James Webb’s record-breaking first science image: explained
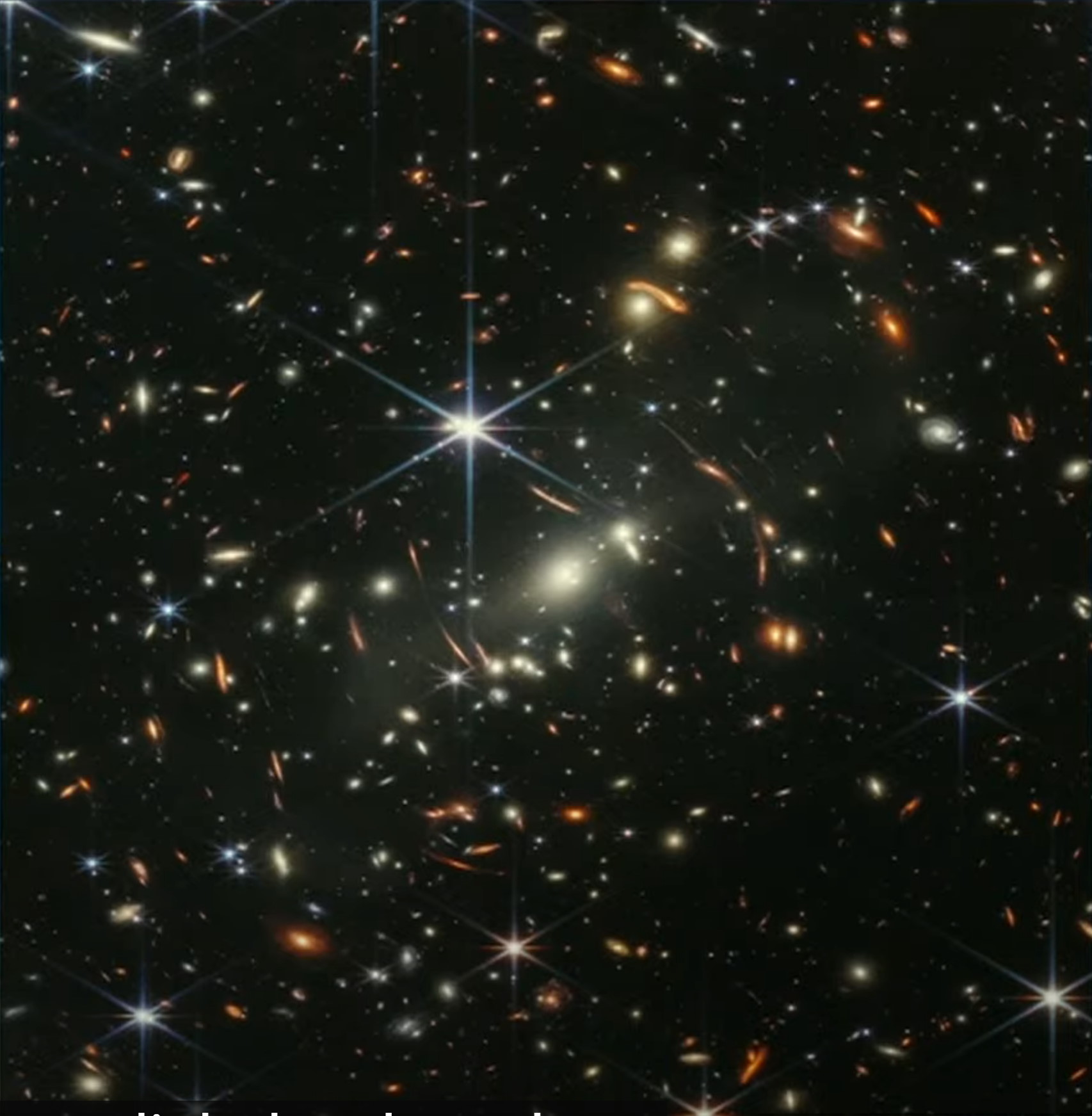
- With the release of James Webb’s very first deep-field image of the lensed galaxy cluster SMACS 0723 and all the background objects it stretches and magnifies, we’ve seen deeper into the distant Universe than ever before.
- There are new features revealed, new galaxies discovered, and new features about previously discovered objects that JWST’s unique capabilities have enabled us to uncover.
- Here’s the science behind what’s been done so far, how it was accomplished, and what it all means. This is the culmination of so much hard work, but it’s only just the beginning, too!
On July 11, 2022, history was made as the very first science image of the James Webb Space Telescope (JWST) was unveiled to the world. Upon its release, it immediately broke the cosmic record for the deepest view ever taken of the Universe: a remarkable feat. This record had previously been held by the Hubble Space Telescope, which first set the record back in 1994 with the original Hubble Deep Field, and which broke its own record numerous times.
Prior to JWST’s first science release, the deepest view of our cosmos came from the Hubble eXtreme Deep Field: a region of space so small it takes up just 1/32,000,000th of the sky. Within it, 5500 galaxies were found, spanning almost the entire history of the Universe: from just 400 million years after the Big Bang until today, or from when the Universe was merely 3% of its current age all the way to its present state.
That image, below, represented the deepest view of the Universe for a full decade. But by simply using its suite of instruments to observe a run-of-the-mill galaxy cluster, SMACS 0723, the JWST has shown us the Universe as we’ve never seen it before. Here’s the science behind the image, along with precisely what gives us such an unprecedented view.
