This Is Why ‘Multi-Messenger Astronomy’ Is The Future Of Astrophysics

Even before MMA was a combat sport, it was a unique type of astronomy. Today, it’s opening up the Universe as never before.
On February 24, 1987, a spectacular signal was seen as never before. From 165,000 light-years away, the first signals from a recently destroyed star — a core-collapse supernova — arrived on Earth. Humans had witnessed supernovae before, both within the Milky Way and in galaxies beyond our own, but this one was special. The first hint of its arrival didn’t come in the form of light, but rather in a signal never measured before: in the form of neutrinos.
It wasn’t until hours later that the light arrived, corresponding to the extra time it took the shock wave occurring in the star’s interior to reach the surface. Whereas light interacts with the material composing the progenitor star, neutrinos simply pass right through it, giving them a significant head start. For the first time, an astronomical event beyond our Solar System had emitted both light and particles that were observed on Earth. The era of Multi-Messenger Astronomy was born. Although it’s still a term that few non-astronomers are familiar with, it truly is the future of studying the Universe.
Originally, astronomy was confined to a very narrow regime: the only signals we were capable of receiving were in the form of visible light. Since that’s what our eyes had adapted to see, those were the tools we had at our disposal to examine the Universe. For countless millennia, human eyes viewed the Sun, Moon, planets, stars, and the fuzzy, distant nebulae we now know to be galaxies as they slowly but surely migrated across the sky.
Even after the invention of the telescope, astronomy was still confined to what we could perceive in visible light. All the telescope did, essentially, was to enhance our light-gathering power by using mirrors and/or lenses to increase the light-collecting area far beyond the limits of even the most thoroughly dilated pupil. Instead of thousands of stars, these tools would reveal hundreds of thousands, millions, and eventually billions of them.
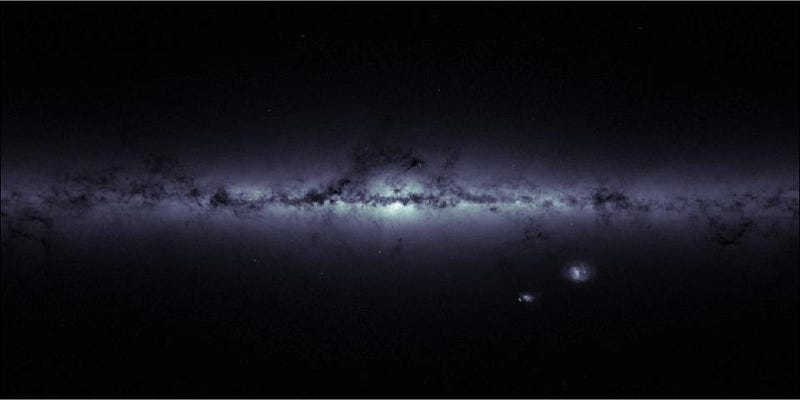
Early on, only the brightest objects appeared to have color features; the others were so far away that only monochrome signals were perceptible. When photographic techniques became available and were applied to astronomy, however, it became possible to place a color filter over the telescope, recording only light of a particular wavelength.
When multiple different wavelengths were sampled either at once or in rapid succession, the data that was collected could be combined to form a single color image. This technique was originally applied to terrestrial images, but was extended to astronomy in short order, enabling scientists to produce color images of objects in the night sky. Even today, the field of astrophotography is enjoyed by not only professionals, but tens of thousands of amateurs and hobbyists from across the world.
