The mystery of the Moon’s two faces could soon be solved
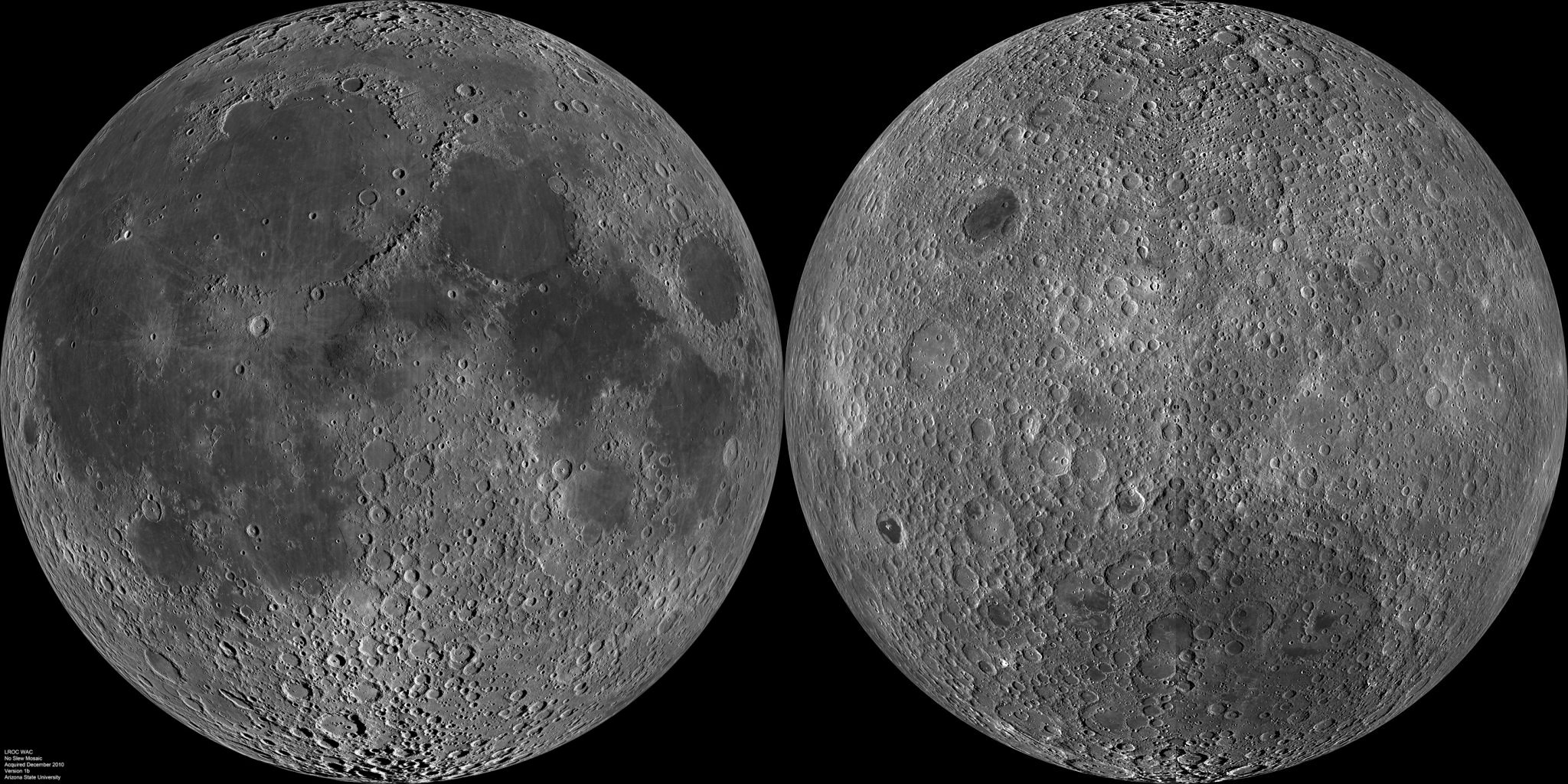
- Although even our pre-human ancestors were familiar with the near side of the Moon, we didn’t get our first glimpse of the lunar far side until 1959, and wow, is it surprisingly different.