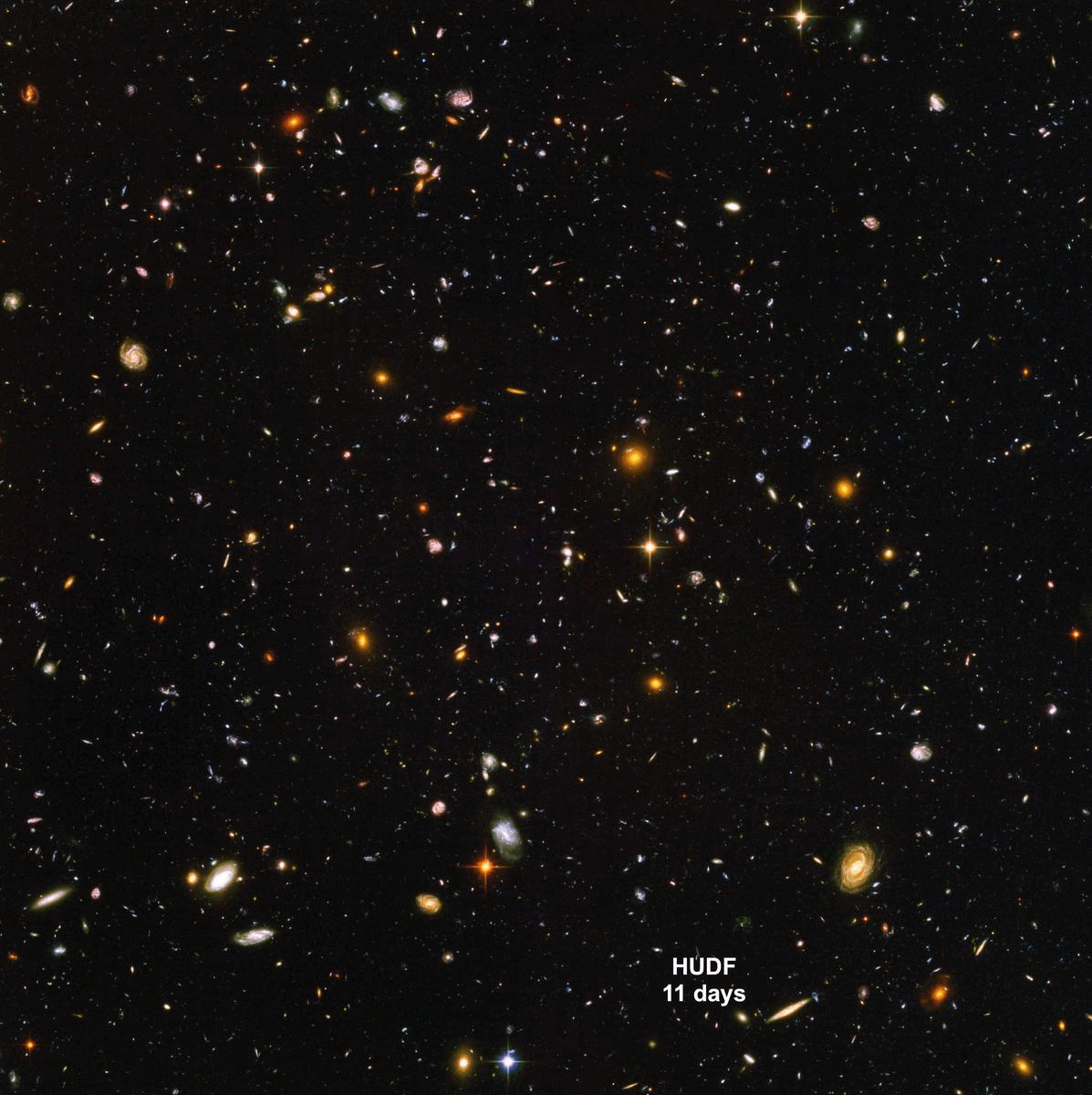
There are a few keys to taking a “deep” view of the Universe. The first is to find an area of the sky that’s relatively pristine:
- with no bright stars from within the Milky Way in its field of view,
- with no bright, extended, extremely nearby galaxies in the vicinity,
- where there’s only a small, negligible amount of neutral, light-blocking matter due to the Milky Way’s foreground,
- and where even the brightest stars and galaxies remaining are faint enough so that they won’t be able to saturate the detectors and instruments under any settings.
Then, in as many relevant filters (which probe a very specific wavelength range) as possible, you take a series of images where you simply gather light, one photon at a time, from the same region of sky. Images that use the same filter — i.e., which view the region of the sky in the exact same wavelength range — then get “stacked” together: where the light from each individual frame gets added together, exposing the faintest objects perceptible in that wavelength range.
And, at last, multiple different filters are combined to produce images. We assign colors to each filter or set of filters, and then our eyes interpret the data the same way we’d interpret a full-color photograph.
