The failed experiment that changed the world
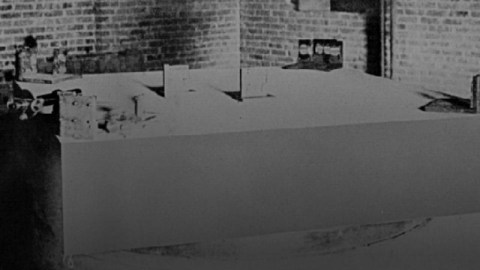
Sometimes, designing a careful experiment and measuring absolutely no effect can be the most important result of all.
“It appears, from all that precedes, reasonably certain that if there be any relative motion between the earth and the luminiferous ether, it must be small; quite small enough entirely to refute Fresnel’s explanation of aberration.” –Albert A. Michelson
In science, we don’t simply perform experiments willy-nilly. We don’t put things together at random and ask, “what happens if I do this?” We examine the phenomena that exist, the predictions our theories make, and look for ways to test them in ever-greater detail. Sometimes, they give extraordinary agreement to new precision, confirming what we had thought. Sometimes, they disagree, pointing the way to new physics. And sometimes, they fail to give any non-zero result at all. In the 1880s, an incredibly precise experiment failed in exactly this fashion, and paved the way for relativity and quantum mechanics in doing so.
Let’s go even farther back in history to understand why this was such a big deal. Gravitation was the first of the forces to be understood, as Newton had put forth his law of universal gravitation in the 1600s, explaining both the motions of bodies on Earth and in space. A few decades later (in 1704) Newton also put forth a theory of light — the corpuscular theory — that stated that light was made up of particles, that these particles are rigid and weightless, and that they move in a straight line unless something causes them to reflect, refract or diffract.
This accounted for a lot of observed phenomena, including the realization that white light was the combination of all other colors of light. But as time went on, many experiments revealed the wave nature of light, an alternative explanation from Christiaan Huygens, one of Newton’s contemporaries.
Huygens proposed instead that every point which can be considered a source of light, including from a light wave simply traveling forward, acted like a wave, with a spherical wavefront emanating from each of those points. Although many experiments would give the same results whether you took Newton’s approach or Huygens’ approach, there were a few that took place beginning in 1799 that really began to show how powerful the wave theory was.
By isolating different colors of light and passing them through either single slits, double slits or diffraction gratings, scientists were able to observe patterns that could only be produced if light was a wave. Indeed, the patterns produced — with peaks and troughs — mirrored that of well-known waves, like water waves.
But water waves — as it was well-known — traveled through the medium of water. Take away the water, and there’d be no wave! This was true of all known wave phenomena: sound, which is a compression and rarefaction, needs a medium to travel through as well. If you take away all matter, there’s no medium for sound to travel through, and hence why they say, “In space, no one can hear you scream.”
So, then, the reasoning went, if light is a wave — albeit, as Maxwell demonstrated in the 1860s, an electromagnetic wave — it, too, must have a medium that it travels through. Although no one could measure this medium, it was given a name: the luminiferous aether.
Sounds like a silly idea now, doesn’t it? But it wasn’t a bad idea at all. In fact, it had all the hallmarks of a great scientific idea, because it not only built upon the science that had been established previously, but this idea made new predictions that were testable! Let me explain by using an analogy: the water in a rapidly moving river.
Imagine that you throw a rock into a raging river, and watch the waves that it makes. If you follow the ripples of the wave towards the banks, perpendicular to the direction of the current, the wave will move at a particular speed.
But what if you watch the wave move upstream? It’s going to move more slowly, because the medium that the wave is traveling through, the water, is moving! And if you watch the wave move downstream, it’ll move more quickly, again because the medium is moving.
Even though the luminiferous aether had never been detected or measured, there was an ingenious experiment devised by Albert A. Michelson that applied this same principle to light.
You see, even though we didn’t know exactly how the aether was oriented in space, what its direction was or how it was flowing, or what was at rest with respect to it, presumably — like Newtonian space — it was absolute. It existed independently of matter, as it must considering that light could travel where sound could not: in a vacuum.
So, in principle, if you measured the speed at which light moved when the Earth was moving “upstream” or “downstream” (or perpendicular to the aether’s “stream”, for that matter), you could not only detect the existence of the aether, you could determine what the rest frame of the Universe was! Unfortunately, the speed of light is something like 186,282 miles-per-second (Michelson knew it to be 186,350 ± 30 miles-per-second), while the Earth’s orbital speed is only about 18.5 miles-per-second, something we weren’t good enough to measure in the 1880s.
But Michelson had a trick up his sleeve.
In 1881, Michelson developed and designed what’s now known as a Michelson interferometer, which was absolutely brilliant. What it did was built on the fact that light — being made of waves — interferes with itself. And in particular, if he took a light wave, split it into two components that were perpendicular to one another (and hence, moving differently with respect to the aether), and had the two beams travel exactly identical distances and then reflect them back towards one another, he would observe a shift in the interference pattern generated by them!
You see, if the entire apparatus was stationary with respect to the aether, there would be no shift in the interference pattern they made, but if it moves at all in one direction more than the other, you would get a shift.
Michelson’s original design was unable to detect any shift, but with an arm length of just 1.2 meters, his expected shift of 0.04 fringes was just above the limit of what he could detect, which was about 0.02 fringes. There were also alternatives to the idea that the aether was purely stationary — such as the idea that it was dragged by the Earth (although it couldn’t be completely, because of observations of how stellar aberration worked) — so he performed the experiment at multiple times throughout the day, as the rotating Earth would have to be oriented at different angles with respect to the aether.
The null result was interesting, but not completely convincing. Over the subsequent six years, he designed an interferometer 10 times as large (and hence, ten times as precise) with Edward Morley, and the two of them in 1887 performed what’s now known as the Michelson-Morley experiment. They expected a fringe-shift throughout the day of up to 0.4 fringes, with an accuracy down to 0.01 fringes.
Thanks to the internet, here are the original 1887 results!
This null result — the fact that there was no luminiferous aether — was actually a huge advance for modern science, as it meant that light must have been inherently different from all other waves that we knew of. The resolution came 18 years later, when Einstein’s theory of special relativity came along. And with it, we gained the recognition that the speed of light was a universal constant in all reference frames, that there was no absolute space or absolute time, and — finally — that light needed nothing more than space and time to travel through.
The experiment — and Michelson’s body of work — was so revolutionary that he became the only person in history to have won a Nobel Prize for a very precise non-discovery of anything. The experiment itself may have been a complete failure, but what we learned from it was a greater boon to humanity and our understanding of the Universe than any success would have been!
Starts With A Bang is based at Forbes, republished on Medium thanks to our Patreon supporters. Order Ethan’s first book, Beyond The Galaxy, and pre-order his next, Treknology: The Science of Star Trek from Tricorders to Warp Drive!