Ask Ethan: What Is Energy?
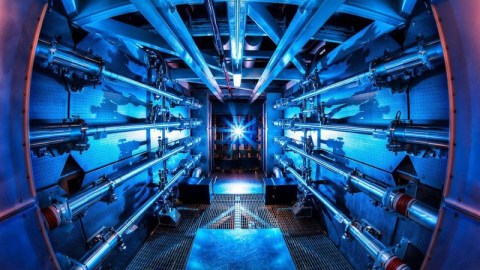
We speak about it, argue over it, and even fight wars for it. We know it when we see it. But just what is energy, anyway?
When it comes to being a human on planet Earth, energy affects practically every aspect of our lives. The energy content of a room determines its temperature; the ability to use it in a directed fashion is how we transport ourselves; we harness it to cook our food; the energy we burn in our bodies is necessary to keep us alive. From the energy of motion to stored energy to distributing or conserving it, energy affects all aspects of our lives. But even defining what energy is can be an awfully big challenge. That’s why Raza Usman asked, for this edition of our Ask Ethan column:
We talk about energy and we know that there are various forms of energy (PE, KE …) and you can do work with it, and it has to be conserved, and energy and matter are interchangeable, etc. But what is energy?
Physics can say a lot about energy, but even the top theoretical physicists have trouble concocting a definition that everyone can be happy with.
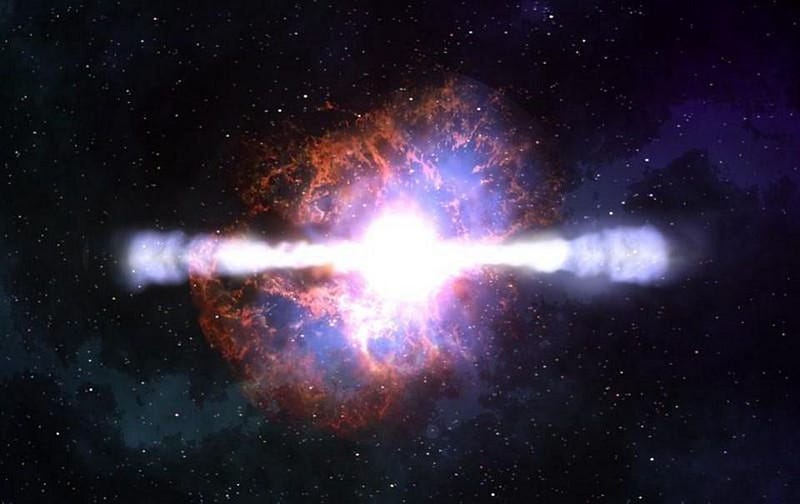
The first definition of energy that the physical definition is built off of was this: energy is the ability to do work. But work, in physics, isn’t haphazardly defined as it is in the colloquial sense. Instead, work means something very specific: a force applied to an object that moves a certain distance, in the same direction the object moves.
If you push on a box with a force of 10 N in the same direction that the box moves a distance of 1 meter, you do 10 J of work.
If you push on a box with a force of 10 N in the opposite direction that the box moves a distance of 1 meter, you do -10 J of work.
And if you push on a box, with a force of 10 N, perpendicular to the direction it moves 1 meter, you do no work at all.
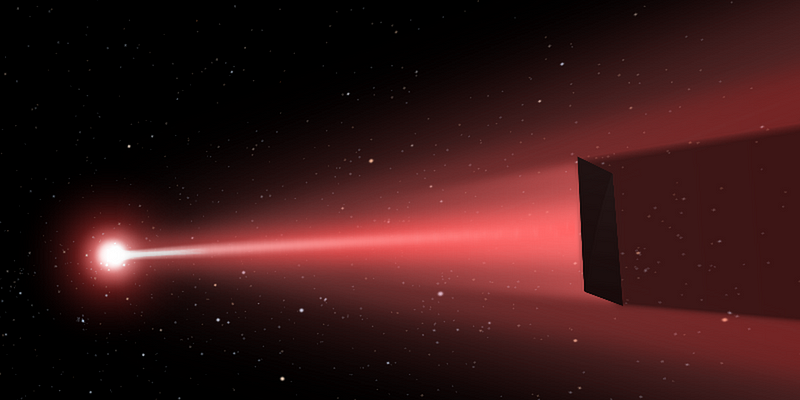
Traditionally, all other definitions of energy rely on the ability to transform into this: the ability to do work. Energy is defined by your ability to do work, but work is (circularly) defined as the transfer of energy from one source to another. Despite our ignorance, however, there are plenty of things we can confidently say about energy that are non-controversial, including:
- all mass and matter contains it,
- it can be quantified,
- we can store it electrically, chemically, thermally, sonically, etc.,
- we can convert it from one form to another,
- we can use it to accomplish things (i.e., to do work),
- we neither create nor destroy it,
- and we can generate, calculate, and measure its various forms.