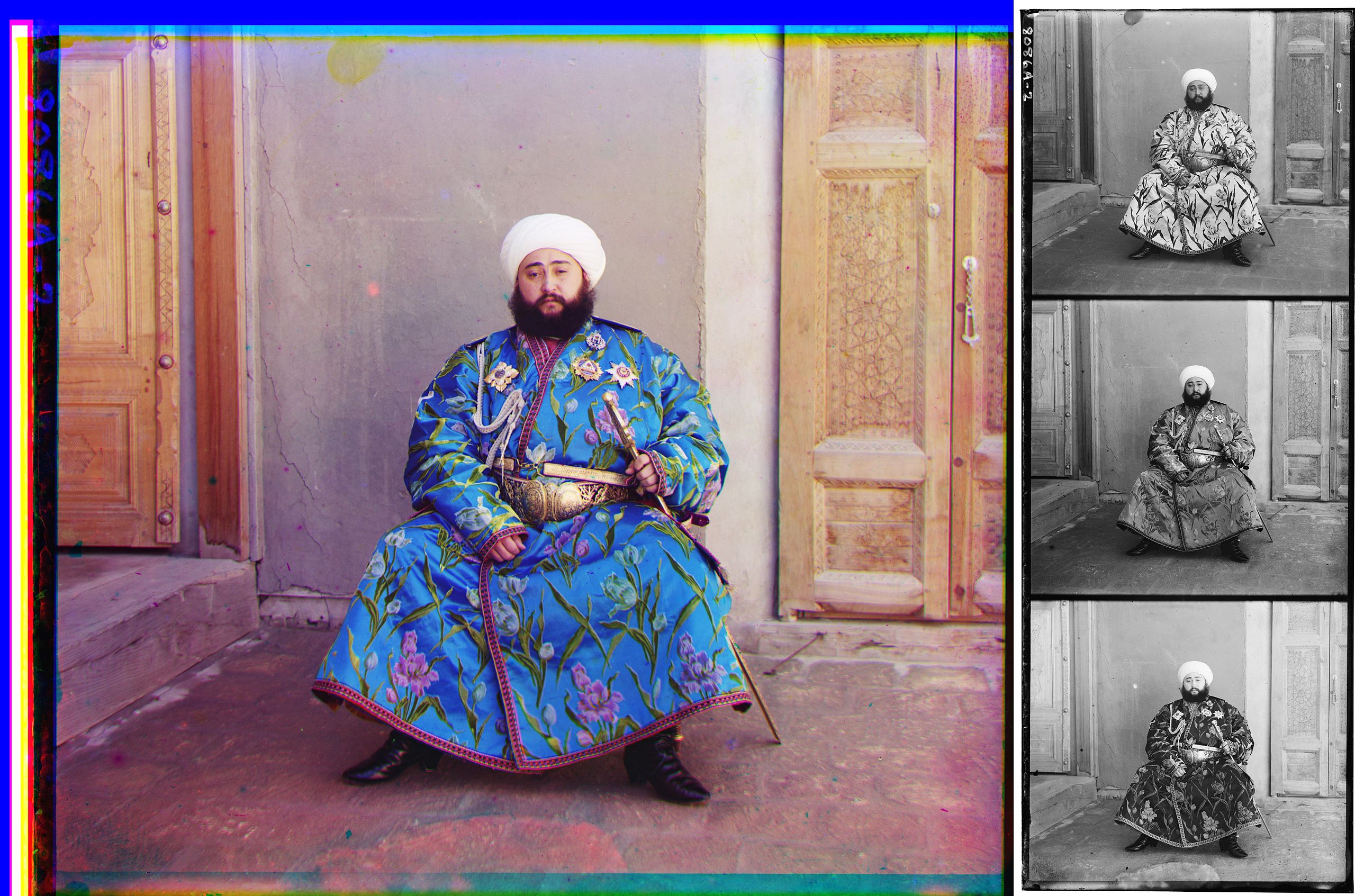
For the Hubble Space Telescope, it was primarily designed as an optical observatory: optimized to look at the same wavelengths of light that human eyes are sensitive to. Because it’s located in space, far above Earth’s atmosphere, it doesn’t have the same limitations that a ground-based telescope possesses: it can look at wavelengths that are otherwise blocked by Earth’s atmosphere. That means that it can see ultraviolet (shorter-wavelength) light as well, where the atmosphere is only partially transparent to it, and it can also see into the infrared (longer-wavelength) portion of the spectrum, where only a selection of wavelength ranges aren’t completely blocked by our atmosphere.
Each different wavelength range will have its own unique resolution when it comes to the light that’s gathered. In general, the resolution is determined by the number of wavelengths of light (in that particular wavelength range) that fit across the diameter of your telescope’s primary mirror. Although human vision extends from 400 to 700 nanometers, telescopes can assign filters to cover whatever wavelength range the operators desire.
For Hubble, with its 2.4-meter diameter mirror, that means its “blue” filter (of about 400 nanometers) has about twice as good a resolution as its “red” filter (of about 800 nanometers), and its infrared filters (which span from 1050 nanometers to 1600 nanometers) are again only half-as-sharp at the longest wavelengths.
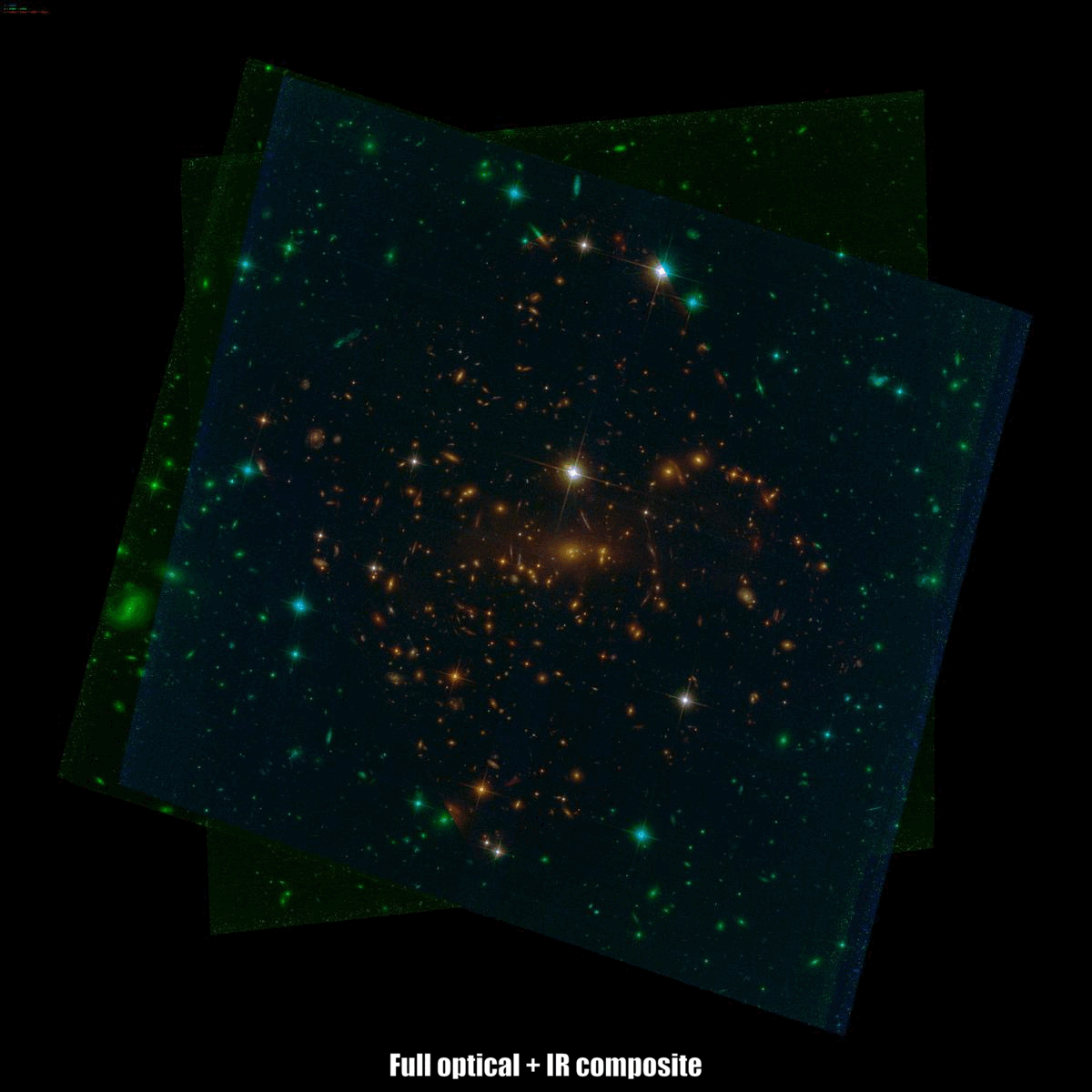
