We Just Measured All The Starlight In The Universe, And It Spells Doom For Our Future
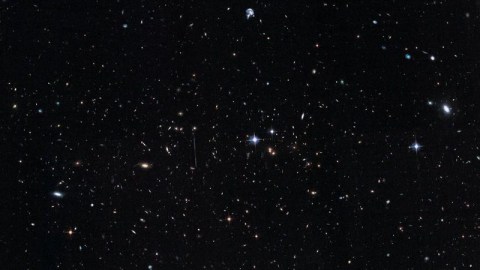
The Universe has been making stars for nearly the entire 13.8 billion years of its history. Here’s what we know.
It’s been 13.8 billion years since the hot Big Bang, and the Universe has come a long way in that time. Our cosmic vision extends for some 46.1 billion light-years in all directions, revealing some 2 trillion galaxies in the process. Each galaxy, on average, contains hundreds of billions of stars, while each star is made of perhaps some 10⁵⁷ atoms. There’s a lot that’s happened in our Universe, but most of it — including the formation of most stars — is a part of our cosmic past, not our present or future.
Thanks to a clever new method developed by scientists working on the Fermi gamma-ray telescope, we’ve been able to measure the star-formation history of the entire Universe throughout all of time. What we arrive at is a startling confirmation of our worst fears: the Universe is dying, and there’s nothing we can do.
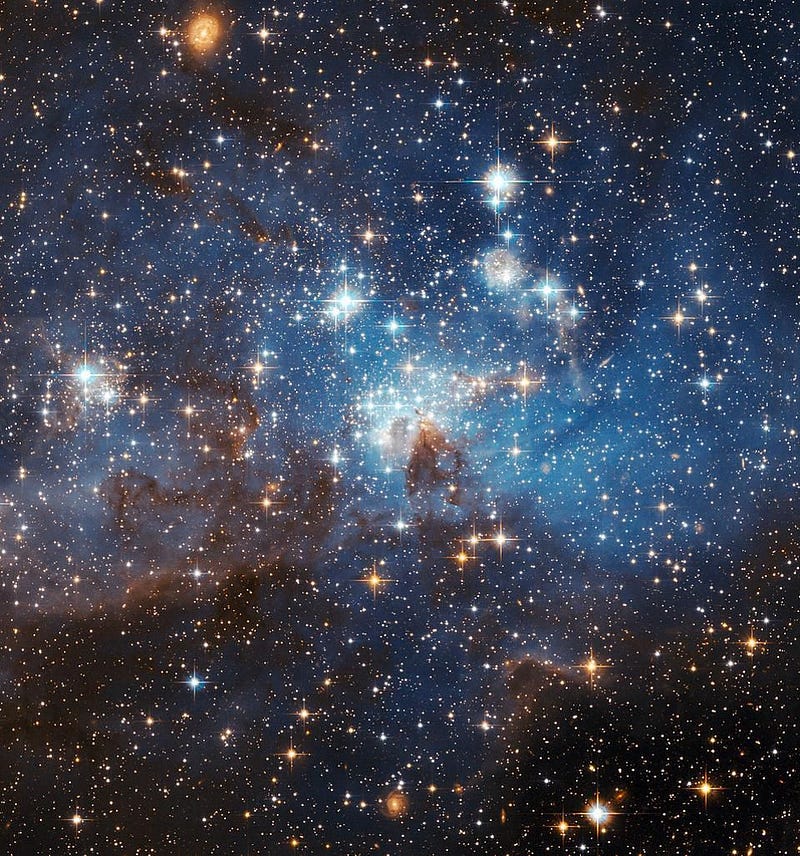
When you form stars, a lot of interesting things happen.
- The molecular cloud that collapses to form them gets ionized by the ultraviolet light produced by these new stars.
- A special type of radiation appears: emission lines, as electrons fall back onto the ionized atomic nuclei.
- This starlight travels through the Universe, interacting with all the atoms they encounter, resulting in an absorption signature.
- And the light has a probability of interacting with gamma-rays, which are the highest energy photons, to produce new particles: electron-positron pairs.
