How quantum levitation works
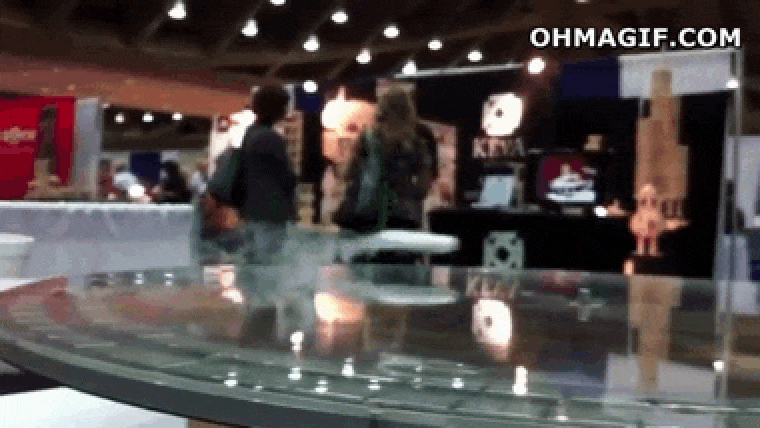
- In our conventional world, if you apply a voltage to any system of charged particles, it will cause them to move, creating a current, but whatever the resistance of the material they’re passing through is will resist that motion.
- However, under certain low-temperature conditions in certain specific materials, the resistance can drop to zero, creating a “lossless” medium for electricity to flow through: a superconductor.
- By leveraging the properties of certain superconducting materials with impurities within them, a properly configured magnetic setup can lead to quantum levitation, just like you see here!
The idea of levitating off the ground has been a staple of science fiction dreams and human imagination since time immemorial. While we don’t have our hoverboards just yet, we do have the very real phenomenon of quantum levitation, which is almost as good. Under the right circumstances, a specially-made material can be cooled down to low temperatures and placed over a properly-configured magnet, and it will levitate there indefinitely. If you make a magnetic track, it will hover above or below it and remain in motion perpetually.
But isn’t perpetual motion supposed to be an impossibility in physics? It’s true that you can’t violate the law of conservation of energy, but you very much can make the resistive forces in any physical system as small as possible. In the case of superconductivity, a special set of quantum effects really does enable the resistance to drop all the way to zero, enabling all sorts of strange phenomena, including the one you see below: quantum levitation. Here’s the physics of how it works.
This now-eleven-year-old video is still shocking to many who see it, even the second, third, or hundredth time. A number of things, even if you don’t look closely, are already apparent:
- the special material that levitates is extremely cold,
- it can levitate either above or below a magnet: it gets pinned in a certain location,
- and if you put it on a magnetic track, it doesn’t lose any speed over time.
This is really counterintuitive stuff and isn’t the way that conventional, classical physics works. The permanent magnets you’re used to — which physicists call ferromagnets — could never levitate like this. Let’s take a look at how those work, and then see how this levitating phenomenon is different.

Every material we know of is made up of atoms, which themselves may or may not be bound into molecules as part of the material’s internal structure. When you apply an external magnetic field to that material, those atoms-or-molecules get internally magnetized as well, and line up in the same direction as the external magnetic field.
The special property of a ferromagnet is that when you take the external magnetic field away, the internal magnetization remains. That’s what makes it a permanent magnet.
Although this is the type of magnet we’re most familiar with, nearly all materials are not ferromagnetic. Most materials, once you take the external field away, go back to being unmagnetized.
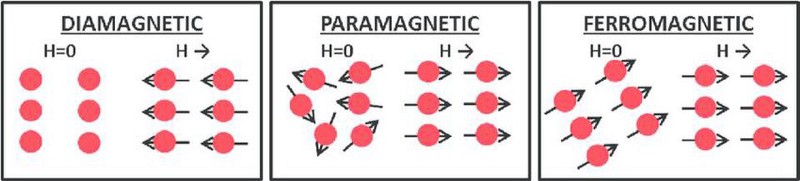
So what happens inside these non-ferromagnetic materials when you apply an external magnetic field? Overall, such materials are either:
- diamagnetic, where they magnetize anti-parallel to the external field,
- or paramagnetic, where they magnetize parallel to the external field.