Above, you can see what a few different views of galaxy cluster SMACS 0723 — the target of JWST’s first science observations — looked like to Hubble’s eyes. Although you can’t quite tell, there are actually seven different sets of filters used as part of the final image composite. For Hubble:
- there are three optical filters, at 435, 606, and 814 nanometers, assigned to red, green and blue, respectively,
- there are four infrared filters, at 1050, 1250, 1400, and 1600 nanometers, all assigned-and-stacked to the same infrared channel,
- and the overall composite combined all seven filters, assigning “blue” to the 435 nm channel, “green” to the 606 + 814 nanometer channel, and “red” to all four infrared channels combined.
By looking at details in these channels separately, you can notice a slew of features. Cooler stars and diffuse starlight are brighter in the infrared. Features of a galaxy’s disk are sharpest in the shortest wavelengths, and become more smeared-out at longer and longer wavelengths. This is expected, but it’s also profound to see it in action. A filter at 1600 nanometers isn’t only one-fourth as good as a filter at 400 nanometers; it’s actually only one-sixteenth as good. A galaxy that takes up 64 pixels (8-by-8) in Hubble’s blue filter would only take up 4 pixels (2-by-2) in Hubble’s farthest infrared filter.
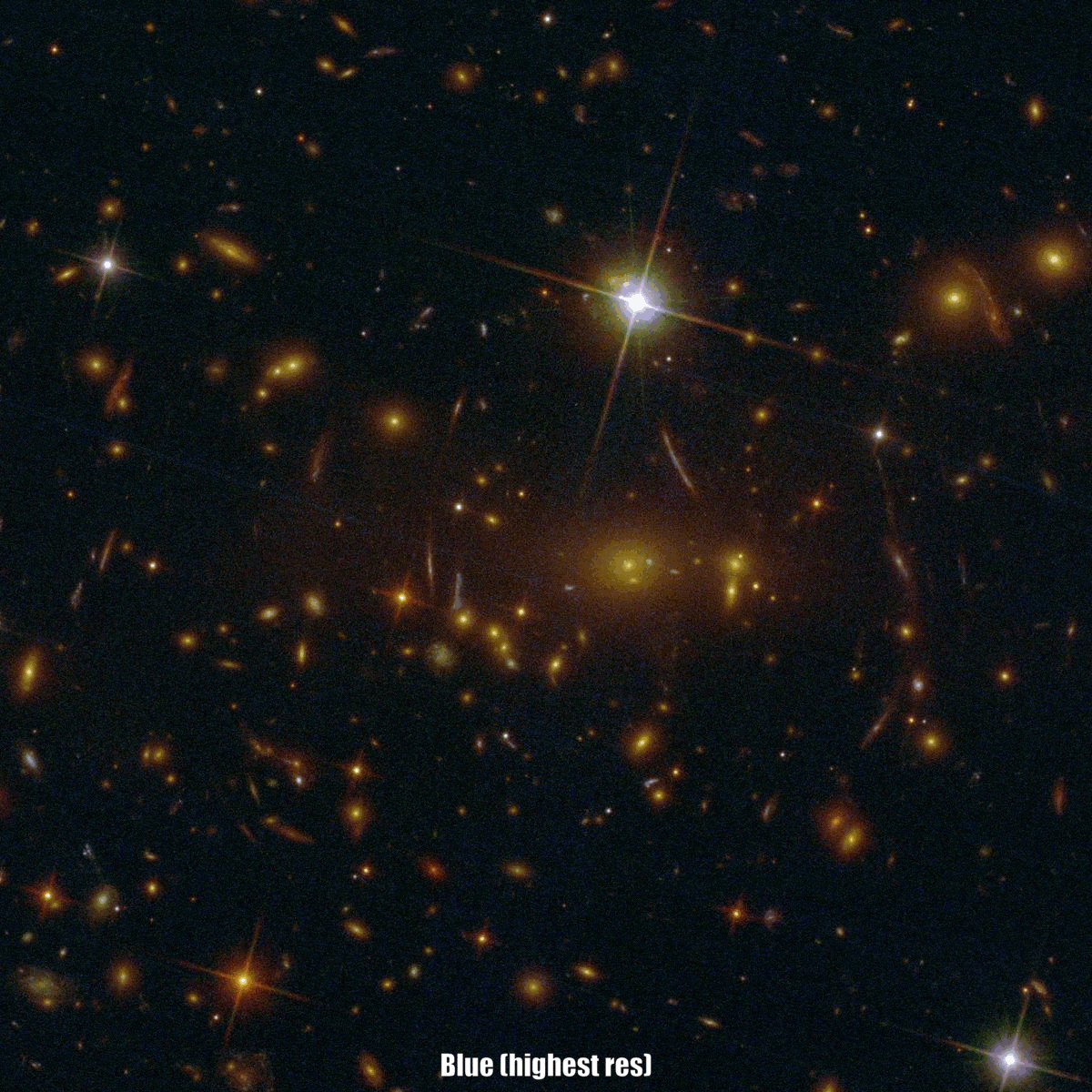
There are two factors at play that limit, at a fundamental level, what Hubble can see; these two factors are literally the difference-maker between Hubble and its successor: JWST.