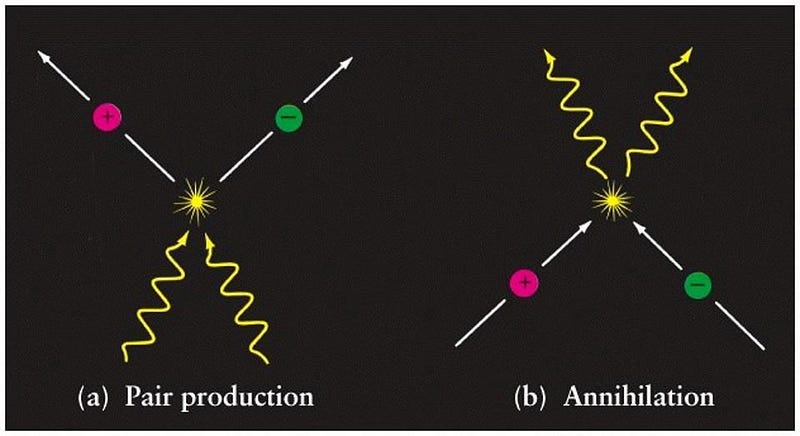
This last point is of particular interest to anyone with a space-based gamma-ray telescope. There are classes of objects in the Universe — active, supermassive black holes — that are very good emitters of extremely energetic particles, including gamma-rays. With enormous event horizons and large, massive accretion disks surrounding them and infalling onto them as they feed, these charged particles generate tremendous magnetic fields as they rotate. These fields accelerate the charged particles, causing them to interact and emit radiation of extremely high energies.
The brightest ones of all, as far as our viewpoint here on Earth is concerned, are the ones whose relativistic jets are pointed right at us. These objects are known as Blazars, because they “blaze” down the line-of-sight right towards your eyes.
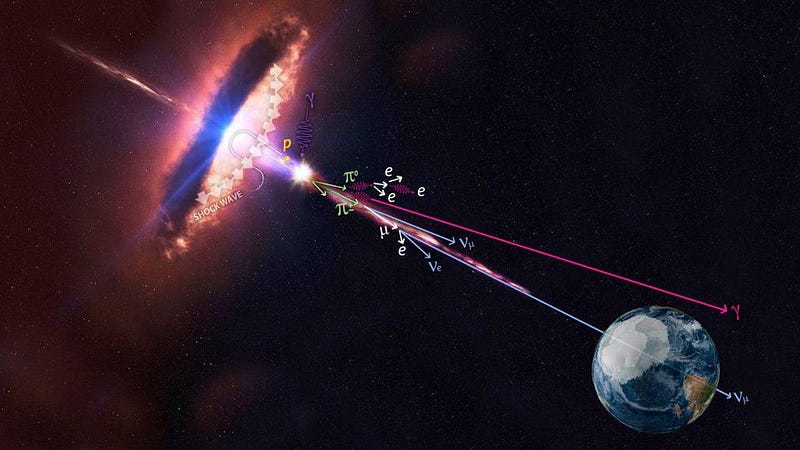
There’s also “stuff” in the way whenever you look at anything in the distant Universe. Gas clouds exist, absorbing a fraction of the light; we can account for those by examining absorption lines. Galaxies and clusters of galaxies often intervene; we can measure their brightnesses, densities, and other properties to calibrate each individual Blazar we examine. Blazars will also be located all over the sky, where zodiacal effects from the Solar System and foreground effects from the Milky Way can affect what we see. And each individual Blazar, at the source, will have properties of energy and flux that are intrinsically unique to it.
By doing the proper accounting of what exists in the Universe — at the source, along the line-of-sight, and received at our eyes — we can determine the source properties of the Blazar we’re examining. We can have a well-calibrated starting point to work from.
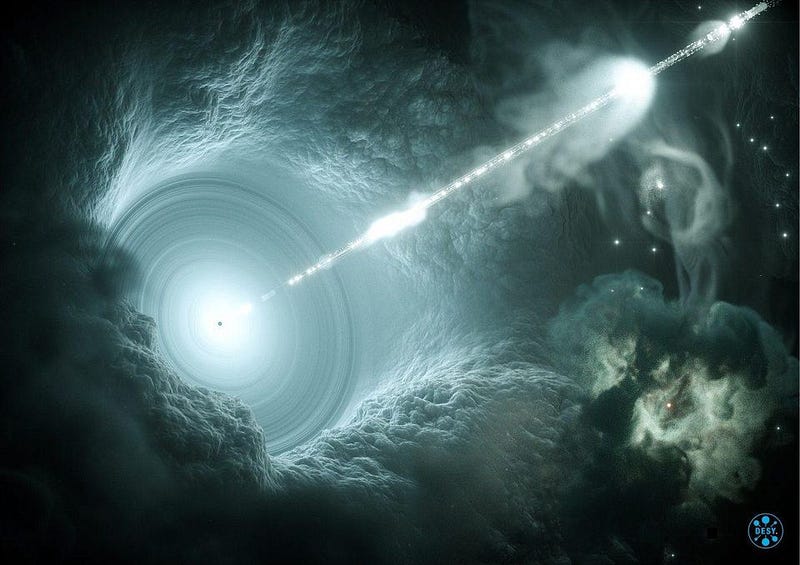
If you had a gamma-ray telescope, this would give you a method for measuring all the starlight in the Universe. Here’s how you’d do it:
- Start by measuring all the blazars everywhere in the Universe that you find them.
- Measure the redshift of each blazar, so you know how far away it lies from you.