- Unlike the near side, it’s practically devoid of lunar maria, and instead is filled with mountainous, high-altitude, heavily cratered highlands. Even in 2024, no one is sure as to why.
- However, humanity’s first successful sample return mission from the Moon’s far side, conducted by China’s Chang’e-6, might solve the mystery. A wild theory is about to be put to the test.
Long ago, something odd must have happened way back when the Moon first formed. We aren’t sure exactly what it was or how it happened, but the aftermath of those events led to a tremendous set of differences between the Moon’s near side — the side that always faces us — and the far side, which can only be accessed via spacecraft. The near side is full of familiar and recognizable features, including:
- heavily cratered white areas, representing old lunar highlands,
- sparsely cratered dark areas, representing lowlands known as lunar maria,
- bright rays emerging from many of the deep lunar craters, but only in the dark maria areas,
- and many craters of different sizes superimposed atop one another, but found almost exclusively in the light highland areas.
But the far side, which was only glimpsed for the first time with a 1959 space mission, is very different. It’s almost exclusively comprised of heavily cratered highlands, and the few dark, low-lying maria that are present are very small in comparison to the near side. The simplest explanation for this effect is already known to be wrong, but a wild-yet-compelling theory is about to be put to the test. Now that China’s Chang’e-6 mission has successfully returned lunar samples taken from the Moon’s far side to Earth for the first time in human history, we can at last analyze them and compare them to near side samples that have been around since the Apollo era. After all these years, the mystery of the Moon’s two hemispheres could finally be solved.
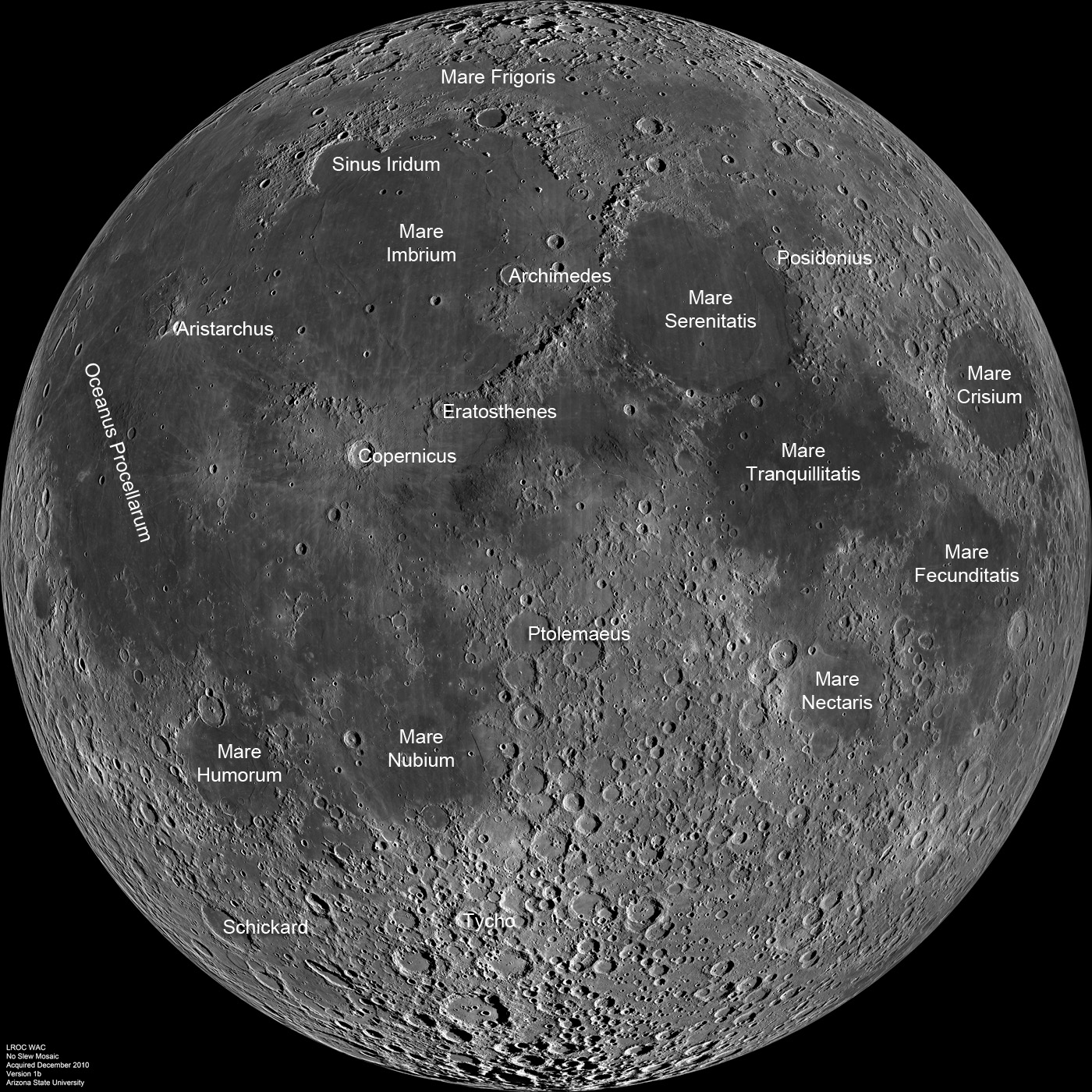