1.) Hubble is warm; JWST is cold. If you want to detect anything and this goes for anything, ever, under any circumstances you need to be able to see a signal from what you’re measuring over and above the noise inherent to your instrument. Infrared radiation is simply a consequence of heat: objects at a certain temperature radiate heat, and that heat shows up as photons of a particular wavelength. The hotter you are, the more photons you’ll emit at higher and higher energies (and shorter and shorter wavelengths).
In low-Earth orbit, Hubble, even wrapped in its highly reflective coating, still hovers somewhere around 200 K and up. This is what limits its wavelength capabilities: beyond ~2000 nanometers (2 microns) or so, the observatory produces too much of its own internal radiation to do useful science. JWST is, thanks to its 5-layer sunshield, passively cooled down to below 40 K for all of its instruments, including NIRCam, the Near-Infrared camera, which can see all the way out to ~5000 nanometers (5 microns) without any active cooling. And then MIRI, the Mid-Infrared instrument, is actively cooled down to 6 K, enabling it to see light between 5000 and 28000 nanometers (5-28 microns) at its ultimate limits.
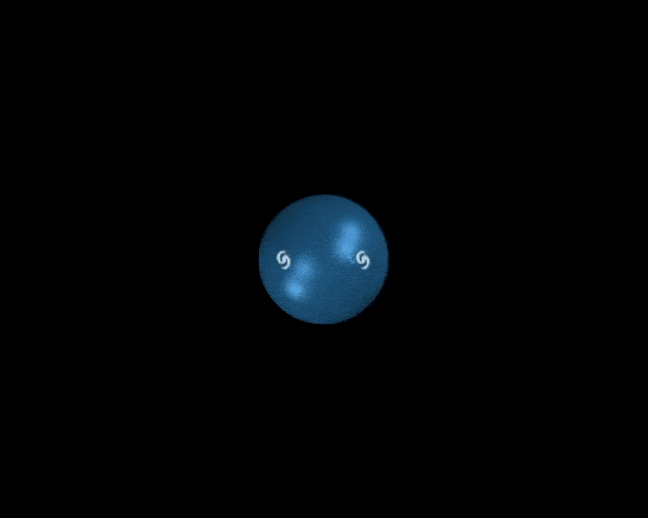
This is really, really important for scientific discovery, as you can’t see the most distant objects by looking in the same wavelengths as the ones where that light was initially emitted. The Universe isn’t static, it’s expanding: distant, gravitationally unbound galaxies all recede from one another against the backdrop of expanding space. When a distant object emits light — even, initially, very blue light — the expansion of the Universe stretches that light to longer and longer wavelengths.
Hubble, even at the longest-wavelengths that it’s sensitive to, can’t really take us back to galaxies that emit light from the first ~3% of the Universe’s history. But thanks to its NIRCam and MIRI capabilities, JWST can see much longer wavelengths, enabling it to reveal objects that are undetectable by warmer observatories.
In fact, you can see this by remembering that Hubble runs into its limits only slightly beyond 1.6 microns as shown by the grey bar in the image below. But JWST can see light that’s ~3 times longer with its NIRCam instrument alone, including with numerous filters that would be useless aboard Hubble.
