Will The LHC Be Humanity’s Last Gasp For Particle Physics?
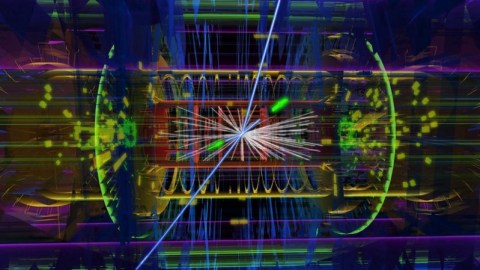
CERN’s bold new proposal has physicists confronting the biggest question of all: is building a new collider worth it?
If you want to discover anything novel about the natural, physical world we inhabit, you have to ask the right questions. In space, that means looking at the Universe with larger telescopes, broad wavelength ranges, wide fields-of-view, and superior instrumentation. In low-temperature physics, that means approaching absolute zero, extreme pressures, and more extreme and exotic quantum states of matter. And in particle physics, that means higher energies, more collisions, and superior detectors.
Sometimes, when you look at the Universe as you’ve never looked before, you find clues of something new. Sometimes you only find what you expect, but other times, you find the unexpected: the serendipitous discoveries that often lead to scientific revolutions and giant leaps forward in our understanding. With a bold new plan to build a transformative collider superseding the LHC, CERN is ready to push our frontiers far into the unknown. But is it too expensive, as detractors claim, for an uncertain science haul? The future of particle physics hangs in the balance.
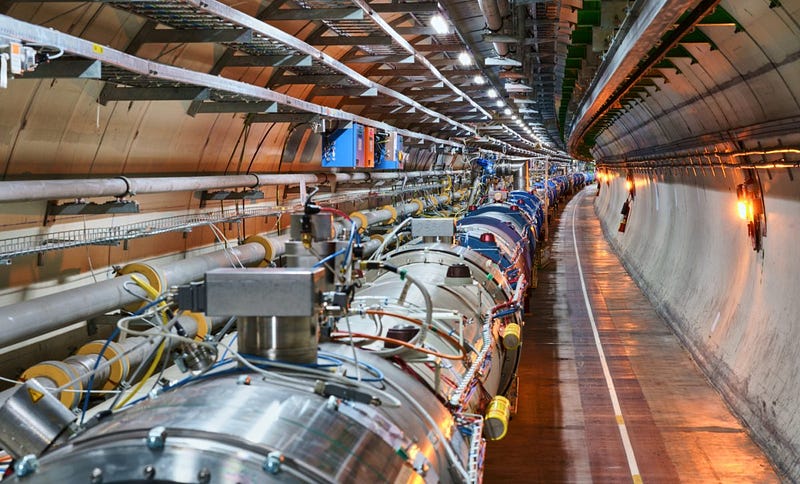
There are two types of approaches to problems in the physical sciences:
- the finesse approach, where you narrowly design an experiment or observatory to specifically address a particular question,
- or the brute-force approach, where you design an all-purpose, frontier-pushing experiment or observatory to probe the Universe in a fundamentally new way over our prior approaches.
The finesse experiments are very specific: you can typically perform them either quickly or cheaply, but the science you’re going to get out of them is restricted. You might learn how a single system behaves under a novel, previously unexplored condition. This can yield interesting and even groundbreaking results, but on its own, it lacks the flexibility that a revolutionary, large, high-powered data set can give you.
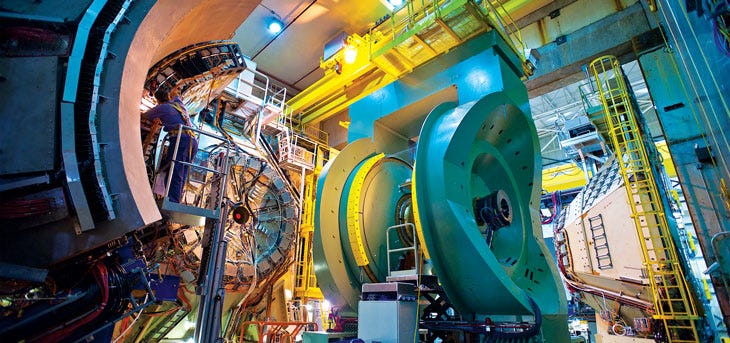
In order to continue to push these brute force frontiers in particle physics, scientists have had no choice but to work together. Whereas there were once more than a dozen cutting-edge particle accelerators that all pushed the limits of our knowledge in various ways, today we only have a single one at the energy frontier: the Large Hadron Collider (LHC) at CERN.