The differences between the lunar near side and the lunar far side are striking, as most of us would struggle to even recognize our own Moon if all we saw were the side that always faces away from Earth. Instead of a large number of dark, lightly cratered maria, there’s only one substantial one: located on the far side’s northern hemisphere. There are no giant mountain ridges, like the one prominently separating Mare Imbrium from Mare Serenitatis on the near side. There are fewer giant craters with enormous rays radiating out from them, like Giordano Bruno and Pierazzo, whereas the near side is rife with them: Copernicus, Aristarchus, Kepler, Tycho, and many more.
But perhaps the biggest difference of all can be seen in terms of elevation. Except for the isolated northern depression, Mare Mascoviense, and the enormous South Pole-Aitken basin — both likely caused by ancient impacts — the far side of the Moon is at a much higher altitude than the near side. Overall, the average elevation of the far side of the Moon is greater than the near side’s average elevation by nearly 2 kilometers, and based on seismic data, the lunar crust on the far side is thicker than the near side crust by approximately 15 kilometers. For some reason, the Moon’s far side is more heavily cratered, at a significantly higher elevation, and possesses a much thicker crust (by ~25% or more) as compared to the Moon’s near side.
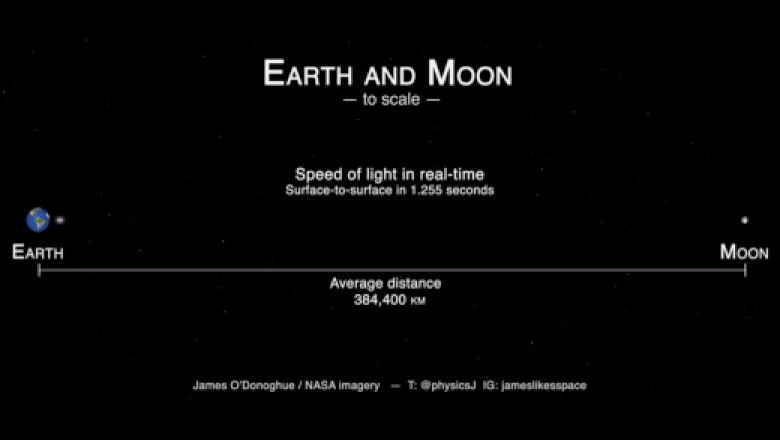
Many of us, upon learning this, have an almost instinctive explanation for why the far side would and should be more heavily cratered than the near side: because the Earth is in the way of the near side. After all, the Moon is relatively close by, at a mean distance of just 380,000 km from Earth, and Earth is much larger (nearly four times the Moon’s diameter) and much more massive (approximately 82 times as massive as the Moon), which should make it a much better target for potential impactors. Because of this, you might be tempted to think that the lunar far side is much more cratered because it lacks the protective effects that the Earth provides: acting like a shield from these impacts for the near side.