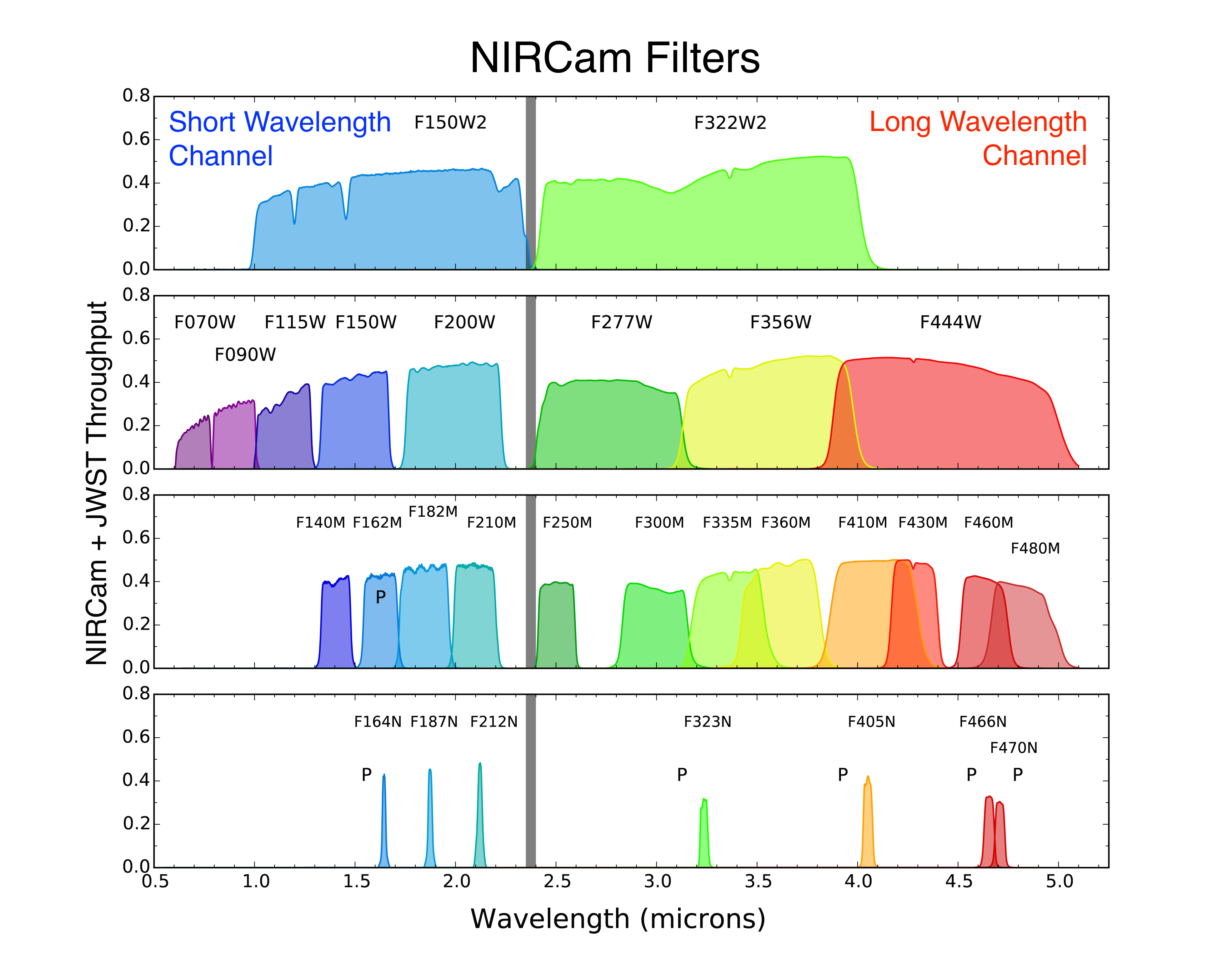
There are objects out there so distant that their light takes 13.4 billion years or more to reach us, and that takes us beyond the limitations of Hubble. But that’s where the cosmic frontier is: beyond the limits of what we’ve already seen with pre-existing technology. If we want to see the earliest galaxies, the first stars, and the youngest objects ever to form in the Universe, we simply have to go beyond the capabilities of current technology. That means longer wavelengths and cooler observatories, and that’s precisely what JWST is delivering.
But it gets even better than NIRCam. By using the MIRI instrument, which is the only actively-cooled instrument on board the JWST, we can go out to a whopping ~14 times the maximum wavelength that the Hubble Space Telescope is sensitive to.
Because of Hubble’s wavelength limitations, many of the galaxies that are currently classified as the most distant — including the dubious record-holder, HD1 — actually lack the spectroscopic confirmation needed, because the spectral lines that we’d look for are beyond the capabilities of even the finest of Hubble’s instruments. With JWST’s exquisite and comprehensive wavelength coverage, however, that problem will disappear.
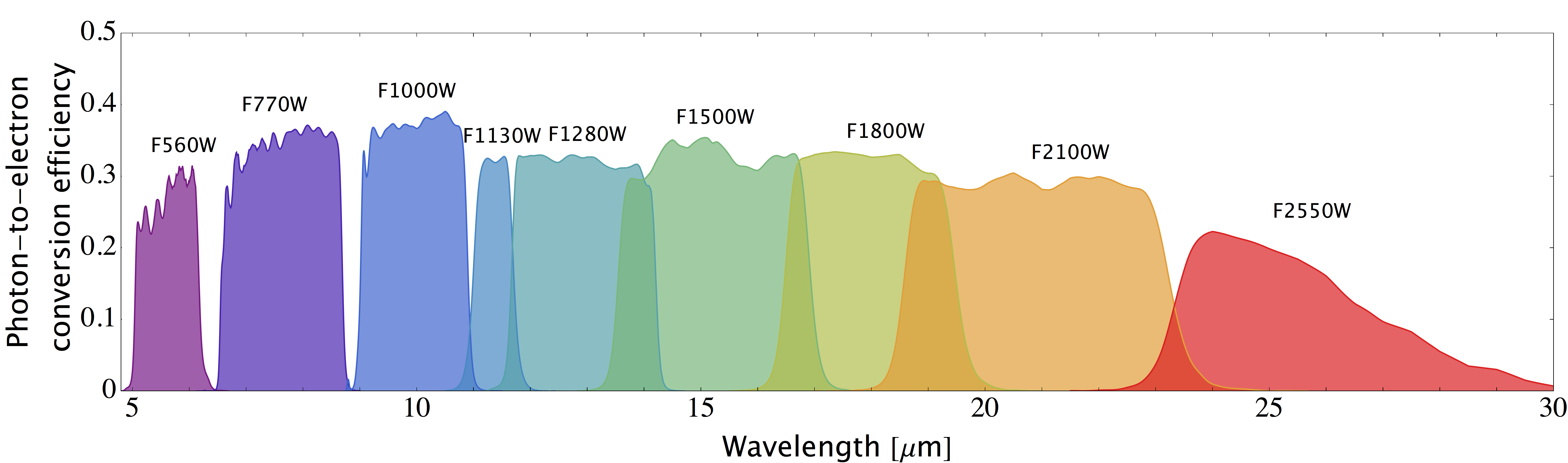
So that’s why an increased wavelength range is so important if we want to overcome Hubble’s limitations, which JWST does. But there’s another way that JWST surpasses Hubble as well that’s just as important.
