What Came First: Inflation Or The Big Bang?
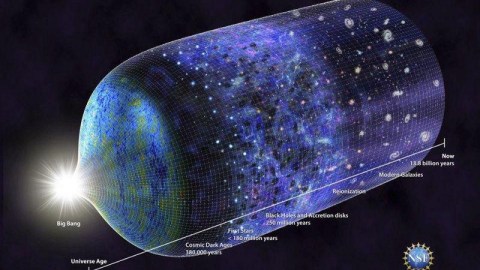
The origin story for our Universe got a major revision nearly 40 years ago. Time to catch up.
13.8 billion years ago, all the matter and energy contained within our Universe was concentrated into a volume of space about the size of a soccer ball. Even with all that energy in such a small space, however, we didn’t collapse into a black hole. Instead, the Universe expanded at a rapid rate that balanced the energy density so precisely that, for all of our measured cosmic history, we’ve walked that fine line between expanding and recollapsing.
Today, all we can see within the Universe extends for some 46 billion light-years in all directions, and scientists can trace this origin back to a hot, dense, more uniform and more rapidly expanding state. Like many theorists, you might be tempted to extrapolate this back even farther, to an arbitrarily hot and dense state: a singularity. But that temptation is the root of most of our misunderstandings surrounding the birth of the Universe. The Big Bang wasn’t the beginning, after all. Instead, that honor goes to cosmic inflation, and everyone should understand why.

When we look out at the Universe today, we see a number of observable facts that cry out for an explanation. They include:
- the fact that more distant galaxies appear to recede from us in direct proportion to their distance from us,
- the fact that galaxies, at greater distances, appear to be smaller, bluer, younger, and less evolved,
- the fact that the Universe, at greater distances, appears to be less clumpy and more uniform, with less clustering on large-scales,
- the fact that the percentage of heavy elements (atoms heavier than hydrogen and helium) asymptotes to 0% at the greatest distances,
- and the fact that we see a very cold but clearly identifiable background of blackbody radiation in all directions in space.
Remarkably, one framework is consistent with each and every one of these observations: the Big Bang.
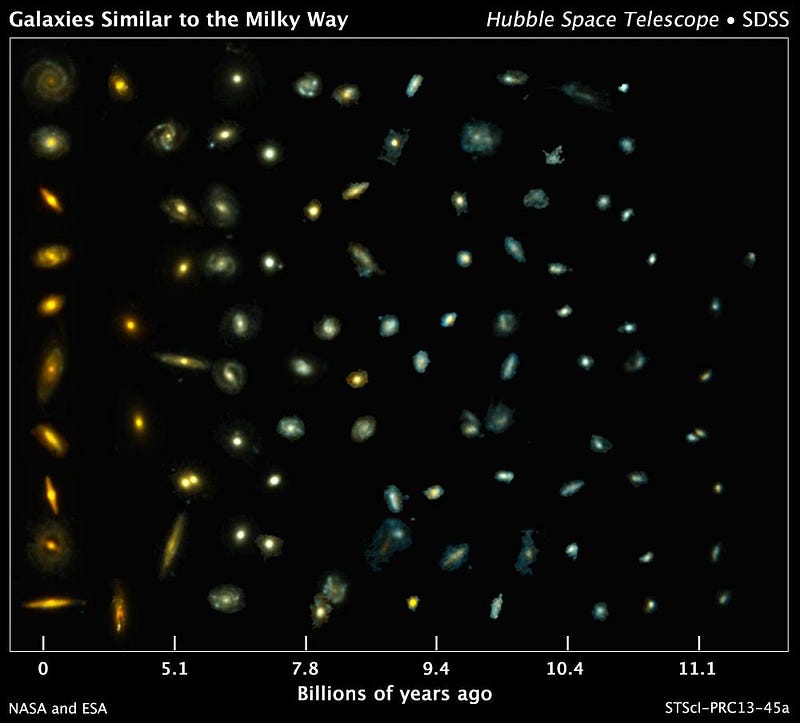
According to the big idea of the Big Bang, the Universe was hotter, denser, and more uniform in the past, and that it evolved into what it is today by expanding, cooling, and gravitating to form a great cosmic web. The fabric of space itself expands as time goes on, as the laws of General Relativity demand for a Universe that’s filled with roughly equal amounts of matter and energy in all directions and locations, causing the wavelengths of photons to stretch, the kinetic energy of massive particles to decrease, and enabling gravitational imperfections to steadily grow.