Unfortunately, this explanation falls apart completely when we quantify the protective effects and compare them to the observed crater rates. The Earth-Moon distance, although small on scales of the Solar System, is actually some ~30 times greater than the diameter of the Earth, meaning that the cratering difference should be less than ~1%. Despite Earth’s massiveness, the cratering rates on both hemispheres of the Moon should be affected nearly equally by Earth’s gravity, again implying a difference in crater rates between the near side and far side of less than ~1%.
But this is not the case at all. The lunar far side is somewhere around ~30% more heavily cratered than the near side: a tremendous difference that cannot be explained by gravitational deflection or impact absorption. It also fails to explain the other differences: in maria abundance or in elevation and crustal thickness. As tempting as this idea is, it’s long been ruled out.
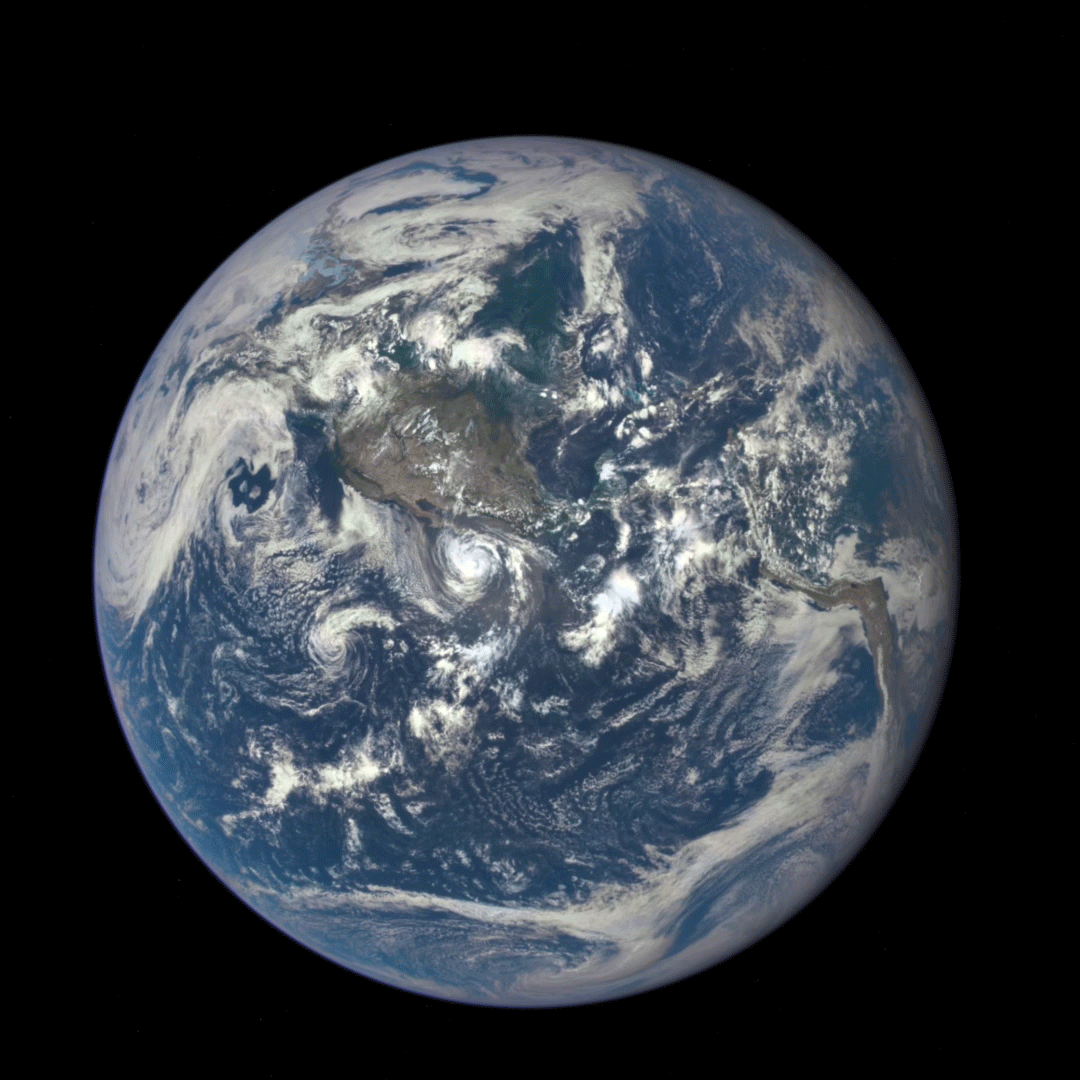
Instead, a better approach is to turn to our picture of how we think the Moon formed in the first place: from a giant impact between proto-Earth and a now-devoured protoplanet known as Theia. You might think of the asteroid impact that wiped out the dinosaurs as a huge one, and by many metrics, it truly was tremendous. At an estimated size of 5-to-10 kilometers across — the size of a large mountain — it created Chicxulub crater: an impact crater that’s about 200 kilometers in diameter.