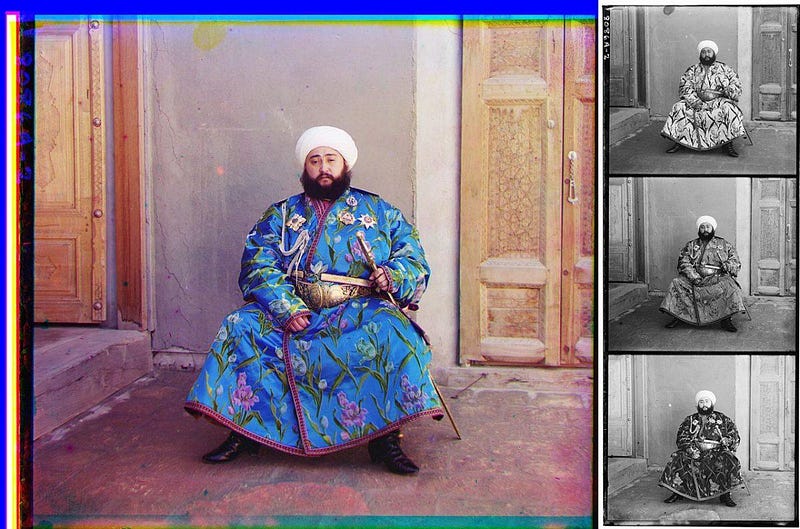
Still, this advance only leveraged the tiniest portion of the electromagnetic spectrum: visible light. In reality, there are many forms of light that are both higher in energy (and shorter in wavelength) as well as lower in energy (with longer wavelengths) that can be perceived and measured by the right type of telescope.
Today, we take advantage of all the different forms of light that there are to study the objects present in the Universe.
- Gamma-rays and X-rays reveal high-energy objects like pulsars, black holes, and transient “burst” events,
- ultraviolet, visible, and near-infrared light reveal stars and star-forming material,
- mid-infrared and far-infrared light shows the presence of cooler gas and dust,
- while microwave and radio light reveals jets of particles, diffuse background emissions, and details in individual protoplanetary disks.
Whenever we look at an object in a different wavelength of light, we have the potential to reveal an entirely new class of information about it.
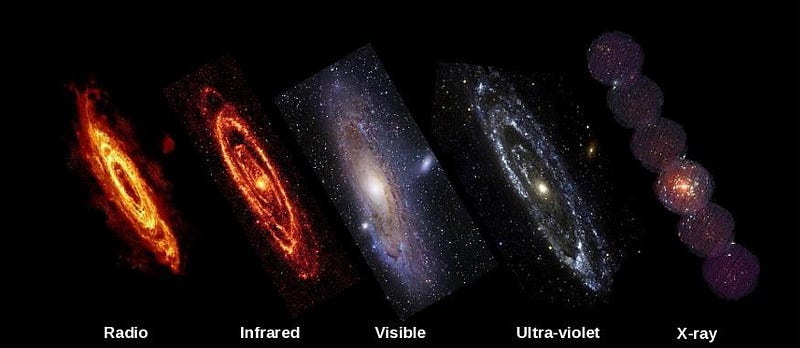
Even though we have different names for these various types of astronomical observing — some of what we observe are rays (gamma-rays and X-rays), some are light (ultraviolet and visible), some are radiation (infrared) and some are waves (radio) — they’re all still light. From a physics point of view, we’re collecting the same thing: photons, or quanta of light. We’re just looking at light with different properties when we’re doing any of these types of astronomy.
In other words, doing astronomy by collecting light of any type always involves the same type of messenger: the same type of information-carrier. However, there are other forms of astronomy, too, because the objects in the Universe doesn’t just emit light. As they undergo all the various astrophysical processes that the Universe allows, they can emit a wide variety of classes of signal, including from fundamentally different messengers.
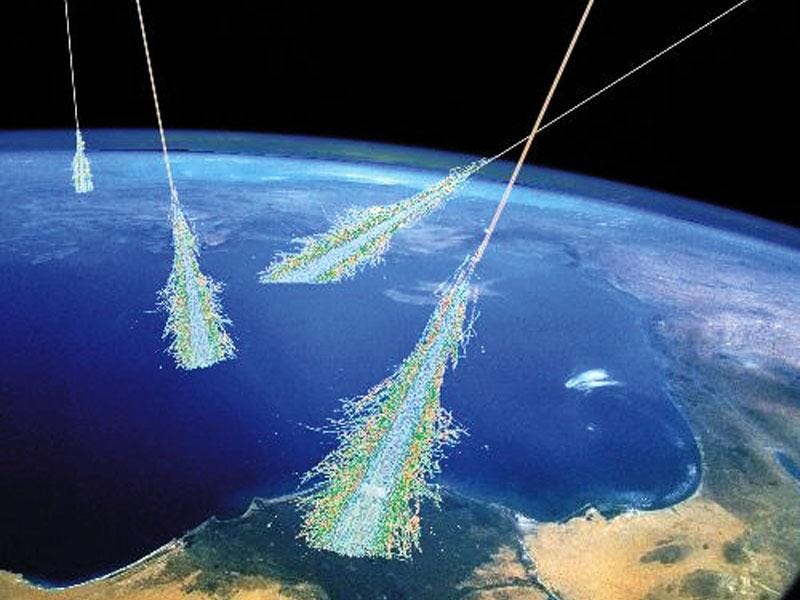
Numerous classes of objects don’t merely emit light, but also particles. From all over the sky, including from the Sun, we detect a wide variety of cosmic ray particles, including:
- electrons,
- positrons (the antimatter counterpart of electrons),
- protons,
- anti-protons,
- neutrinos and anti-neutrinos,
- and even heavier, complex atomic nuclei, from helium all the way up to iron.