In the framework of the Big Bang, each of the observable phenomena mentioned earlier get a physical explanation: distant galaxies appear to redshift because the expanding Universe stretches the light’s wavelength; more distant galaxies really are younger and less evolved; the Universe was less clustered in the past; the primeval atomic ratios are 75% hydrogen, 25% helium, and 0.00000007% lithium; the leftover radiation was discovered in the mid-1960s.

That last discovery pretty much killed every one of the Big Bang’s alternatives, and installed the Big Bang as the cosmic origin story for everything within our observable Universe. The Universe emerged from this early hot, dense, and uniform state, and over time it expanded and cooled.
When it cools below a specific energy threshold, it becomes unable to spontaneously generate particles whose mass (via E = mc²) is too great; over the first few fractions-of-a-second, every antimatter particle other than positrons and anti-neutrinos annihilate away.
About 1 second after the Big Bang, neutrinos and antineutrinos “freeze out,” meaning their (energy-dependent) interaction rates drop to such a low frequency that they effectively never interact again.
And as we move forward, nuclear reactions occur and then cease; neutral atoms stably form, releasing that primeval radiation; gravitational imperfections grow on progressively larger and larger scales, leading to the formation of the first stars, then galaxies, and then the enormous cosmic web.
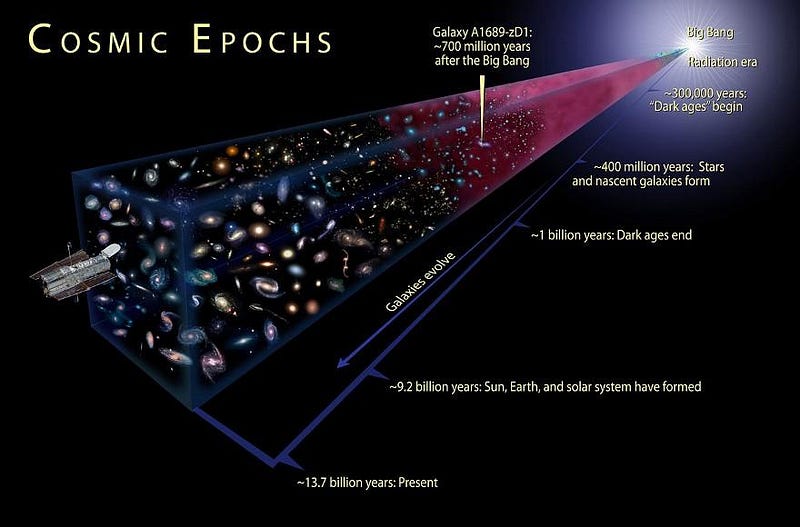
But what about the Big Bang’s origin story? Where did the Big Bang itself come from?
If you extrapolate the expanding and cooling Universe all the way back as far as theoretical physics allows you to go, you’ll come to an event in the past known as a singularity. Essentially, you’d be packing all the matter and energy in the Universe into a single point. (The laws of physics break down, and stop giving sensible answers, once you reach an extremely high energy of ~10¹⁹ GeV per particle, which corresponds to an “age of the Universe” of ~10^–43 seconds after the Big Bang.)
A singularity, from the perspective of General Relativity, is the only event that can correspond to the beginning or ending point of space and time. Therefore, we could extrapolate all the way back to a singularity in the framework of the Big Bang, and arrive at a point that we could legitimately refer to as “the beginning.”
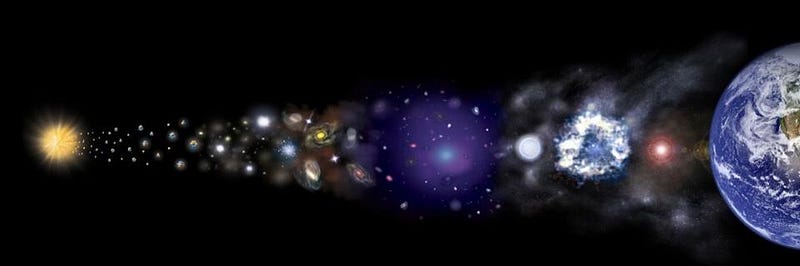
From the 1920s up through the 1970s, scientists thought they had a satisfactory story for our cosmic origins, and only a few questions remained unresolved. All of them, however, had something in common: they all asked some variety of the question, “why did the Universe begin with a specific set of properties, and not others?”