- Measure the number of gamma-rays received by your gamma-ray telescope as a function of redshift and blazar brightness.
- And finally, because you know that gamma-rays, when they collide with this extragalactic, background starlight, can produce electron-positron pairs, use all of this information to calculate how much background starlight must be present, as a function of redshift/distance, to account for the loss of gamma rays.
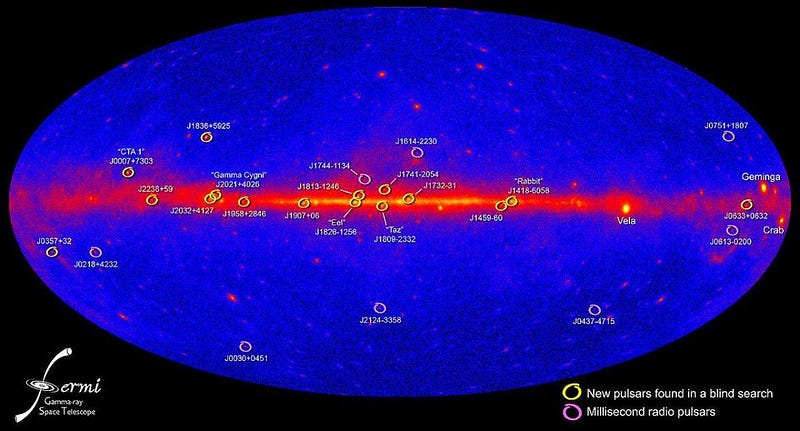
All told, the Fermi-LAT collaboration (where LAT is the Large Area Telescope instrument aboard Fermi) was able to make these measurements for all the known Blazars appearing in the gamma-ray sky: 739 of them. The closest one comes to us from just 200 million years ago; the most distant has its light arriving after a journey of 11.6 billion years: from when the Universe was just 2.2 billion years old.
Because of how these Blazars are distributed in space and (lookback) time, we have to model when the Universe transitions from being opaque to transparent in gamma rays, which the Fermi-LAT team was able to do as part of this work.
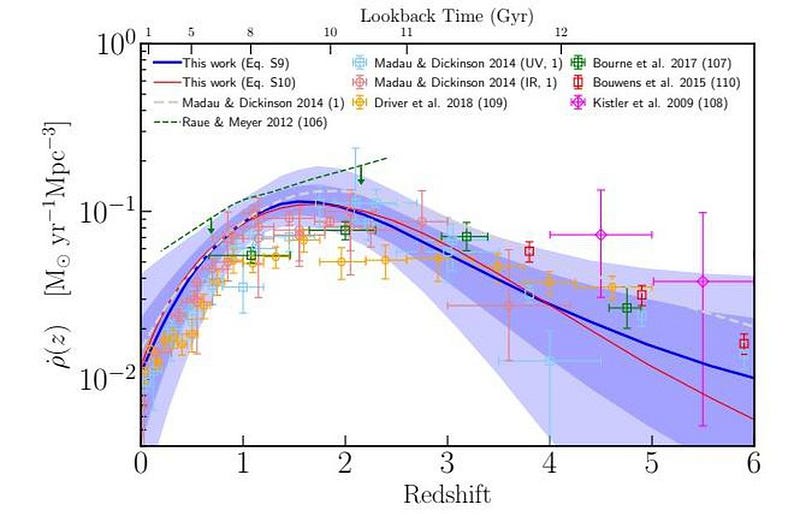
The net results they found agreed with previous work and improved the precision: the Universe had its star-formation rate peak when it was approximately 3 billion years old, and the star-formation rate has been falling ever since. Today, it’s at just 3% of that early, maximal rate, and the rate that we’re forming new stars in the Universe continues to drop.
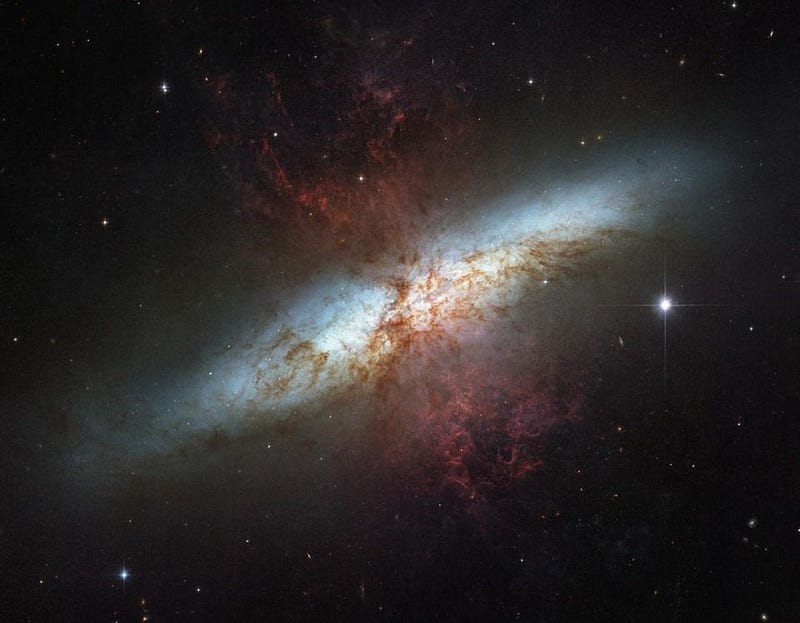
But one interesting and novel result to come out of this study really is revolutionary. According to the lead author of the Fermi-LAT study, Marco Ajello:
From data collected by the Fermi telescope, we were able to measure the entire amount of starlight ever emitted. This has never been done before.