At the LHC, particles travel with seven times the energy of the world’s previous record-holder (the Tevatron at Fermilab, whose research programme came to an end nearly a decade ago), and with much greater collision rates, colliding about 100 times as many particles each second.
Both of these factors — energy and the total number of particle collisions — are vital for maximizing what you can discover. Although we typically associate Fermilab with discovering the top quark in 1995 and CERN with the Higgs boson in 2012, we were also able to measure a number of previously unknown particle properties over the final years of the Tevatron’s life.
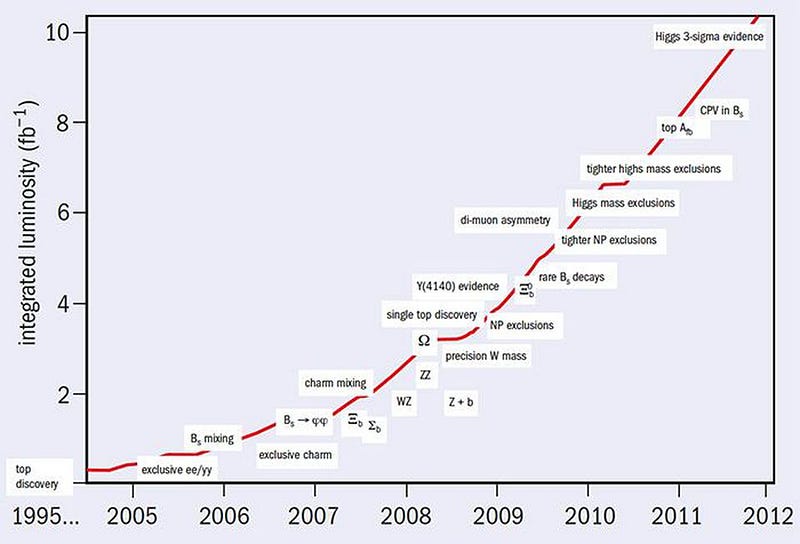
The LHC, meanwhile, is presently shut down as upgrades are performed on it. Although the energy won’t change very much as a result of this upgrade, the collision rates of particles in the accelerator — what particle physicists call luminosity — will increase tremendously. The LHC has been operating for nearly half its total lifetime, having first turned on back in 2008, and planned to operate until the early 2030s.
However, almost all of the data it will eventually collect is in our future; fewer than 3% of the total number of collisions that it will achieve over its lifetime have occurred so far. In terms of the potential for new discoveries, including:
- measuring rare decays,
- understanding how particles are created,
- probing the violation of fundamental symmetries,
- and searching for beyond-the-Standard-Model physics,
most of what the LHC is capable of lies in its future, not its past.
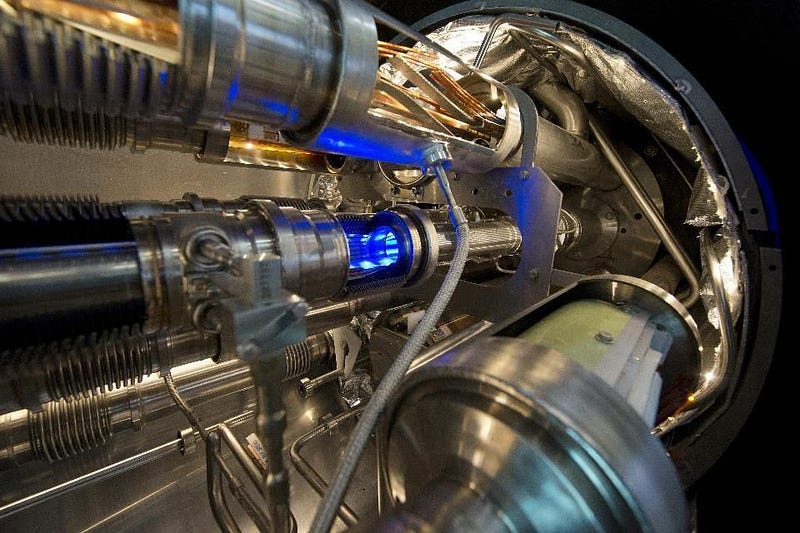
But at the same time, it’s important to recognize the fundamental limitations of not only this particular collider but of colliders in general: they can only reveal large-enough effects that show up at the energies they can probe. If we were looking for the Higgs boson (which requires an energy of ~125 GeV to create) and were only colliding particles at lower energies than that, then Einstein’s E = mc² ensures that we would never find it.
Reaching for higher energies is the most important factor in determining the “discovery potential” of a machine. And in order to achieve higher energies, at least for a circular collider (which reaches higher energies than a linear collider can), there are only two things you can do:
- build a larger ring with a larger turning radius,
- and/or put stronger magnets in your collider.