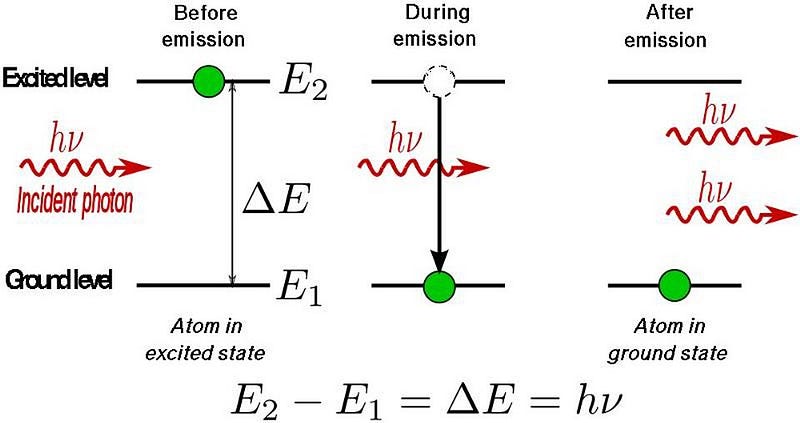
As far as the various forms of energy go, there is really no limit. If you have any configuration from which energy can be extracted, transferred, or from which work can be done, you’ve got a new form of energy. This can be mechanical, electrical, or chemical; it can be in a kinetic (moving) or potential (unreleased) form; it can be in the form of heat or light; it can be particle-based or wave-based; it can be classical or quantum in nature.
But energy can’t always be extracted. Along with all of these different forms, physics also gives you this idea of a ground state, or a lowest-energy state that any quantum system can achieve. This zero-point energy is not necessarily equal to the classical value of a zero-energy state, but can often be a finite, non-zero value. For example, the energy of a hydrogen atom in the lowest (ground) state isn’t zero, but a larger value.
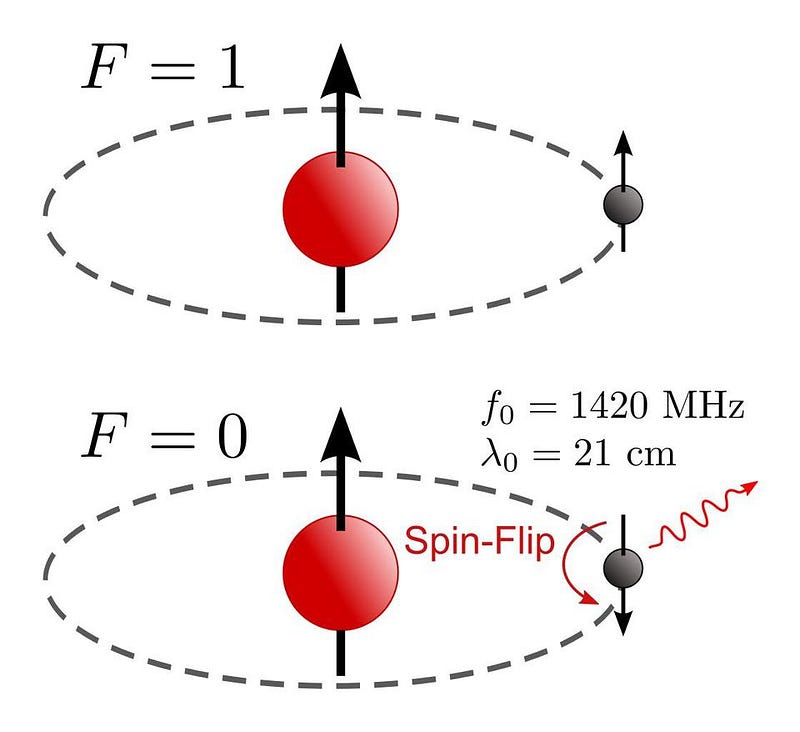
That difference, between the ground state and the classical value of zero, defines what we know of as zero-point energy. In perhaps the most stunning discovery in the history of physics, studies of the expanding Universe have, for the past 20 years, led scientists to the conclusion that the zero-point energy of space itself is not zero, but some larger, finite value.