2.) Telescope size determines both resolution and light-gathering power. If you want to see farther into the distant Universe, and to see features you couldn’t see before, you have to increase the total amount of light you capture, and also observe the Universe at superior resolutions. With JWST, the NIRCam images, especially at the lowest wavelengths, will produce sharper images than Hubble ever could, owing to its large, 6.5-meter primary mirror that’s 270% the diameter of Hubble’s.
But at all wavelengths, JWST gathers more than 7 times the total amount of light as Hubble, meaning that it can not only expose fainter, more distant objects than Hubble ever could, but that it can do it with less observing time than Hubble would ever require. What Hubble requires a week for, JWST can do better in a day. What Hubble requires two months for, JWST can outperform in a week.
The reason JWST can see what Hubble cannot is because of the difference in size, temperature, wavelength coverage, and the correspondingly-optimized instrument suite.

So, with all of that out of the way, let’s take a detailed look at the very first deep-field image from the JWST!
It is, as expected, of the galaxy cluster SMACS 0723, although it’s also about so much more than that. This massive galaxy cluster contains thousands of Milky Way-analog (or more massive) galaxies and represents a tremendous concentration of mass in the Universe. The presence of this mass is enough to actually bend and distort the fabric of spacetime, causing light from behind the cluster to become:
- bent,
- distorted,
- often split into multiple images,
- and, perhaps most importantly, magnified.
The power of gravitational lensing, and strong gravitational lensing explicitly, reveals background sources that could never be practically seen otherwise. But this natural magnifying glass amplifies the light we receive, even at such long wavelengths, so JWST is able to reveal a number of objects never seen before because they were either too faint, too distant (and hence, their wavelength was stretched to too great a value to be seen previously), or a combination of the two.
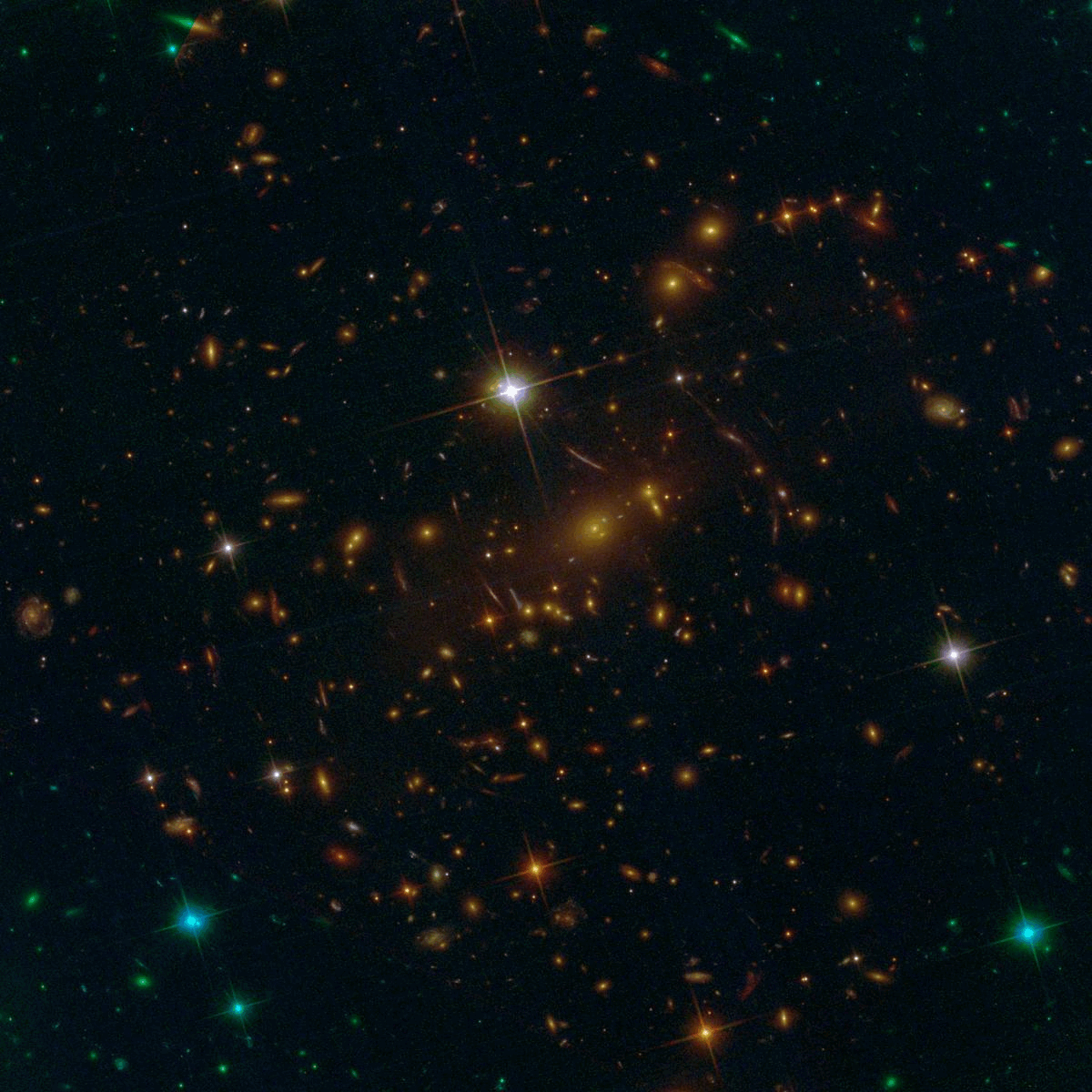
But take a look at what we’re seeing! Compared even to the full composite Hubble image of the exact same region, JWST is seeing both objects that have never been exposed before and also details within previously discovered objects that were completely obscure before it. Contained within this first JWST Deep Field are:
- a number of faint “blobs” that aren’t seen in the Hubble image, corresponding to galaxies that were too faint and/or too long in wavelength to be seen previously,
- a number of objects that appeared only as faint smudges, previously, that now have obvious structure to them, such as spiral arms, resulting from JWST’s improved resolution, light-gathering power, and superior wavelength coverage,
- a brighter, wider glow from the central region: an example of what’s almost certainly intracluster light, inherent to most galaxy clusters that aren’t largely gas-depleted,
- and numerous instances of “smudges” that were seen previously that are now seen to be multiple galaxies that appear to overlap: a tremendous demonstration of JWST’s superior resolution.