It’s the same principle as driving a racecar around a track: if you want to go faster without slamming your racecar into the track walls, you either need to build a track where the turns are wider rather than narrower, or you need to increase the no-slip friction between the tires and the road. In particle physics, a racetrack with less curvature is a larger circle, and increased friction between the car and the road is a stronger magnetic field.
In the jump from the Tevatron to the LHC, the radius increased by a factor of 4 and the magnet strength increased by a factor of almost 2, bringing the total energy up by a factor of 7. In order to make the next “step up” worth it, the ambitious plan put forth for a Future Circular Collider (FCC) and just approved by the CERN council, plans to make the same sized leap: to nearly four times the length and nearly double the magnet strength of the current LHC.
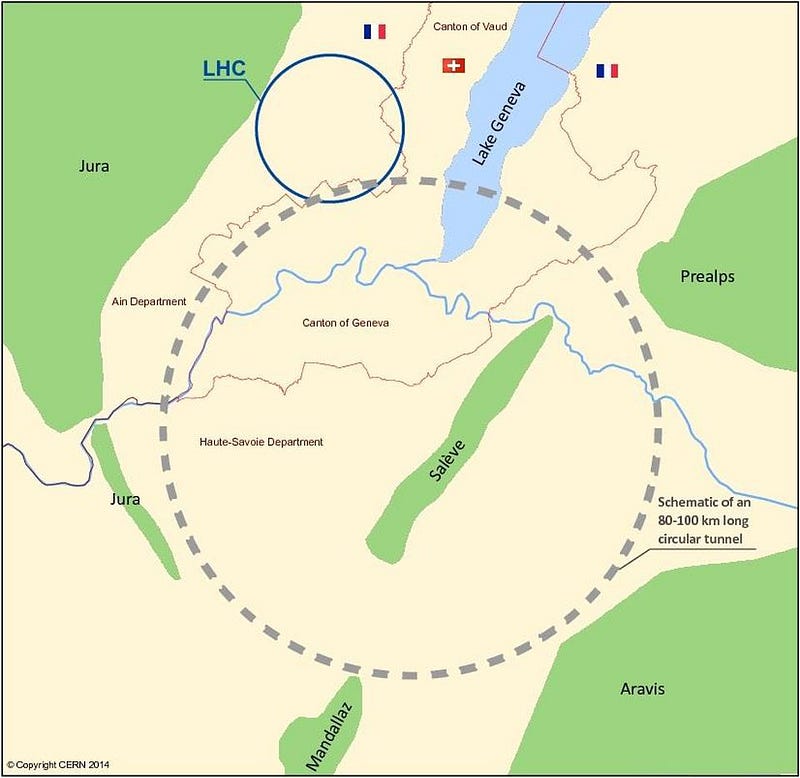
The current proposal for the FCC is truly a best-of-all-worlds scenario for particle physics. Yes, it’s expensive, but it touches all the bases as far how we can explore the frontiers of the high-energy Universe. This includes:
- running the world’s most powerful, highest-energy electron/positron collisions, which will enable precision studies of the heaviest, toughest-to-create particles in the Standard Model, including the Higgs, the top quark, and the W and Z bosons,
- an upgrade to a proton-proton collider, which will pass the 100 TeV energy threshold, compared to the LHC’s 14 TeV and the Tevatron’s 2 TeV collisions,
- and the capacity to maintain the expertise of the scientists that have devoted their lives to experimental particle physics at the energy frontier.
More than 17,000 people currently work at CERN: the majority of active particle physicists and associated scientists and technicians.
