Not All Particles And Antiparticles Are Either Matter Or Antimatter
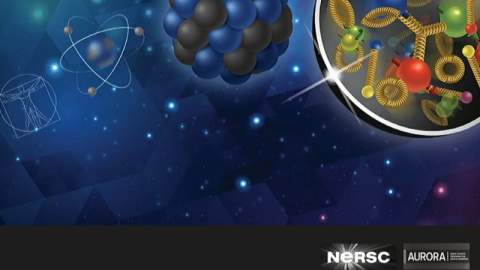
If you think ‘particles are matter’ and ‘antiparticles are antimatter,’ think again.
In this Universe, there are certain rules that have never been observed to be broken. Some of these rules we expect have never been broken. Nothing can move faster than the speed of light; when two quanta interact, energy is always conserved; linear and angular momentum can never be created or destroyed, etc. But some of these rules, even though we’ve never seen them violated, must have been broken at some point in the past.
One such rule is a particular symmetry between matter and antimatter: every interaction that creates or destroys a matter particle also creates or destroys an equal number of their antimatter counterparts, which we typically think of as antiparticles. Given that our Universe is made up almost entirely of matter with virtually no antimatter — there are no antimatter stars, galaxies, or stable cosmic structures in our Universe — clearly this was violated at some point in the past. But how that occurred is a mystery: the puzzle of the matter/antimatter asymmetry remains one of physics’ greatest open questions.
Additionally, we commonly say “particles” to mean things that make up matter, and “antiparticles” to mean things that compose antimatter, but that’s not exactly true. Particles aren’t always matter, and antiparticles aren’t always antimatter. Here’s the science behind this counterintuitive truth about our Universe.
When you think about the material we find here on Earth, you probably think that absolutely 100% of it is made of matter. This is approximately true, as practically our entire planet consists of matter made of protons, neutrons, and electrons, all of which are, in fact, matter particles. Protons and neutrons are composite particles, made of up-and-down quarks which bind together by exchanging gluons to form atomic nuclei. Those atomic nuclei, in turn, have electrons bound to them so that the total electric charge of each atom is zero, with the electrons remaining bound through the electromagnetic force: an exchange of photons.
Every once in a while, however, one of the particles inside an atomic nucleus will undergo a radioactive decay. A typical example is beta decay: where one of the neutrons will decay to a proton, also emitting an electron and an anti-electron neutrino. If we look at the properties of the various particles and antiparticles that participate in this decay process, we can learn a lot about how our Universe works.
The neutron, which we started with, has the following properties:
- it’s electrically neutral, with no net electric charge,
- it’s made of three quarks: two down quarks (each with electric charge -⅓) and one up quark (with electric charge +⅔),
- and it contains a total amount of about 939 MeV of energy, all in the form of its rest mass.
The particles that it decays into, the proton, the electron, and the antielectron neutrino, also have their own unique particle properties.
- The proton has an electric charge of +1, is made of one down quark and two up quarks, and contains about 938 MeV of energy in its rest mass.
- The electron has an electric charge of -1, is a fundamentally indivisible particle, and contains about 0.5 MeV of energy in its rest mass.
- And the anti-electron neutrino has no electric charge, is fundamentally indivisible, and has an unknown but non-zero rest mass that’s no more than about 0.0000001 MeV worth of energy.
All our mandatory conservation rules are intact. Energy is conserved, with the little bit of “extra” energy that was in the neutron getting converted to kinetic energy in the product particles. Momentum is conserved, as the sum of the momenta of the product particles always equals the initial momentum of the neutron. But we don’t just want to examine what we start with and what we wind up with; we want to know how it happens.
For a decay to occur in quantum theory, there has to be a particle that mediates it. In the theory that describes it — the quantum theory of the weak interactions — the particle responsible is the W- boson, which acts on one of the neutron’s down quarks. Think about, in detail, what’s happening here to the fundamental particles.
One of the down quarks in the neutron emits a (virtual) W- boson, causing it to transform into an up quark. The number of quarks is conserved in this part of the interaction.
The (virtual) W- boson could decay into a lot of different things, but is restricted by the conservation of energy: its end products have to be no more energetic than the difference in the rest mass between the neutron and the proton.
Because of this, the primary pathway that occurs is a decay into an electron (to carry the negative charge) and an anti-electron neutrino. On rare occasion, you’ll get what’s known as a radiative decay, where an additional photon is produced. You could, in principle, have a W- boson decay into a quark-antiquark combination (like a down quark and an anti-up quark), but that requires too much energy: more energy than is available during a neutron decaying into a proton plus additional products.
Now, let’s flip the script: from matter to antimatter. Instead of a neutron decaying, let’s imagine we’ve got an anti-neutron decaying instead. An anti-neutron has very similar properties to the neutron we mentioned earlier, but with some key differences:
- it’s electrically neutral, with no net electric charge,
- it’s made of three antiquarks: two anti-down quarks (each with electric charge +⅓) and one anti-up quark (with electric charge -⅔),
- and it contains a total amount of about 939 MeV of energy, all in the form of its rest mass.
All we did, to go from matter to antimatter, was replace all of the particles in play with their antiparticle counterparts. Their masses remained the same, their composition (except for the “anti” part) remained the same, but the electric charge of everything flipped. Even though both the neutron and the anti-neutron are electrically neutral, their individual components flipped sign.
This is measurable, by the way! Even though it’s neutral, the neutron has what’s known as a magnetic moment: something that requires both spin and electric charge. We’ve been able to measure its magnetic moment to be -1.91 Bohr magnetons, and similarly, the magnetic moment of the anti-neutron is +1.91 Bohr magnetons. The “charged stuff” inside of it, that makes it up, must be the exact opposite for antimatter as it is for matter.