Remember the original definition of energy: that it’s the ability to do work (exert a force along the direction of motion). If space itself is filled with some sort of energy, known today as dark energy, then it exerts a negative pressure, which is a force over an area. And if the Universe is expanding, that means the surface area of the observable Universe’s boundary is changing by a certain distance. Therefore, dark energy does work on the expanding Universe itself.
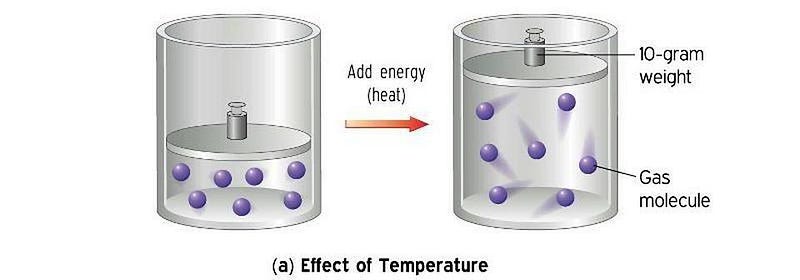
But how is this okay? It seems like a Universe filled with dark energy doesn’t conserve energy. If the energy density — energy-per-unit-volume — remains constant, but the volume of the Universe is increasing, doesn’t that mean the total amount of energy in the Universe is increasing? And doesn’t that violate the conservation of energy?
Here’s where we start encountering problems. You see, I lied to you a little bit, when I talked about dark energy exerting a force that works against the Universe as it expands. The truth is more complex and counterintuitive, but boils down to this: in an expanding Universe, energy is not conserved. In fact, in an expanding spacetime under the laws of General Relativity, energy isn’t even, at a global level, defined at all.
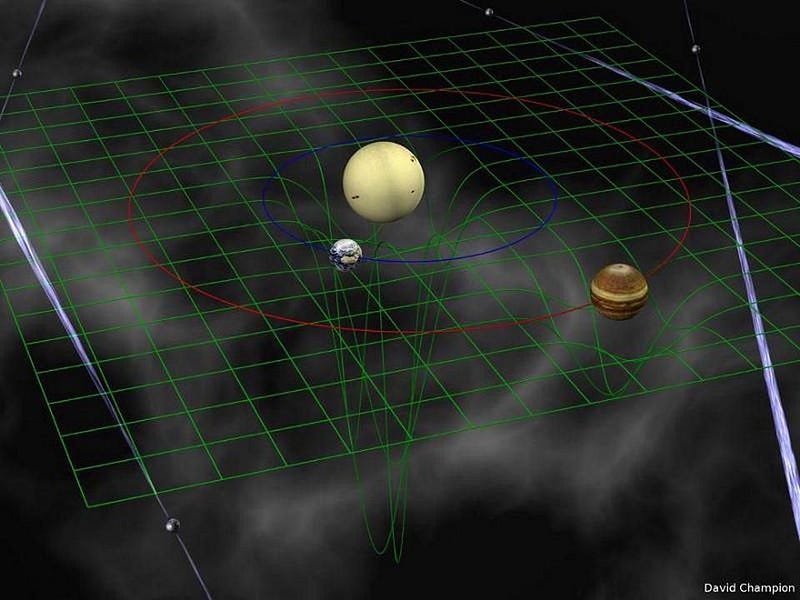
The two major takeaways are as follows:
- When particles interact in an unchanging spacetime, energy must be conserved. When the spacetime they’re in changes, that conservation law no longer holds.
- If you redefine energy to include the work done, both positive and negative, by a patch of space on its surroundings, you can save the conservation of energy in an expanding Universe. This is true for both positive-pressure quantities (like photons) and negative pressure ones (like dark energy).
But this redefinition is not robust; it’s simply a mathematical redefinition we can use to force energy to be conserved. The truth of the matter is that energy is not conserved in an expanding Universe.
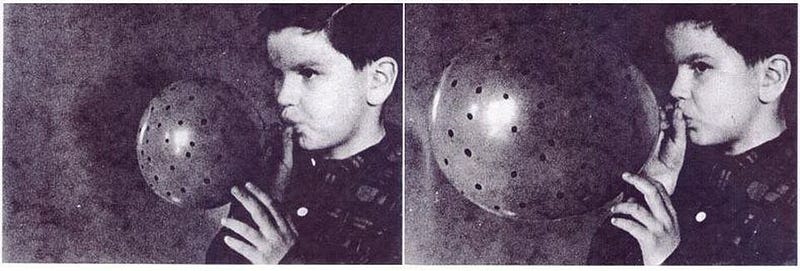
So, this brings us back full circle to the original question. What is energy? As best as we know it, energy cannot exist independently of particles or systems of particles. (Even gravitational waves are made of theoretical particles known as gravitons, just as electromagnetic waves are made of photons.) Energy comes in a variety of forms: some fundamental and some derived.