We’ve been collecting these types of particles from within the Solar System for extremely long periods of time, as arguably every time we encounter a meteor shower, we’re witnessing particle showers in our atmosphere originating from past-and-present comets. The Sun emits a wide variety of cosmic rays. And recently, with sophisticated observatories like Kamiokande (and its successors) and IceCube, we’re detecting both solar and cosmic neutrinos.
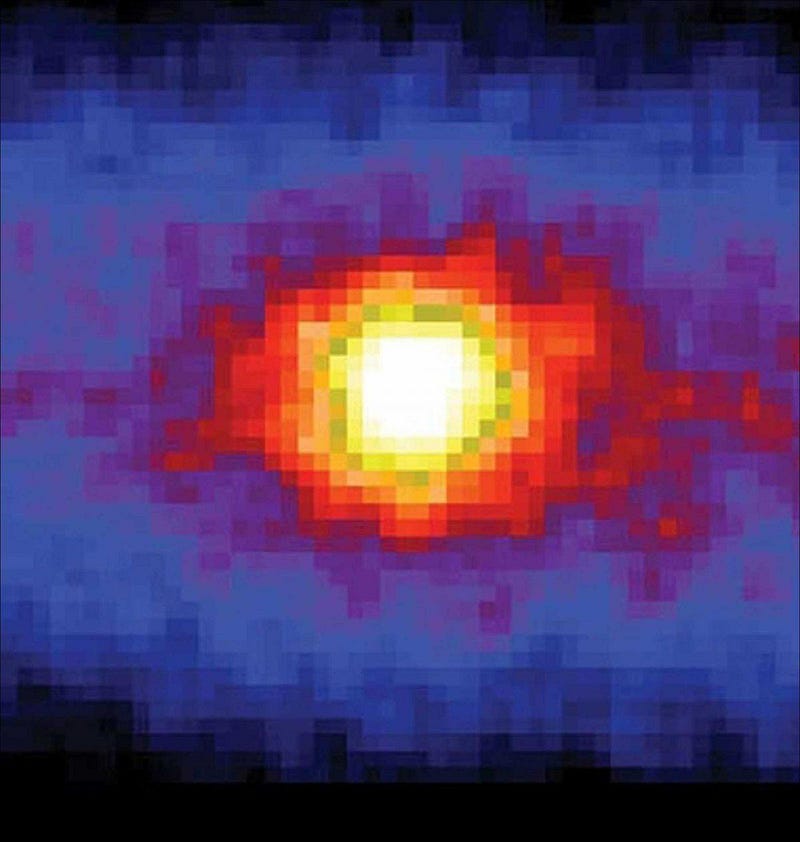
Light and particles are each a completely independent type of “messenger” in astronomy, as they require fundamentally different techniques, equipment, and interpretations in order to make sense of the Universe. But the 2010s brought us something even more remarkable: a third type of fundamental messenger. On September 14, 2015, the first new signal arrived: in the form of gravitational waves.
Gravitational waves are the only signal ever directly detected that has no type of known, measured, Standard Model particle associated with it. They are generated whenever a mass accelerates through a region of space that changes in its curvature, but it’s only the strongest, largest-amplitude signals of a specific frequency that we’re able to detect. Using a large, extraordinarily precise laser interferometer, scientists are able to detect gravitational waves that correspond to a change in those arm lengths of no more than 10^-19 meters: about 1/10,000th the width of a proton.