That’s right: for the first time ever, we’ve been able to measure the entire amount of starlight emitted throughout the history of the Universe.
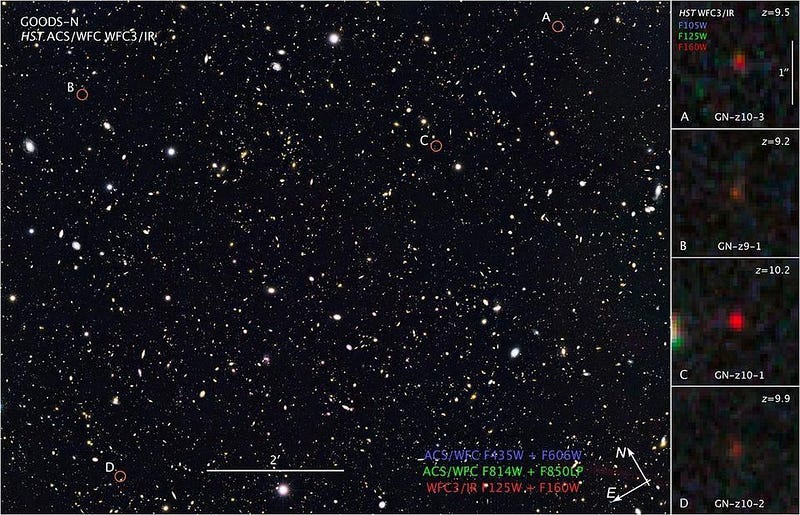
The total amount? It corresponds to a total of approximately 4 × 10⁸⁴ photons, which is an amazing large number: thousands of times larger than all the protons, neutrons, and electrons present in our Universe combined. But this is still a very, very small number compared to all the photons that exist in the Universe as part of the leftover radiation from the Big Bang, which number approximately 10⁸⁹-to-10⁹⁰: hundreds of thousands times as many photons as stars have ever created.
Still, it brings up a fascinating cosmic coincidence. The average energy of these photons from starlight is approximately 10,000-to-100,000 times the average energy of a photon left over from the Big Bang. When all is said and done, the energy produced by all the stars, in terms of radiation, now almost equals the amount of energy in photons from the Big Bang itself.
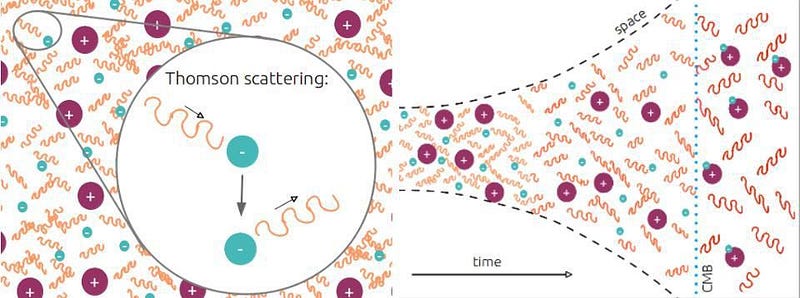
An enormous part of our cosmic history has just been revealed for the very first time. We can bypass the foregrounds of our own Solar System, thanks to these gamma-ray signals and how they interact with the extragalactic background of starlight, to understand and measure how star-formation has occurred over all of cosmic time in our Universe, and to infer the total amount of starlight ever produced.
In the future, scientists may be able to go back even farther, and probe how stars formed and emitted light back before the Fermi-LAT team’s instrumentation is capable of reaching. Star formation is what turns the primordial elements from the Big Bang into the elements capable of giving rise to rocky planets, organic molecules, and life in the Universe. Perhaps, one day, we’ll find a way to reach all the way back to the earliest moments of our Universe, uncovering the truths behind the greatest cosmic mysteries of all. Until then, enjoy each and every step — like this one — that we take along the journey!
Ethan Siegel is the author of Beyond the Galaxy and Treknology. You can pre-order his third book, currently in development: the Encyclopaedia Cosmologica.