saturns are stable on scales of the lifetime of a typical star, as this exoplanet, which weighs in at around 90 times the mass of the Earth, is only losing ~1 Earth mass of atmosphere for every billion years of time that passes. Additionally, it tells us that “rocky cores,” or stripped planetary cores, would also be stable on the lifetime of a typical star, as the heavy elements making up a planetary surface wouldn’t be photoevaporated in the same way.
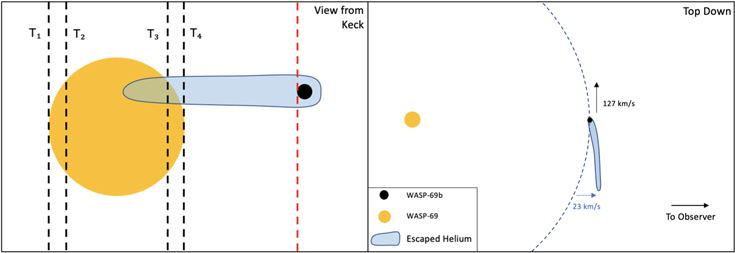
In other words, this data is very suggestive of the idea that the reason “hot Neptunes” don’t exist is because, if they’re hot, their atmospheres will be quite inflated, leading to very low escape velocities for the top layers of their atmospheres. Lower escape velocity means that the rates of atmospheric stripping will be greater, and that may very well mean that unless there’s a large amount of mass – enough to build up to a Saturn/Jupiter-sized planet instead of a Neptune-sized one – the atmospheres of Neptune-like planets might be entirely stripped away if they’re in close orbit around their parent stars, forbidding the existence of hot Neptunes.
The radius gap may also be explicable in this fashion as well, although via a slightly different mechanism. When you’re first forming a planet, if you’re far enough away from your parent star, the type of planet you wind up with depends on how much mass you accrue, as well as how fast you accrue it. Below a certain mass, around ~2 Earth masses, you won’t have enough gravity to hold onto your hydrogen and helium (volatiles) even at substantial distances from the parent star; you cannot become Neptune-like. But above that mass threshold, you could hold onto them at great enough distances, and so only at small distances (and hot temperatures) will you not retain a volatile envelope, and so you’re destined to become either a mini-Neptune or a full-fledged Neptune-like world.
These new observations of WASP-69b show, definitively, how one of the lowest-mass “hot Jupiters” around a relatively nearby star – just 160 light-years away – is actively experiencing photoevaporation. The atmosphere gets inflated by the heat from the parent star, the volatile-rich atmosphere experiences stripping due to the energetic particles and radiation that create outflows, and those volatiles are not only blown off of the host planet itself, but create a comet-like tail behind the planet: perhaps a tail that’s even much longer than what’s observed, as an imperfect alignment between the planet and our line-of-sight to the star could admit such a possibility.
The implications of this observation are staggering, however. They could explain why hot Jupiters (and hot Saturns) exist in great abundances around stars, but that hot Neptunes don’t while solid, planetary cores do again. They are consistent with explaining the radius gap with the notion that either you can successfully hold onto your volatiles, making you Neptune-like, or not, making you Earth-like (or at least a solid-surfaced planet), and that “yes or no” question is why there are precious few exoplanets between 140% and 200% the radius of Earth.
But most importantly, what was only a theoretical scenario now has direct, powerful observational evidence supporting it. Perhaps, with future observations, we’ll definitively solve the “hot Neptune” deficit and the “radius gap” problems once and for all!