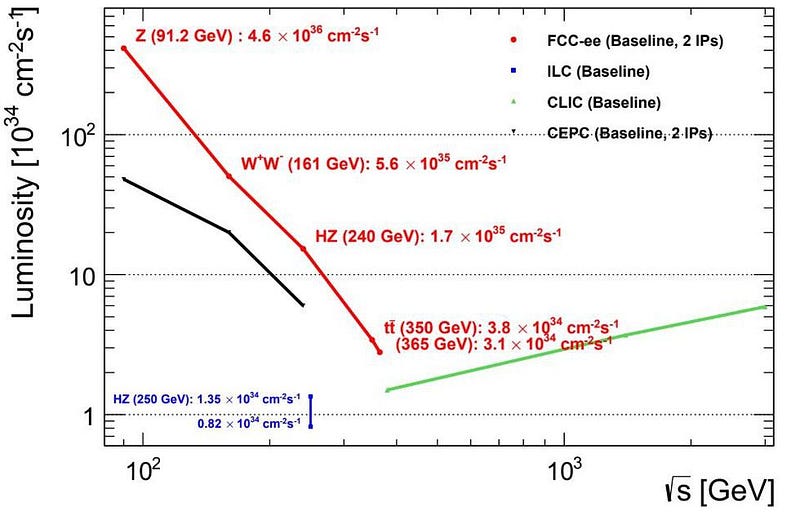
From a science point of view, it’s a no-brainer: if we look, we learn more about the Universe; if we don’t look, we don’t learn it. We have the Standard Model and our current understanding of it right now, but also a number of unexplained puzzles that we cannot answer. We don’t know, for instance:
- how our Universe, exactly, created more matter than antimatter,
- why the masses of the fundamental particles have the values that they do (and not any other values),
- how neutrinos get their masses,
- what dark matter and dark energy are,
- why the strong interactions don’t violate the combination of charge conjugation © and parity (P) symmetries,
along with many other mysteries. Building a more powerful, higher-luminosity collider is a way to probe these and other puzzles in a manner that no known “finesse-style” experiment can equal.
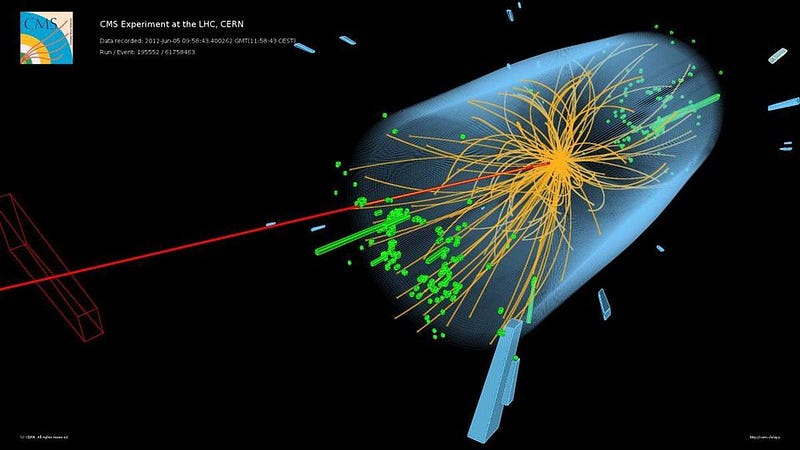
And yet, there are detractors. Some of them trot out the same arguments they always do when one argues against funding fundamental science: it’s not practical, it’s too expensive, there are too many other problems that deserve our resources, etc. The path back to the dark ages is paved with these arguments, and they are just as invalid for particle physics as they are for NASA, for evolutionary biology, or for geological sciences.
However, there is one major problem that the field must reckon with: neither the Tevatron nor the LHC has found any robust hints of physics beyond the Standard Model, and any future collider might not, either. Particle physicists call this the nightmare scenario, and it might be true. Sure, there’s new physics out there to be discovered, but if it won’t be revealed until we achieve energies that are billions of times what a terrestrial collider could ever reach, what’s the justification for building this machine?
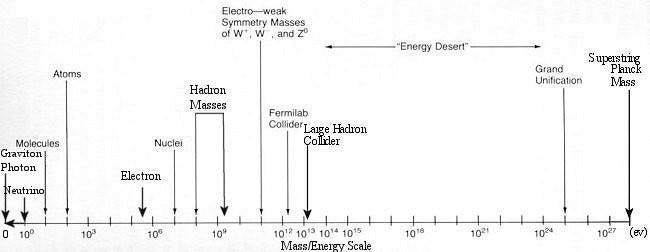
Theoretically, all of the popular ideas that are out there — supersymmetry, extra dimensions, string theory, various incarnations of quantum gravity, etc. — have no evidence for them in all the data from all of our experiments. It’s a real possibility that even if we put all this time and effort into the endeavor of a new collider, we’ll only learn new details about the Standard Model. There may be nothing new that’s fundamental for a new collider to teach us.
That is simply part of the adventure of doing science. Most ideas are not new ideas; most new ideas are bad ideas; most good ideas turn out to still be wrong. We have an opportunity to look where we’ve never looked before, and if we take advantage of it, we still might not find what we’re seeking. But if we look, we’ll learn what’s there. If we don’t, we won’t. Over the coming months and years, the world will decide whether this type of fundamental knowledge is worth investing in. If we choose it, that knowledge is ours for the taking; if not, the LHC will mark the end of frontier-pushing particle physics on planet Earth.
Ethan Siegel is the author of Beyond the Galaxy and Treknology. You can pre-order his third book, currently in development: the Encyclopaedia Cosmologica.