Although the official image release doesn’t include scientific data, a number of these objects are claimed to have their light come to us from as far back as 13.5 billion years ago: pushing our earliest cosmic views back by approximately 100 million years. A closer view, even without knowing any further specifics, reveals objects in the JWST image that simply were unseeable with Hubble’s technology.
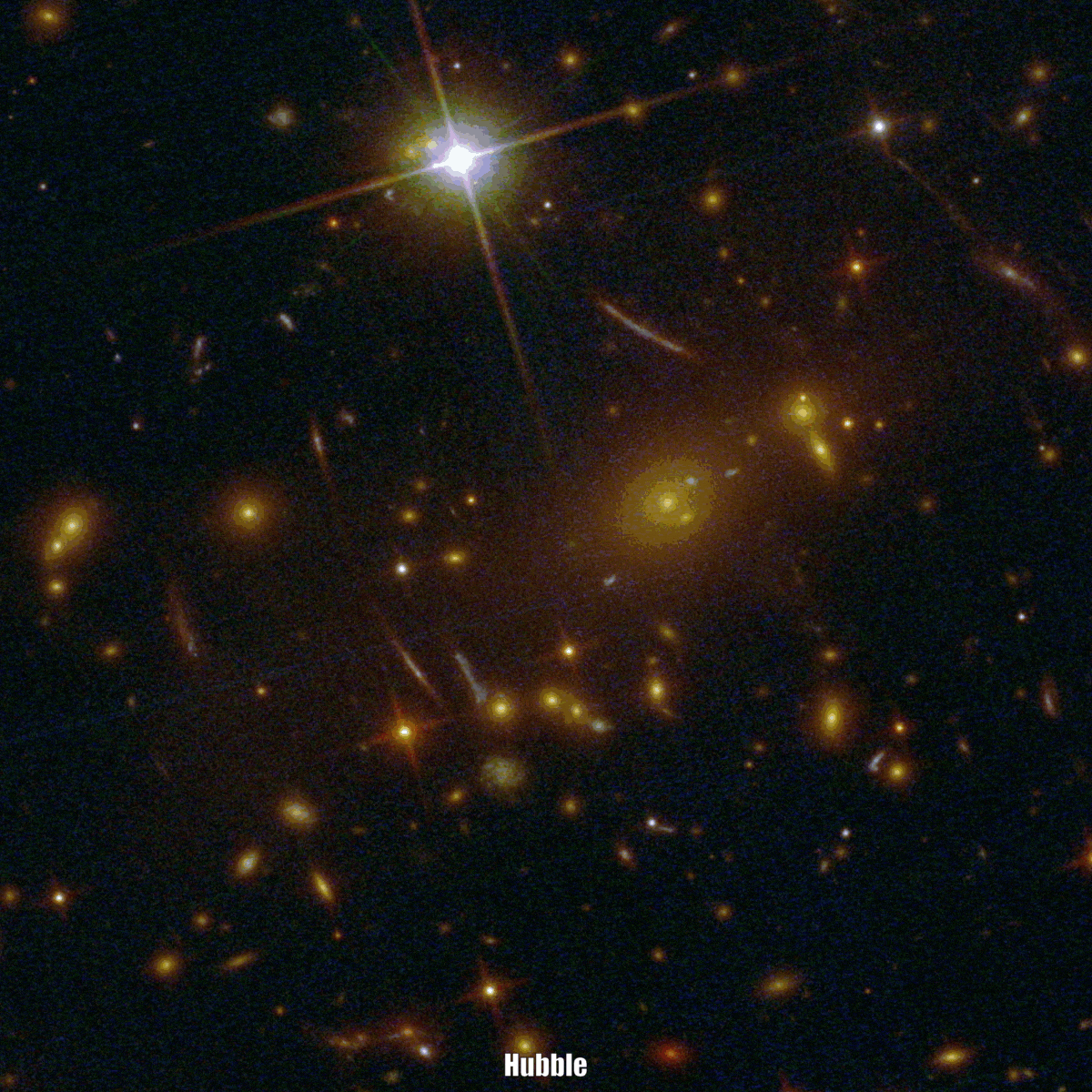
With all of that said, it’s important to remember the following: this is only the beginning for JWST. This image, along with all of the record-breaking data contained within it including the unprecedented views of ultra-distant galaxies, never-before-seen quasars, and the stellar populations inside each of them is just the first deep-field view of the Universe that JWST has delivered. It’s not going to be the deepest; it’s not going to be the longest; it’s not going to be the widest-field or largest-area of JWST’s views. It’s just the first science result using this new observatory in this particular manner.
Over the coming years and decades, an enormous number of cosmic records are going to be broken, most of them over and over again. A decade from now, we’ll likely have dozens of galaxies fainter, more distant, and with more pristine stellar populations that go beyond any of the cosmic record-holders lurking in the lensed field of this galaxy cluster. A new generation of astronomy is officially underway. Although the best is yet to come, each new observation, especially at this early stage, exposes a new portion of our shared cosmic story for the very first time. The Universe, to everyone studying it, will never be the same again.