But if we go back about 4.5 billion years in time, we’d learn there was a much larger impact early on in our planet’s history that makes the Chicxulub impactor look like a mere pebble. Back when the Solar System was in its infancy, the Earth was mostly formed: of almost the same mass and radius that it has today. But there was another large protoplanet nearby, perhaps similar in size to Mars. About 50 million years after the other planets finished forming, proto-Earth and this hypothesized protoplanet, known as Theia, collided with each other.
In the aftermath of this collision, an enormous cloud of debris was kicked up, forming a theoretical structure known as a synestia. While the majority of this mass eventually fell back to Earth or escaped into interplanetary space, enough of it gravitationally coalesced to form our Moon.
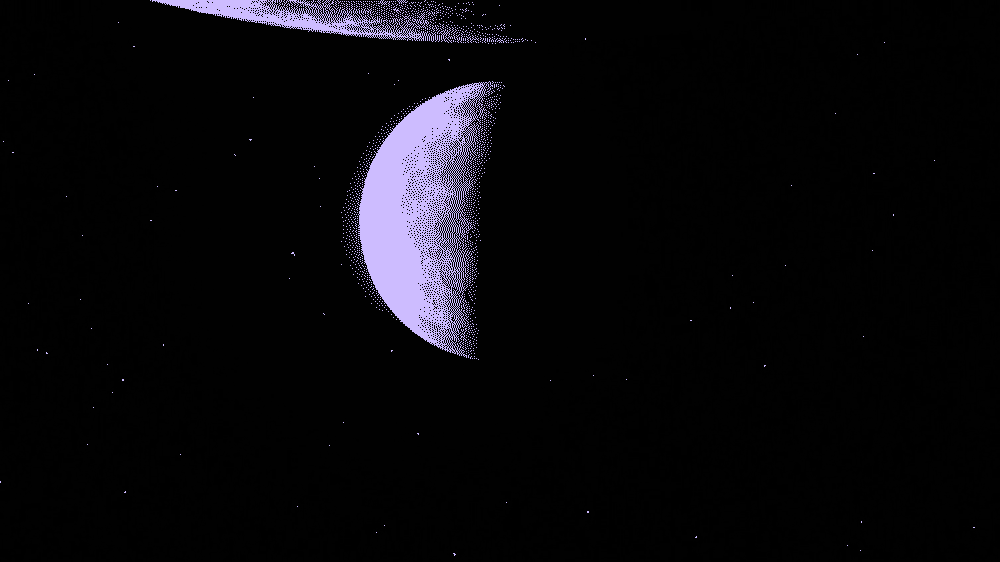
In fact, we now think that giant impacts are the primary way that small, solid planets get their moons, as giant impacts are theorized to be the mechanism behind how Mars got its system of moons, and also how Pluto’s system of moons came to be. Although the giant impact hypothesis was regarded as a fringe idea when it was first proposed in the 1970s, an astounding piece of evidence led to its gradual acceptance: the first lunar sample returns showed that the composition of material from the Moon matched, exactly, the composition of material from the Earth itself. This astonishing fact lent great support to the idea of a giant impact in our distant past, and gave us a new scenario for the creation of the Moon.