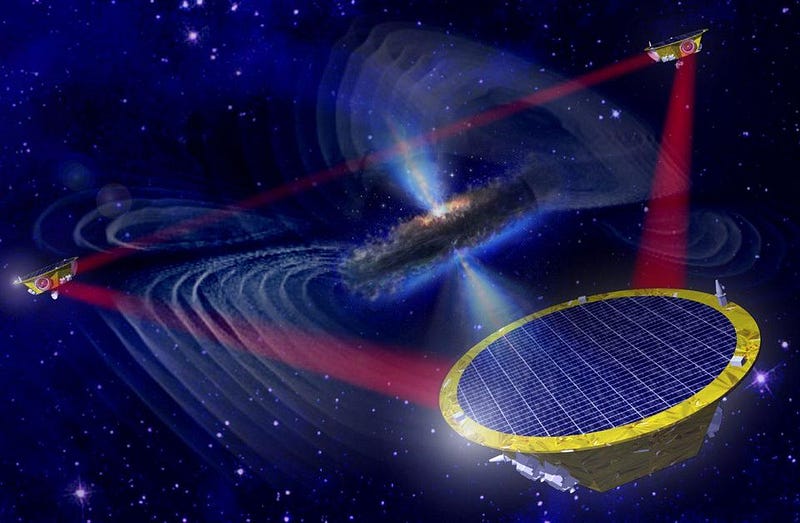
A particle’s rest mass energy, for example, is inherent to every particle in the Universe itself. But all other forms of energy that exist are relative. Kinetic energy is relative; electric energy is stored relative to other charges; chemical energy relies on breaking and forming bonds. An atom in an excited state has more energy than an atom in a ground state, but that energy can only be released through the emission of a photon.
You cannot make that transition from one energy state to another without conserving energy, and that energy needs to be carried by a particle.
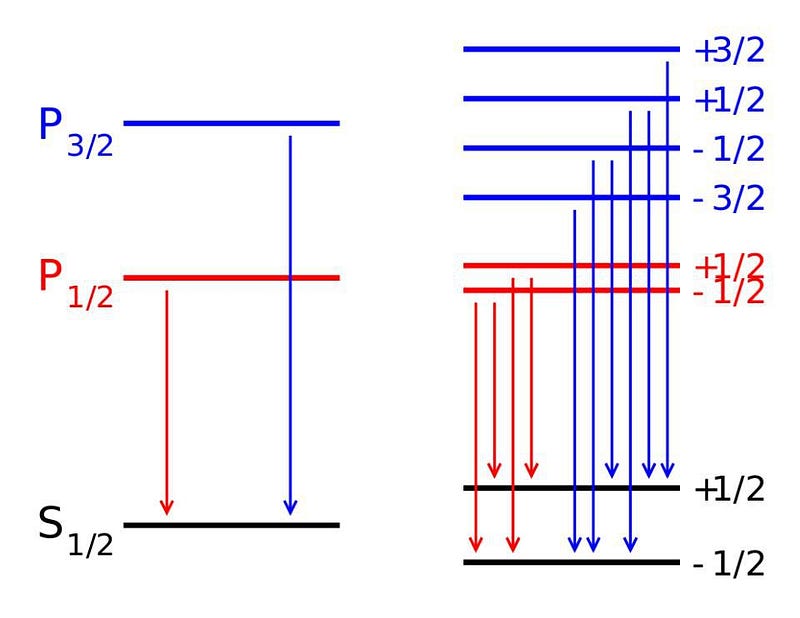
As far as we can tell, energy is not something we can isolate in a laboratory, but only one of many properties that matter, antimatter and radiation all possess. Energy can only be defined relative to another, somewhat arbitrary state. and is entirely dependent on the full suite of particles that make up your system. It has been over 300 years since physics introduced the work-related definition of energy, and while we still use it for everything that transitions, it doesn’t apply universally.
A little over a century ago, the esteemed physicist Henri Poincaré noted the following, “science is built up of facts, as a house is built of stones; but an accumulation of facts is no more a science than a heap of stones is a house.” We speak all the time of what energy can do, how it’s used, where it appears and in what quantities, and how to accomplish a myriad of tasks with it. But a fundamental, universal definition? That’s an accomplishment that’s still beyond our reach.
Send in your Ask Ethan suggestions to startswithabang at gmail dot com!
Ethan Siegel is the author of Beyond the Galaxy and Treknology. You can pre-order his third book, currently in development: the Encyclopaedia Cosmologica.