As it turns out, all materials exhibit diamagnetism, but some materials are either also paramagnetic or ferromagnetic. Diamagnetism is always weak, so if your material is paramagnetic or ferromagnetic also, that effect can easily overwhelm the effect of diamagnetism.
So when you turn an external field on or off — which is the same thing, physically, as moving a material closer to or farther away from a permanent magnet — you change the magnetization inside the material. And there’s a physical law for what happens when you change the magnetic field inside a conducting material: Faraday’s Law of Induction.
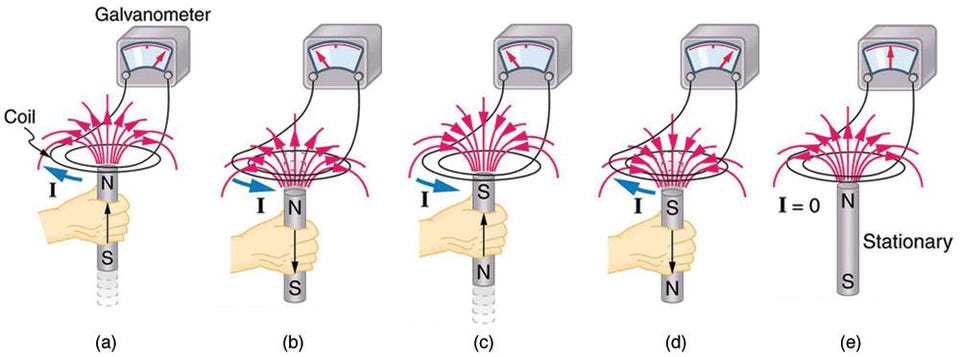
This law tells you that changing the field inside a conducting material causes it to generate an internal electric current. These little currents you generate are known as eddy currents, and they oppose the internal change in the magnetic field. At normal temperatures, these currents are extremely temporary, as they encounter resistance and decay away.
Now, at normal temperatures, the eddy currents created inside are extremely temporary, as they encounter resistance and decay away.
But what if you eliminated the resistance? What if you drove it down all the way to zero?
Believe it or not, you can drive the resistance down to zero in pretty much any material; all you have to do is bring it down to low enough temperatures, until it becomes a superconductor!
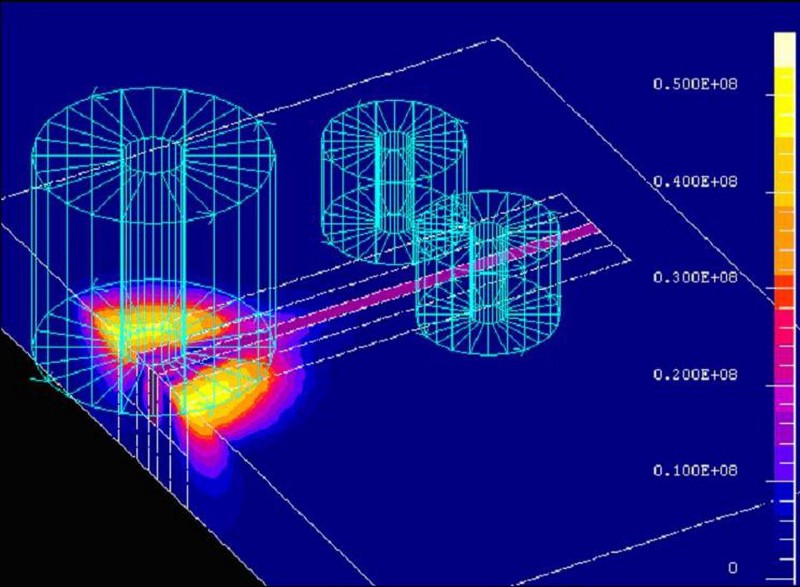
These levitating materials are indeed made of specific materials that superconduct — or have their resistance drop to zero — at very low temperatures. In principle, any conducting material can be made to superconduct at low-enough temperatures, but what makes these particular superconductors interesting is that they can do it at 77 K: the temperature of liquid nitrogen! These relatively high critical temperatures make it easy to create a low-cost superconductor.