So if that’s how and when the Moon first formed, what type of scenario does that set up?
We have to remember that today, the factor that primarily determines the temperature of a planet is the combination of heating from the Sun, atmospheric heat-trapping and planetary radiation, as well as internal processes like gravitational contraction and the decay of radioactive elements in the planet’s interior. But early on, during the early stages of planetary formation, there was a lot of heat left over from the pre-solar nebula that caused these planets to form, and the estimated temperature for the Earth during these early stages was nearly ten times as hot as it is today: somewhere around 2700 K, or the temperature of a traditional warm white light bulb.

We also know, from observations of features that have been imprinted into the Earth over geologic history, that early on, our planet rotated much more quickly about its axis, and — by the laws governing angular momentum — that implies that the Moon was much closer to Earth in the past. Now, let’s put these three pieces of information together:
- the fact that a giant impact created a synestia-like structure,
- which gave rise to a Moon that formed in close proximity to Earth,
- and that the Earth was very hot at this time: around 2700 K.
It’s likely that the Moon, even upon its initial formation, was created already tidally locked to Earth, where one face of the Moon always points toward the Earth and the opposite face always points away, but even if that weren’t the case and the Moon was born rotating, gravitational physics teaches us that it would’ve become tidally locked very quickly: on timescales of ~100,000 years, at most.
Now, picture it: we have a young, hot Earth that’s in close proximity to a tidally locked large Moon, which itself forms from a puffy cloud known as a synestia and may create, as part of the aftermath of the collision, a circumplanetary disk of matter surrounding the Earth. As you might expect, this excess material doesn’t persist forever, but much of it will wind up landing on (and adding to) the Moon, on both the Earth-facing and the away-from-Earth sides.

What would this imply, as far as the similarities and differences between the two faces of the Moon, in such a scenario?
The details of this were only worked out, for the first time, ten years ago, in a paper by Arpita Roy, Jason Wright, and Steinn Sigurdsson. The key realization is that the heat from the young, hot Earth — an extreme version of Earthshine — should have produced not only a temperature gradient affecting the material surrounding the Earth, but also a chemical gradient: where heavier elements that are closer to the hot Earth are more easily vaporized, but those same elements, when farther away, are less easily vaporized.
Elements like calcium and aluminum, in particular, should preferentially be deposited on the lunar far side as compared to the lunar near side, which means we now have a prediction to look for: greater abundances of these easily vaporized elements on the side of the Moon that always faces away from Earth. The authors then went on to show that greater abundances of calcium and aluminum in the more distant part of the Moon-forming structure — either a circumplanetary disk or a synestia (the latter of which wasn’t coined until a couple of years later) — would lead to a thicker crust on the lunar far side as compared to the lunar near side. Because the crust is the least dense part of a world, effectively “floating” atop the interior mantle, that should correspond to greater elevations on the far side as well.

In other words, if this is the creation story for our Earth-Moon system, then there’s now a testable prediction: certain easily-vaporized elements should be more abundant as part of the lunar far side’s crust as opposed to the lunar near side’s crust. If the Moon were formed so that it was perfectly (or nearly-perfectly) tidally locked to Earth, then the near side should be depleted in these elements, while the far side should be abundant in them. On the other hand, if the Moon rotated consistently throughout its formation, then these elements should be deposited the same way char is deposited on a rotisserie chicken: relatively evenly across its surface.