- Why was the Universe born perfectly spatially flat, with its total matter-and-energy density perfectly balancing the initial expansion rate?
- Why is the Universe the exact same temperature, to 99.997% accuracy, in all directions, even though the Universe hasn’t existed for enough time for different regions to thermalize and reach an equilibrium state?
- Why, if the Universe reached these ultra-high energies early on, are there no high-energy relics (like magnetic monopoles) predicted by generic extensions to the Standard Model of particle physics?
- And why, since the entropy of a system always increases, was the Universe born in such a low-entropy configuration relative to its configuration today?
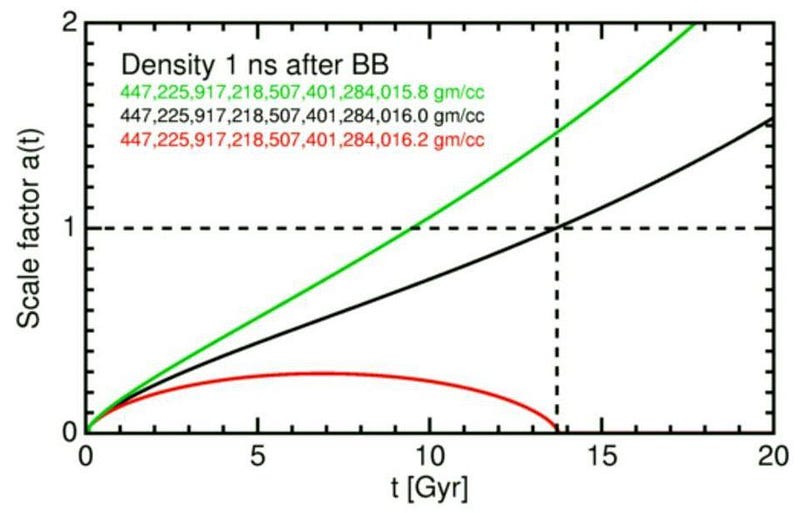
In physics, we have two ways of dealing with questions like these. Because all of these questions are about initial conditions — i.e., why did our system (the Universe) begin with these specific conditions and not any others — we can take our pick of the following:
- We can attempt to concoct a theoretical mechanism that transforms arbitrary initial conditions into the ones we observe, including that reproduces all the successes of the hot Big Bang, and then tease out new predictions that will allow us to test the new theory against the old theory of the plain old Big Bang without any alterations.
- Or, we can simply assert that the initial conditions are what they are and not only is there no explanation for those values/parameters, but we don’t need one.
Although it’s not clear to everyone, the first option is the only one that’s scientific; the second option, often touted by those who philosophize about the landscape or the multiverse, is tantamount to giving up on science entirely.