Every material has a critical temperature (labelled Tc, below), and when you cool that material below its critical temperature, it no longer has any resistance to electrical current at all. But just what is it that happens when you drop the temperature of a material below its critical temperature, to make it superconducting? It expels all the magnetic fields from inside! This is known as the Meissner Effect, and it turns a superconducting material into a perfect diamagnet.
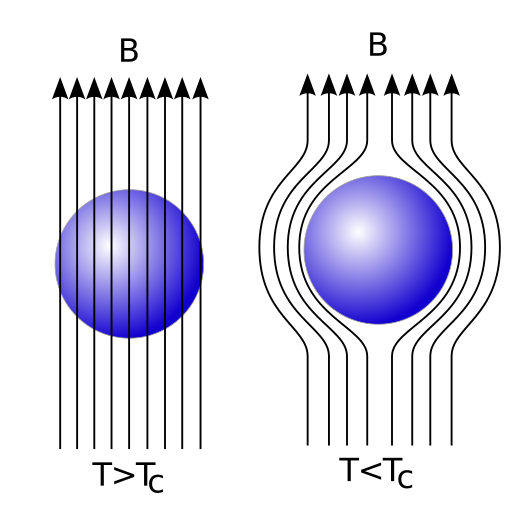
Materials like aluminum, lead, or mercury behave as a superconductor exactly in this fashion when you cool them down below their critical temperatures, expelling all internal magnetic fields. But most superconducting materials will superconduct at higher, more accessible temperatures if you mix multiple types of atoms together to create various compounds, and those compounds can have different properties at different locations within the material.
This allows us to go a step farther than simply creating a superconductor.
Instead of a uniform, perfect diamagnet, let’s imagine we have one with impurities inside of it. If you then cool your material down below the critical temperature and change the magnetic field inside of it, those interior magnetic fields still get expelled, but with an exception. Anywhere you have an impurity, the field remains. And because it cannot enter the expelled region, those field lined get pinned inside the impure regions.
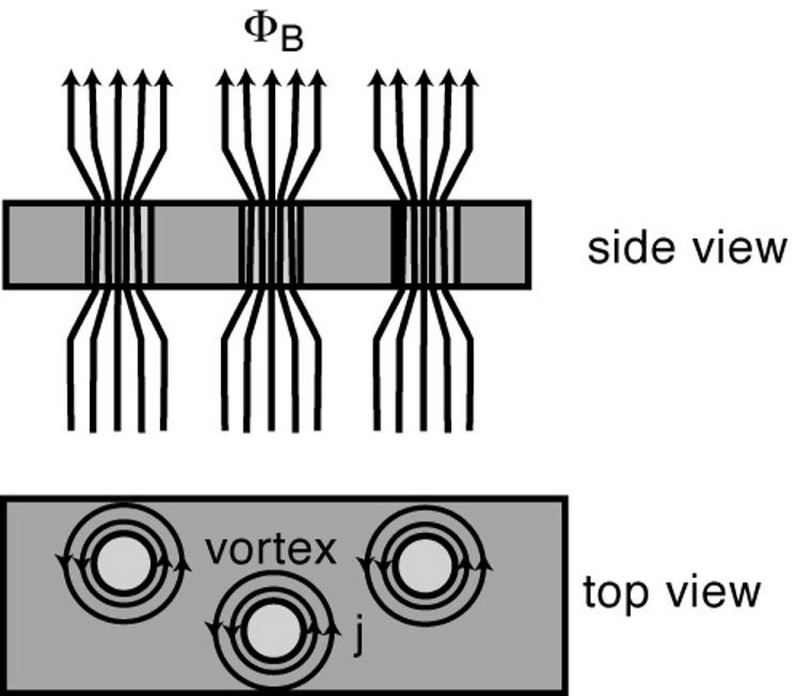
The impurities are the key to making this phenomenon of magnetic quantum levitation happen. The magnetic field gets expelled from the pure regions, which superconduct. But the field lines penetrate the impurities, which changes the field inside and creates those eddy currents.