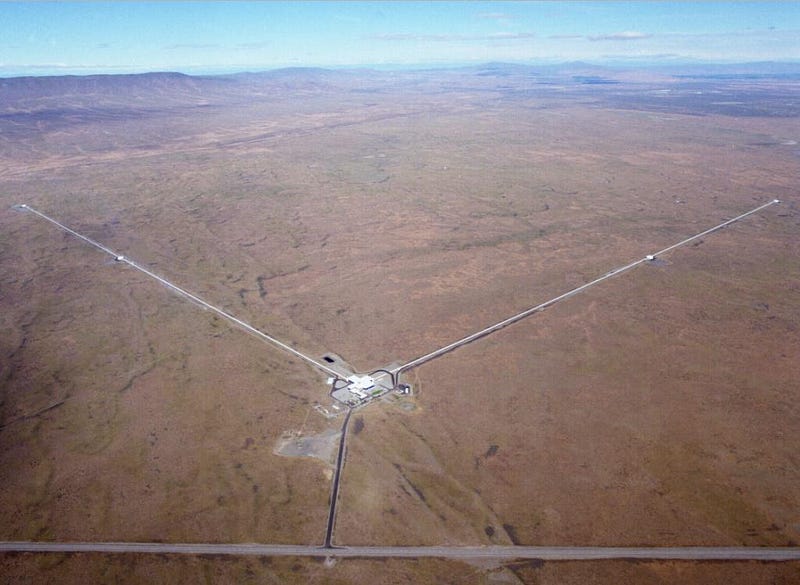
With three fundamentally different types of astronomy, we’ve gained new windows on the Universe and new methods of gaining information about all that’s out there. Light, particles, and gravitational waves are intrinstically different types of messengers for astronomers, with each class of signal revealing information about the Universe that the other two cannot.
But the most powerful examples of these various astronomical techniques occur when we’re able to use more than one of them at the same time. When astronomers use the term “Multi-Messenger Astronomy,” this is the key concept they’re referring to: detecting the same object or event with either light and particles, light and gravitational waves, particles and gravitational waves, or all three together. As the sciences of traditional (light-based) astronomy, gravitational wave astronomy, and cosmic ray astronomy all advance, these multi-messenger events will reveal the Universe as never before.
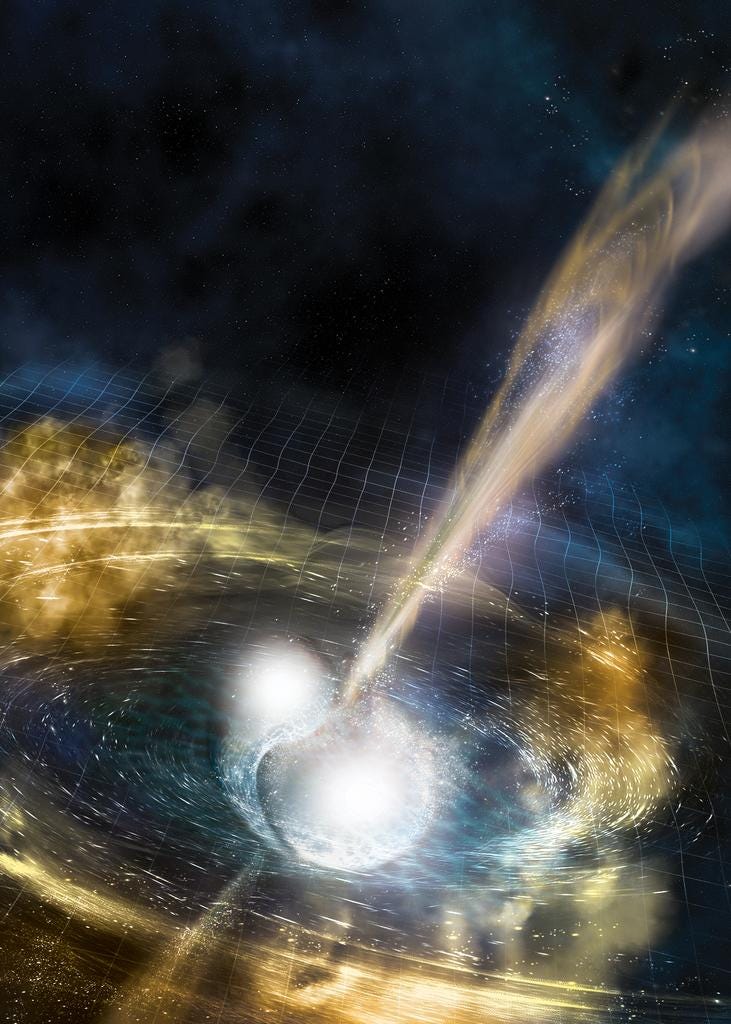
In 2017, gravitational wave astronomers observed a signal unlike any other, which wound up corresponding to the merger of two neutron stars some 130 million light-years away. Almost simultaneously — just two seconds after the gravitational wave signal ceased — the first electromagnetic signal (in the form of gamma-rays) arrived. The first robust multi-messenger signal involving gravitational waves had been detected.