When it decays, an anti-down quark emits a W+ boson, the antimatter counterpart of the W- boson, transforming the anti-down quark into an anti-up quark. Just as before, the W+ boson is virtual — meaning it’s unobservable, as there isn’t enough available mass/energy to create a “real” one — but its decay products are visible: a positron and an electron neutrino. (And yes, you can have radiative effects too, where a small fraction of the time, one or more photons join those decay products.) Everything is flipped from before, where every matter particle is replaced with its antimatter counterpart, and every antimatter particle (like the anti-electron neutrino) is replaced with its matter counterpart.
When you think about what we have here on Earth, almost everything is made of matter: protons, neutrons, and electrons. A small fraction of those neutrons are decaying, meaning that we also have W- bosons, additional protons and electrons (and photons), and a few anti-electron neutrinos. Everything that we know of is described extremely well by the Standard Model, with nothing more than the particles and antiparticles we know of required to describe them.
If we swapped out Earth for an imagined antimatter version of ourselves, an “anti-Earth” of sorts, we could just exchange every particle for its antiparticle counterpart. Instead of protons and neutrons (made of quarks and gluons), we’d have antiprotons and antineutrons (made of antiquarks, but still those same 8 gluons). Instead of a neutron decaying through a W- boson, we’d have an antineutron decaying through a W+ boson. Instead of producing an electron and an anti-electron neutrino (and sometimes a photon), you produce a positron and an electron neutrino (and sometimes a photon).
The particles that make up the normal matter in our Universe are the quarks and leptons: the quarks make up protons and neutrons (and baryons, in general), while the leptons include the electron and its heavier cousins, as well as the three regular neutrinos. On the flipside, there are antiparticles that make up the antimatter that exists in our Universe: the antiquarks and antileptons. Through natural decays that involve a number of pathways that leverage both W- and W+ bosons, there’s a tiny bit of antimatter in the form of positrons and anti-electron neutrinos. This would persist even if we somehow manage to “turn off” the outside Universe, including the Sun, cosmic rays, and any other sources of particles or energy.
But what about the other particles and antiparticles? When we talk about matter and antimatter, we’re talking only about the fermions in our Universe: the quarks and leptons. But there are bosons as well:
- the 1 photon, which mediates the electromagnetic force,
- the 8 gluons, which mediate the strong nuclear force,
- the 3 weak bosons, the W+, W-, and Z⁰, which mediate the weak force and weak decays,
- and the Higgs boson, which is entirely unique when compared to the others.
Some of these particles are their own antiparticles, like the photon, the Z0, and the Higgs. The W+ is the antiparticle counterpart of the W-, and you can match up three pairs of gluons as clearly being the antiparticle counterparts of one another. (The gluons are a little complicated when it comes to the fourth pair.)
If you collide a particle with its antiparticle counterpart, they annihilate away, and can produce anything that’s energetically allowed, so long as all the quantum conservation rules — energy, momentum, angular momentum, electric charge, baryon number, lepton number, lepton family number, etc. — are all obeyed. This includes particles that are their own particles, just as equally as particles that have distinct antiparticle counterparts.
What’s remarkable about this is where the idea of “matter” versus “antimatter” comes in. If you have a positive baryon or lepton number, you’re matter. If you have a negative baryon or lepton number, you’re antimatter. And if you don’t have either baryon or lepton number… well, you’re neither matter nor antimatter! Even though there are two types of particles — fermions (which include quarks and leptons) and bosons (which include everything else) — it’s only the fermions in our Universe that can be either matter (for the normal fermions) or antimatter (for the anti-fermions).
(Note that if neutrinos turn out to be Majorana fermions, this will need to be revised, as Majorana fermions can indeed be their own antiparticle.)
That means composite particles, like pions or other mesons that are made of quark-antiquark combinations, are neither matter nor antimatter; they’re equal amounts of both. Positronium, which is an electron and a positron bound together, is neither matter nor antimatter. If leptoquarks or the superheavy X or Y bosons that arise in Grand Unified Theories exist, they’d be examples of hypothetical particles with both baryon and lepton numbers; there would be both matter and antimatter versions of them. And it means that, if supersymmetry were correct, we could have fermions like the supersymmetric counterpart of the photon — the photino — that are neither matter nor antimatter. Possibly, we could even have supersymmetric bosons, like squarks, whose particle and antiparticle versions really are matter and antimatter.
It’s such a simple idea to think that there are particles in our Universe, and that’s what matter is, and that the antiparticle counterparts of these particles would make up antimatter. This is partly true, as if we chopped up the particles that exist in our Universe, most of them would be made of constituent particles that we consider matter. Similarly, if we swapped all of those particles for their antiparticle counterparts, we’d wind up with what we consider antimatter. This works for every quark (with baryon number +⅓ each), every lepton (with lepton number +1 each), as well as every antiquark (with baryon number -⅓ each) and every antilepton (with lepton number -1 each).
But everything else in the Universe — all of the bosons, which carry neither lepton nor baryon number, and all of the composite particles with a net baryon and lepton number of zero — lives in a nebulous area where they’re neither matter nor antimatter. It isn’t fair to designate one type as a “particle” and another type as an “antiparticle” in this case. Sure, W+ and W- might annihilate just like all particle-antiparticle pairs do, but neither one has any more of a claim to be “matter” or “antimatter” than any other boson, which is to say, they have no claim to that status. Asking “which one is matter and which one is antimatter” has no meaning; they’re simply one another’s antiparticle, with neither one having properties of matter or antimatter at all.
Starts With A Bang is written by Ethan Siegel, Ph.D., author of Beyond The Galaxy, and Treknology: The Science of Star Trek from Tricorders to Warp Drive.