And this is where the key lies: those eddy currents are moving electric charges, which encounter no resistance because the material is superconducting!
So instead of the currents decaying away, they’re sustained indefinitely, for as long as the material remains superconducting and at temperatures below the critical one.
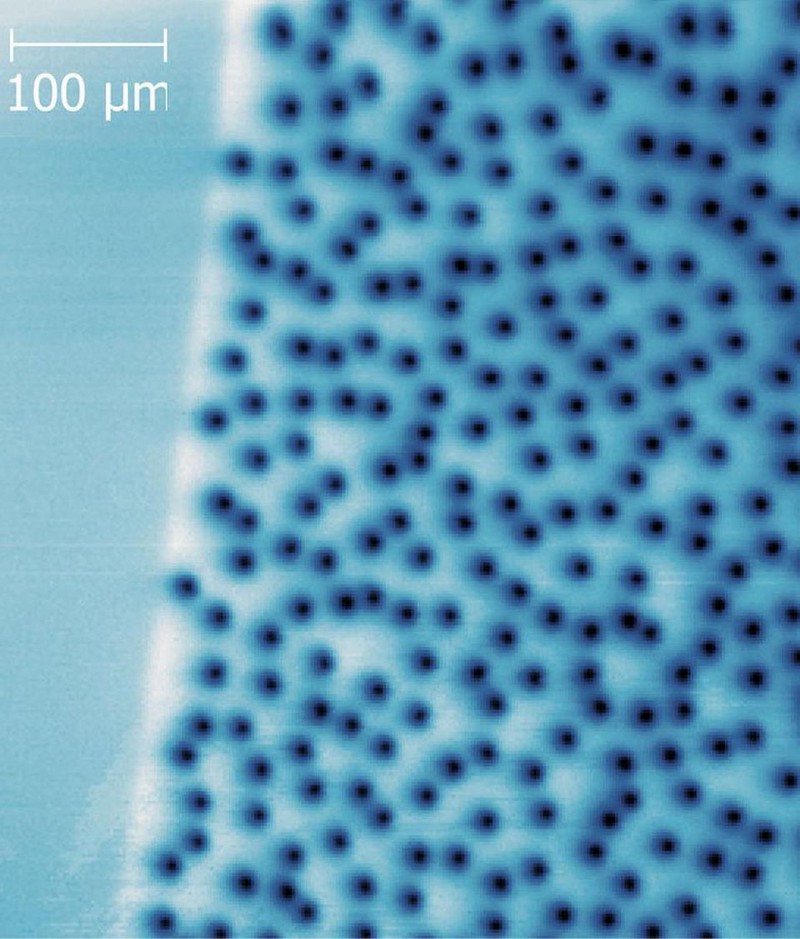
Overall, we have two separate things happening in the two different regions:
- In the pure, superconducting regions, the fields are expelled, giving you a perfect diamagnet.
- In the impure regions, magnetic field lines get concentrated and pinned, passing through them and causing sustained eddy currents.
It’s the currents generated by these impure regions that pin the superconductor in place, and create the levitating effect! Strong-enough external magnetic fields can destroy the effects, but there are two types of superconductors. In Type I superconductors, increasing the field strength destroys superconductivity everywhere. But in Type II superconductors, superconductivity only gets destroyed in the impure region. Because there are still regions where the field gets expelled, Type II superconductors can experience this levitation phenomenon.