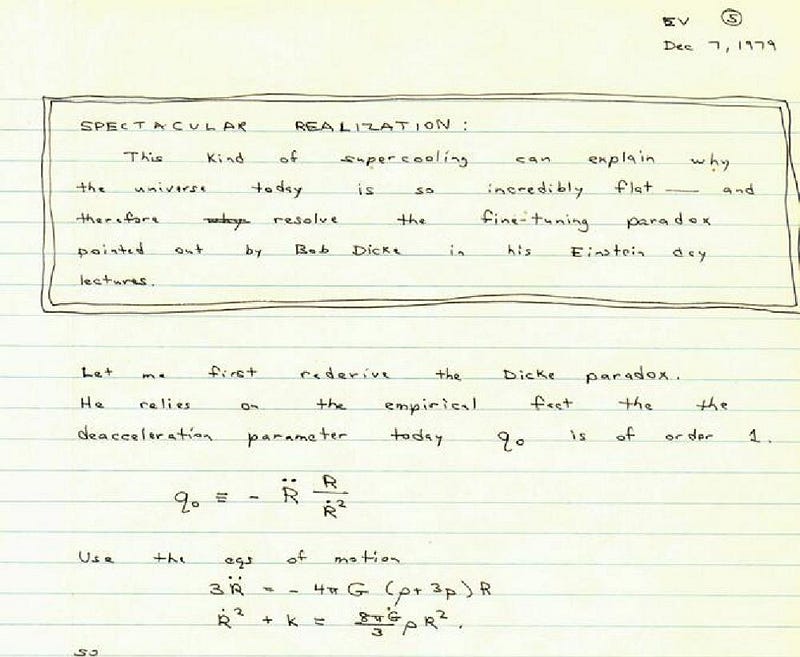
The big idea that actually succeeded is known, today, as cosmic inflation. In 1979/80, Alan Guth proposed that an early phase of the Universe, where all the energy wasn’t in particles or radiation but in the fabric of space itself instead, would lead to a special type of exponential expansion known as a de Sitter phase. In this state, any initial patch of the Universe that began inflating would:
- get stretched, on incredibly short timescales, to such a large size that its topology would become indistinguishable from flat to any observer,
- have the same initial conditions (density and temperature) everywhere, up to the scale of quantum fluctuations, which get superimposed atop a uniform background, since our entire observable Universe was once all causally connected in the same region of space in the distant past,
- only reach a maximum temperature that was significantly lower than the Planck scale (that 10¹⁹ GeV energy scale mentioned earlier) when inflation ends and transitions into the hot, dense, uniform, expanding-and-cooling state we associate with the hot Big Bang,
- and would go from a lower-entropy state of an inflating Universe to a much-higher entropy state of the hot Big Bang, where entropy will continue to increase as it does in our observed Universe.

Since inflation was first proposed and refined during the early-to-mid 1980s, we’ve learned a lot about our cosmic origins. In addition to reproducing the hot Big Bang’s successes and explaining these otherwise inexplicable initial conditions, it made six novel predictions about properties the Universe should have today, with four observationally verified and two not yet sufficiently tested to know for certain. Among most people who study the early Universe, inflation is accepted as the new consensus theory. We might not know everything there is to know about inflation, but either it — or something so similar to it that we don’t have an observation to tell them apart — must have happened.
With all that said, what does that mean for our cosmic origins? From a timeline perspective, what comes first: the Big Bang or inflation?
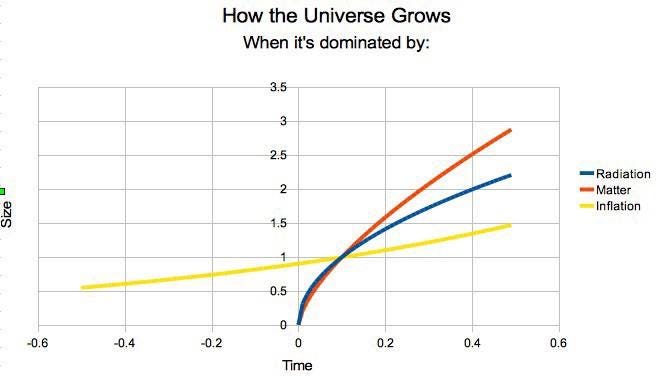
Believe it or not, the above graph contains all the information you’d need to know for certain. Two of the curves — red and blue — represent a Universe dominated by either matter or radiation. As you can clearly see, if you extrapolate them back arbitrarily to the past, you get an inifinitely small size at a finite time of t=0, which is a singularity.
But if at some early time, the Universe isn’t dominated by matter or radiation, but by a form of energy inherent to space itself, you get the yellow curve. Note how this yellow curve, since it’s an exponential curve, never reaches zero in size, but only approaches it, even if you go infinitely far back in time. An inflating Universe doesn’t begin in a singularity like a matter-dominated or radiation-dominated Universe does. All we can state with certainty is that the state we call the hot Big Bang only came about after the end of inflation. It says nothing about inflation’s origins.