This theory, although it received very little public notice when it was first proposed, should now be of intense interest to planetary scientists, as for the very first time in history, humanity now has the materials necessary to put it to the test. On June 25, 2024, the Chang’e-6 mission successfully returned more than two kilograms of lunar samples from the Moon’s far side: the first rocks from the far side of the Moon ever to be brought back to Earth. Although the Apollo missions (as well as later missions by the Soviet Union and China) returned many lunar samples previously, from both the low-lying maria regions as well as from the heavily cratered lunar highlands, all had been from the Moon’s near side.
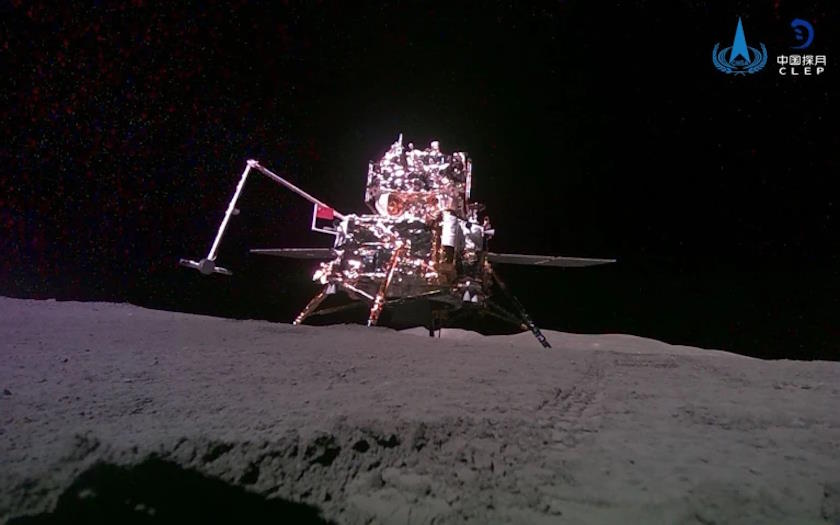
It’s an incredible technical achievement, as there is no way to directly communicate with any spacecraft on the lunar far side from Earth; there is no direct line-of-sight for two-way communication. However, if a far side lander is accompanied by a lunar orbiter, then it becomes possible to communicate directly with the orbiter from Earth, and then have the orbiter communicate with the lander on the surface. Alternatively, the mission could be completely automated, where the lander obeys a pre-defined sequence of events, and then lifts off to dock with a re-entry capsule. Through its series of Chang’e missions, the CNSA has taken extraordinary steps forward on both of these fronts.
The mission first pre-selected a landing site in the South Pole-Aitken basin, and had the lander successfully touch down there. Then, a robotic arm was deployed, along with a drill, to conduct the sample collection procedure. Finally, the cargo was launched on an ascent trajectory to rendezvous with a re-entry capsule in lunar orbit, where it then returned to Earth. Just 47 minutes after the re-entry procedure began — including an atmospheric skip, re-entry itself, and parachute deployment to ensure a (relatively) soft landing — the samples touched down on Earth, where they were quickly located and identified by the recovery team. Humanity’s first-ever samples from the Moon’s far side have successfully been brought back to Earth.