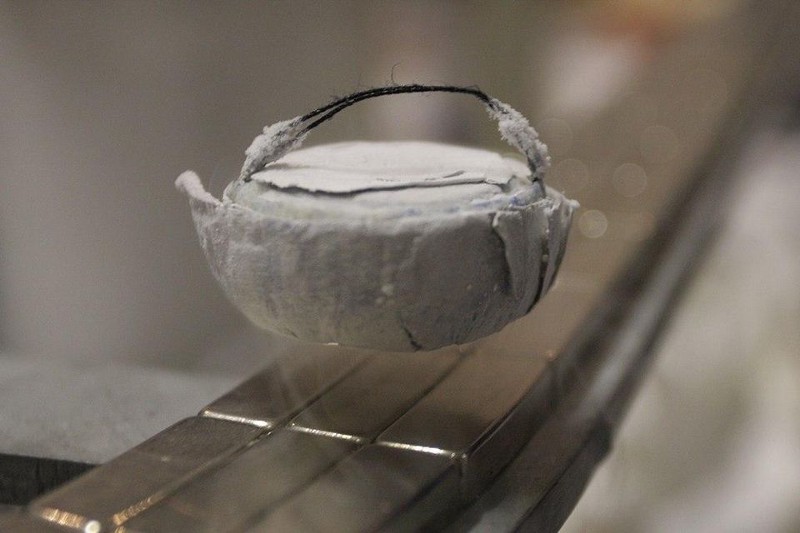
So long as you have that external magnetic field, which is conventionally provided by a series of well-placed permanent magnets, your superconductor will continue to levitate. In practice, the only thing that brings the effect of magnetic, quantum levitation to an end is when the temperature of your material rises back up above that critical temperature.
This gives us an incredible holy grail to aim for: if we can create a material that superconducts at room temperature, then it will remain in this levitating state indefinitely.
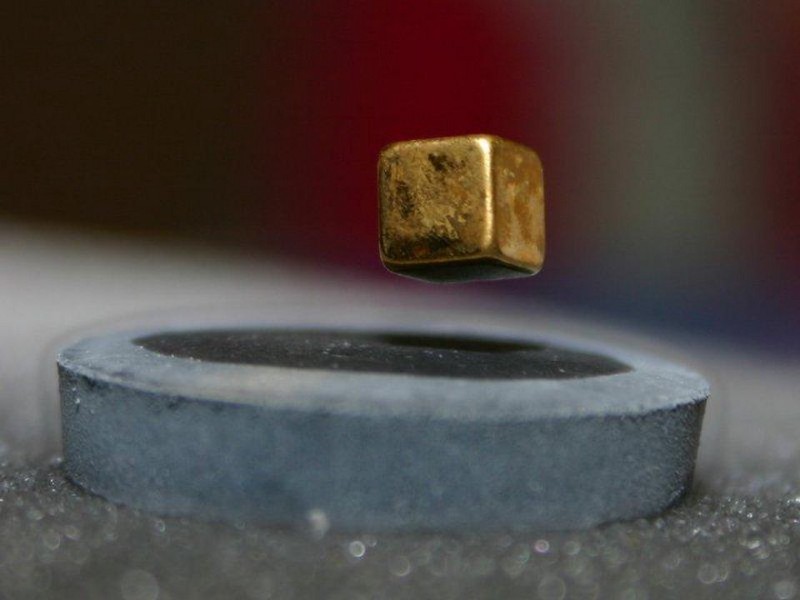
If we designed-and-built a magnetic track for it, made this impurity-laden superconductor, brought it to room temperature, and started it in motion, it would remain in motion without bound. If we did this in a vacuum chamber, removing all air resistance, we would literally create a perpetual motion machine: a device that can continue in motion, forever, without losing any energy as it continues to move.
What does all of this mean? That levitation is actually real, and has been achieved here on Earth. We could never do this without the quantum effects that enable superconductivity, but with them, it’s merely a question of designing the right experimental setup.
It also gives us a tremendous sci-fi dream for the future. Imagine roads made out of these properly-configured magnetic tracks. Imagine pods, vehicles, or even shoes with the right type of room-temperature superconductors in them. And imagine coasting along at the same speed without ever needing to use a drop of fuel until it’s time to slow down.
If we can develop room-temperature, normal-pressure, Type II superconductors, all of this could become a reality. If you start at absolute zero, or 0 K on the Kelvin temperature scale, we’ve made it more than halfway there toward room-temperature superconductors at atmospheric pressure. Science has the potential to truly bring this “holy grail” of low-temperature physics into reality in the very near future.