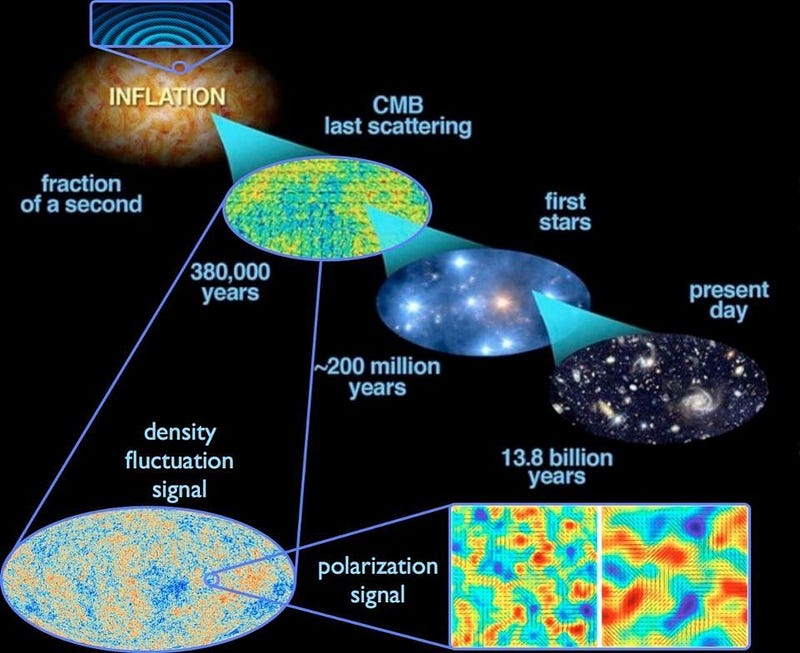
In fact, our entire observable Universe contains no signatures at all from almost all of its pre-hot-Big-Bang history; only the final 10^–32 seconds (or so) of inflation even leave observably imprinted signatures on our Universe. We do not know where the inflationary state came from, however. It might arise from a pre-existing state that does have a singularity, it might have existed in its inflationary form forever, or the Universe itself might even be cyclical in nature.
There are a lot of people who mean “the initial singularity” when they say “the Big Bang,” and to those people, I say it’s long past due for you to get with the times. The hot Big Bang cannot be extrapolated back to a singularity, but only to the end of an inflationary state that preceded it. We cannot state with any confidence, because there are no signatures of it even in principle, what preceded the very end-stages of inflation. Was there a singularity? Maybe, but even if so, it doesn’t have anything to do with the Big Bang.
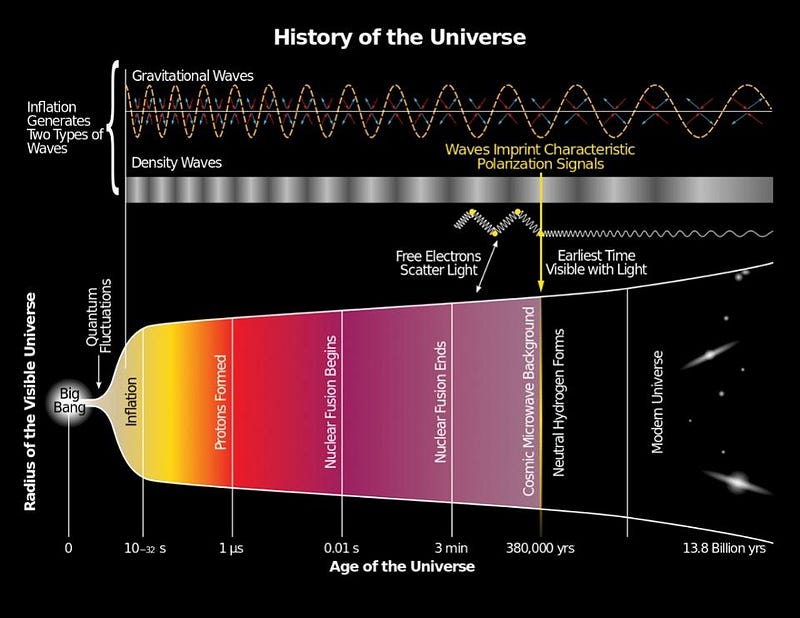
Inflation came first, and its end heralded the arrival of the Big Bang. There are still those who disagree, but they’re now nearly a full 40 years out of date. When they assert that “the Big Bang was the beginning,” you’ll know why cosmic inflation actually came first. As far as what came before the final fraction-of-a-second of inflation? Your hypothesis is just as good as anyone’s.
Ethan Siegel is the author of Beyond the Galaxy and Treknology. You can pre-order his third book, currently in development: the Encyclopaedia Cosmologica.