This is only going to get better with time and improved technology. When the next nearby supernova occurs, we’ll certainly be able to detect both light and particles, and might even get gravitational waves, too. In fact, we had a candidate (that didn’t pan out) for our first trifecta signal earlier this year. When a pulsar glitch is picked up by a gravitational wave detector, it will also be a multi-messenger signal. And when LISA, our next-generation gravitational wave detector comes online, we’ll even be able to predict these cosmic mergers that LIGO and Virgo see today well in advance, giving ourselves plenty of lead time to make simultaneous observations of a possible multi-messenger event at that critical, “t=0” moment.
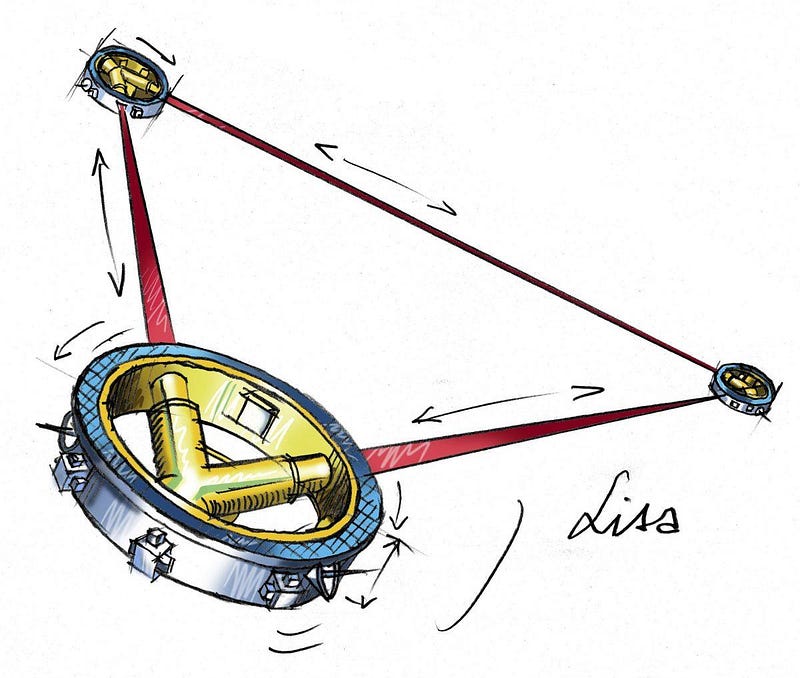
The three types of signals we know how to collect from the Universe — light, particles, and gravitational waves — all deliver fundamentally different types of information right to our front door. By combining the most precise observations we can take with each of these, we can learn more about our cosmic history than any one of these signal types, or “messengers,” can provide in isolation.
We’ve already learned how neutrinos are produced in supernova, and how their travel path is less impeded by matter than light’s is. We’ve already linked merging neutron stars with kilonovae and the production of the heaviest elements in the Universe. With multi-messenger astronomy still in its infancy, we can expect a deluge of new events and new discoveries as this science progresses throughout the 21st century.
Just as you can learn more about a tiger by hearing its growl, smelling its scent, and watching it hunt than you can from a still image alone, you can learn more about the Universe by detecting these fundamentally different types of messengers all at once. Our bodies might be limited in terms of the senses we can use in any given scenario, but our knowledge of the Universe is limited only by the fundamental physics governing it. In the quest to learn it all, we owe it to humanity to use every resource we can muster.
Ethan Siegel is the author of Beyond the Galaxy and Treknology. You can pre-order his third book, currently in development: the Encyclopaedia Cosmologica.