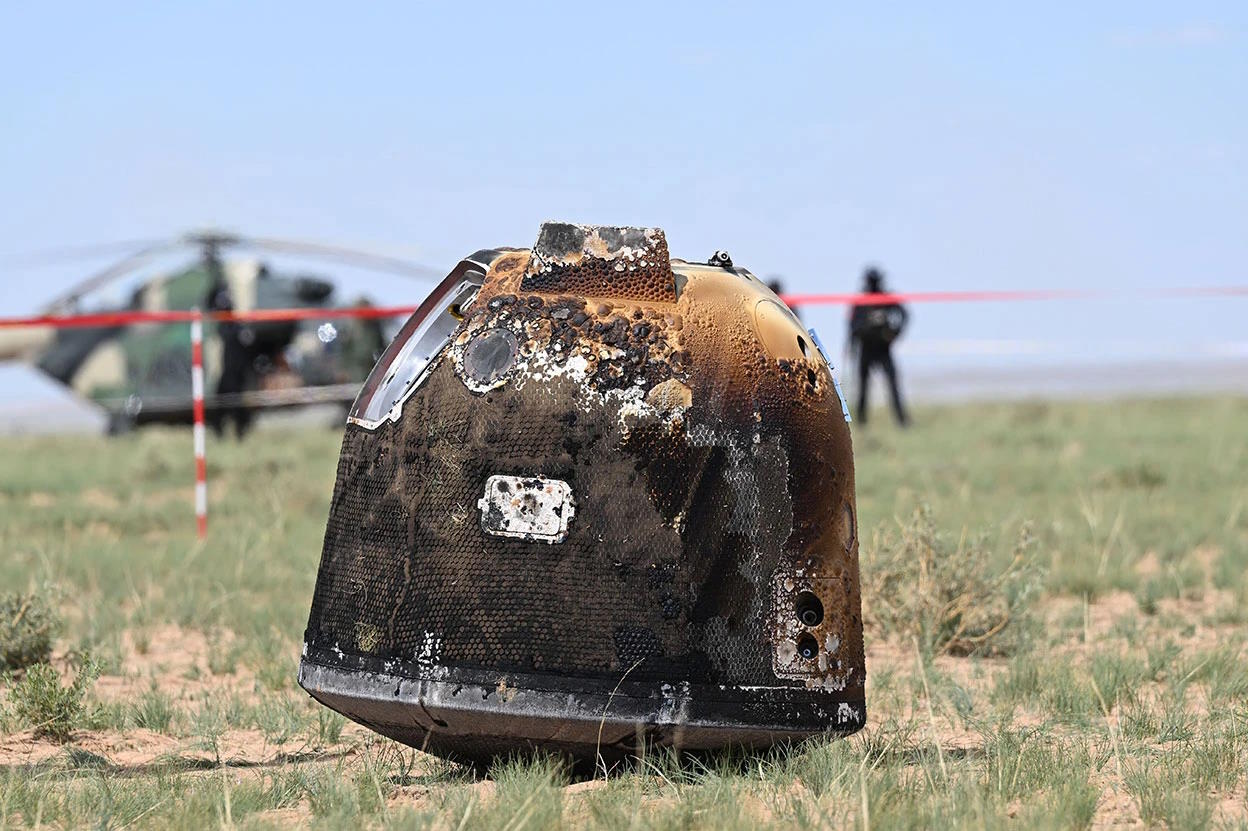
And now, from a scientific point of view, the fun truly begins. What will a sample analysis of the material from the lunar far side show? Will the samples be identical in composition to the ones previously returned: from the lunar near side? Will they be different in any appreciable way, such as in terms of the abundance of various elements? Will they shed any new light on the various formation theories — and details about those theories — for the Moon itself? And will they be consistent with the stories we tell ourselves about the Moon and Earth today, or will there be some novel information that compels us to rewrite what we thought we already knew?
In many ways, this is the beauty of science: laid bare for all to see. We have a few ideas for what we think might have happened, as well as how it happened, all of which are consistent with the evidence we’ve gathered so far. But what will this new evidence reveal? Will it support or refute this exciting vaporization scenario, driven by Earthshine, for the Moon’s far side being higher, thicker, and chemically different than the near side? Will it remain consistent with our ideas about a synestia and a giant impact hypothesis? Or will there be even bigger surprises in store? As we all wait for the analysis to come in and the data to be published, it’s important to look to what the leading thoughts on the Moon’s structure and formation are at present, with the recognition that they’ll soon be put to a novel, critical test. After all, the best way to learn about the Universe is simply to ask it about itself, and listen closely to what its answers reveal.