Quantum sensors use “spooky” science to measure the world with unprecedented precision
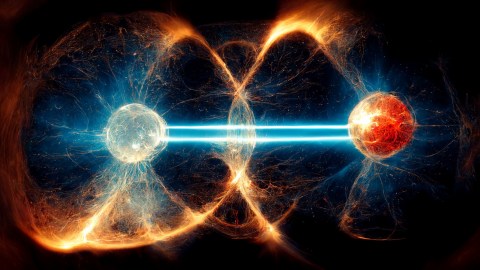
- Quantum systems and quantum entanglement can help us carefully sense an environment and measure it with unparalleled precision.
- A quantum sensor essentially watches how a particle interacts with its environment.
- Quantum entanglement may remain mysterious, but it has a very practical side, too.
When you stepped on your bathroom scale this morning, you probably measured your weight accurately within about a tenth of a pound. Chances are that is all you need. But there are times you want to weigh something with more precision, like a piece of mail. The scales at the post office will weigh an envelope more finely than your bathroom scale would. This is precision, and it is an important factor in measurement.
There are instances where extremely precise measurements are critical. Knowing how to precisely measure location allows GPS to help you navigate to the post office. Still more precise measurements allow a spacecraft to land on Mars.
Improved measurements can help us do more and understand more. This is where quantum systems and entanglement can be used. They can help us carefully sense an environment and measure it with unparalleled precision.
Extra sensory powers
Decoherence is a major problem for quantum communications. It happens when quantum particles interact with something in their environment — for example, the edge of a fiber optic cable — causing their wave function to collapse.
Decoherence happens because quantum states are intensely sensitive to their environment. This is a problem for quantum communications, but it is actually a benefit when it comes to sensing. Their reactions to small changes in the environment are precisely what make quantum sensors so accurate, allowing them to reach a precision we never before dreamed would be possible.
A quantum sensor essentially watches how a particle interacts with its environment. There are quantum sensors of different types that can measure all sorts of things — magnetic fields, time, distance, temperature, pressure, rotation, and a host of other observables. As we go into more detail about how quantum sensors work, we can get a glimpse of their power and how they can affect our lives.
Seeing deep into the ground
In the original Jurassic Park, paleontologists used some undefined, fictitious technology to compose an image of dinosaur bones hiding underground. The scene is a bit ridiculous, but it does help us understand the impact of a tool that allows us to see underground without digging. Such a technology might not help us find surprisingly intact dinosaur skeletons, but it could help us locate a host of other things — abandoned mine shafts, pipes or cables, aquifers, and any variety of underground irregularities. Knowing where things are underground before they start to dig could help companies save millions of dollars during construction of anything from subways to skyscrapers.
How can atoms help? Just like the Sun and the Earth, the things around us have a gravitational pull — albeit a much smaller one. Dense matter like a vein of granite would have a greater gravitational pull than would an empty subway tunnel. The difference may be tiny when measured from above the ground, but a precise enough sensor could detect it.
Using atoms as quantum sensors, a group at the University at Birmingham illustrated how precise such sensors can be. They placed two atoms in a gravitational field, giving one a little “kick” upward. This atom fell back down under the force of gravity. Because particles can act as waves, the two atoms get in each other’s way, creating an interference pattern. Two crests of the atom waves may align, causing constructive interference. Alternately, a crest may align with a trough, causing destructive interference. A tiny difference in gravity would change the interference pattern of the atoms, allowing for minute measurements in the gravitational field.
Not only can this let us know what is under our feet, but it can also help us predict when volcanoes will erupt. Magma filling an empty chamber under a volcano will change the local gravity. Sensors distributed over a volcano might be able to sense when a chamber is filling, and hopefully give advance warning before an eruption.
There’s no time like quantum time
Atomic clocks are another example of quantum sensors that can generate extreme precision. These clocks rely on the quantum nature of atoms. For starters, all electrons in an atom have some energy. Imagine the electron orbiting the nucleus at a certain distance. The electron can only orbit at discrete states separated by highly specific energy levels. To shift from one energy level to another, the electron can either absorb a photon of a precise frequency to move up, or emit a photon to move down. An atomic clock works when an electron changes its energy state around the atom.
Right now, the standard time of the United States is determined by a cesium atomic clock at the National Institute of Standards and Technology. This clock is so accurate that it will neither gain nor lose a second in 100 million years. To measure time with such accuracy, the clock uses a laser beam to shower cesium atoms with extremely precise frequencies of light, kicking their electrons to higher levels. The precise calibration of the laser’s light frequency is what allows time to be obtained. (Remember that frequency is the inverse of time.)
We can do even better if our atoms aren’t working on their own, but are instead entangled with one another. In 2020, a team at MIT made an atomic clock using entangled atoms. The accuracy of this clock is truly mind-blowing: It loses only 100 milliseconds over the age of the Universe.
From the very small to the very big
Quantum sensors can allow our telescopes and microscopes to show us more.
Normally when we think about exploring the Universe, we imagine a telescope that collects photons — whether they be optical, infrared, or radio. But we can also explore the Universe using gravitational waves.
When a pair of black holes merge or a supernova explodes, the fabric of space and time itself is stretched and squeezed like ripples on a pond. We can detect these ripples using an interferometer, which precisely compares the distance for two perpendicular directions. To measure this, the instrument sends a beam of light down each axis. The beams bounce off mirrors, return to the source, and recombine, creating an interference pattern. If a ripple from a gravitational wave passes the interferometer in one direction it might be stretched slightly, while from the other it would be squeezed, causing the interference pattern to change. This difference is small, but it would indicate the passage of a gravitational wave.
Here, again, entangled photons can offer an advantage. The interferometer’s ability to measure is limited by the difference in arrival times of photons within the beam of light. Put simply, some of the photons arrive earlier to the detector than others. By combining entangled photons and a technique called “photon squeezing” with the Heisenberg Uncertainty Principle, we can reduce the spread in these photons’ arrival times at the expense of another observable. Using this method, interferometers like LIGO and Virgo can detect vibrations 100,000 times smaller than an atomic nucleus.
Squeezing light can also help improve the sensitivity of microscopes. In order for a microscope to work, light must illuminate the subject. As that light bounces off the sample and returns to the microscope, randomness in photon arrival time introduces noise. Normally, this shot noise, as it is called, can be reduced by increasing the brightness. But at some point, the intensity of light actually damages the sample, especially if it is biological tissue of some sort. A team at the University of Queensland showed that using entangled photons and squeezing them increased the sensitivity of the microscope without frying the sample.
Measurement is about understanding our environment at a deeper level. Whether they be of temperature, electrical field, pressure, or time, such measurements are about more than numbers. They are about understanding what those numbers mean and how to use small changes. Quantum sensors can be used in MRIs and in navigating without GPS systems. They can help self-driving cars better sense their environment and scientists predict volcanic eruptions. Quantum entanglement may remain mysterious, but it has a very practical side, too.