Is the Universe 13.8 or 26.7 billion years old?
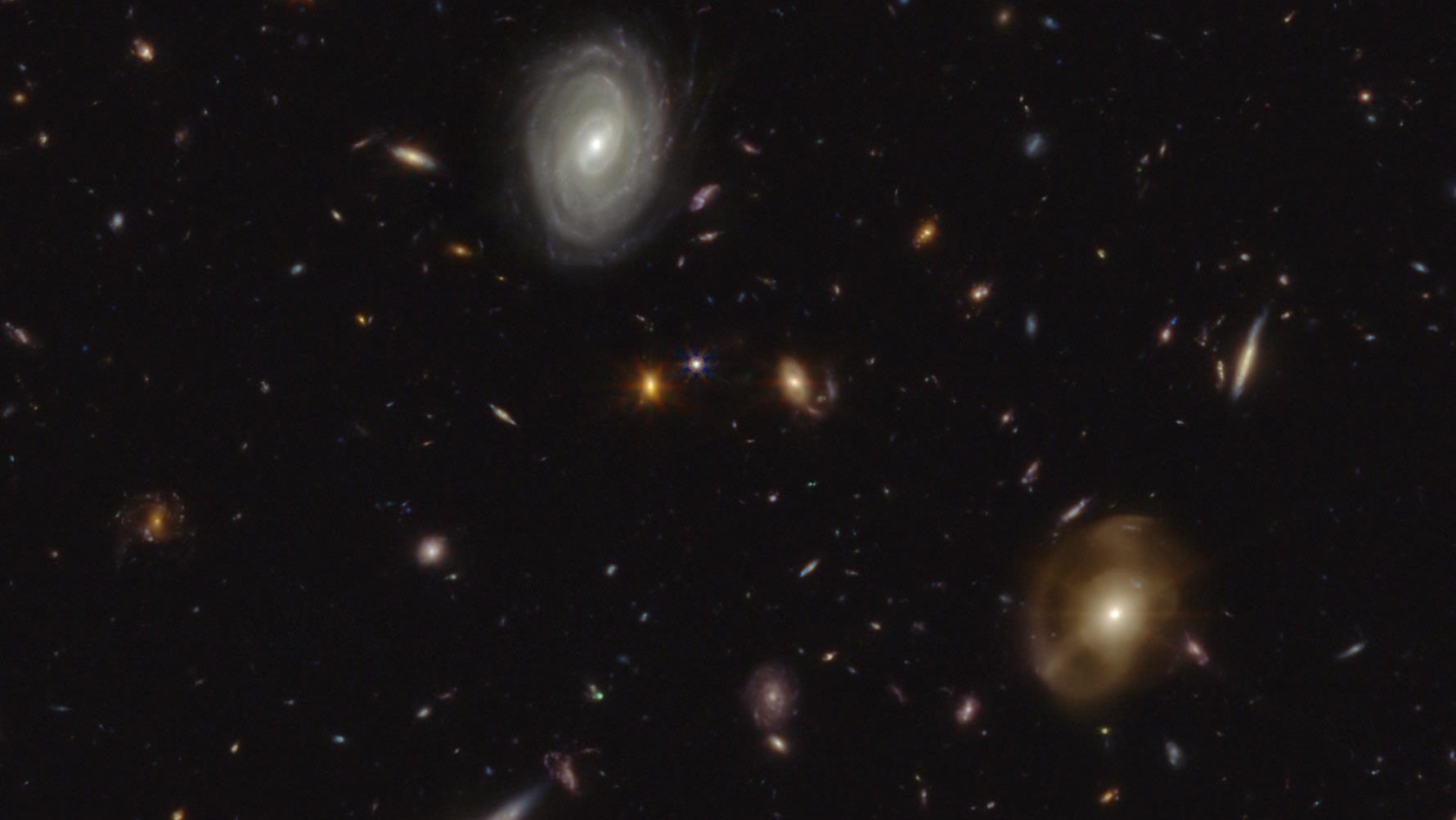
- According to the “Standard Model” of cosmology, the Universe is 68% dark energy, 27% dark matter, 5% normal matter, and is 13.8 billion years old: as measured since the hot Big Bang.
- Recent observations from the James Webb Space Telescope (JWST), however, have found numerous galaxies that appear early, but look surprisingly grown-up.
- A new theory claims to solve this “early, grown-up galaxies” problem by changing the age of the Universe to 26.7 billion years old. Here’s how scientists will decide.
Perhaps the greatest achievement of late-20th and early-21st century astrophysics is the arrival of a “consensus model” of the entire Universe: Lambda-CDM (ΛCDM) cosmology. For countless generations of humans, we’ve marveled at questions such as:
- What is the Universe?
- What makes it up?
- How far, to the limits of what it’s possible to observe, does it go on for?
- How did it come into existence, and how long ago?
- How did it grow up to be the way it is today?
- And what will its ultimate fate be?
Today, after unprecedented measurements of galaxies all throughout cosmic history, all-sky imaging of the Universe at microwave wavelengths, and thousands upon thousands of supernovae and other transient events all across the Universe, we finally have our answers to these questions. Our Universe, made of 68% dark energy, 27% dark matter, and just 5% “normal stuff,” began from a small, dense, nearly-perfectly-uniform state some 13.8 billion years ago in a hot Big Bang, and has been expanding, cooling, and gravitating ever since.
At least, that’s the consensus picture. Recently, a challenge to that picture has gotten some popular attention, based on a recently published paper claiming that the Universe is actually 26.7 billion years old, not 13.8 billion years old. Let’s look at these two theories side-by-side and unpack what’s true versus what would need to be true in order to truly determine the age of the Universe.
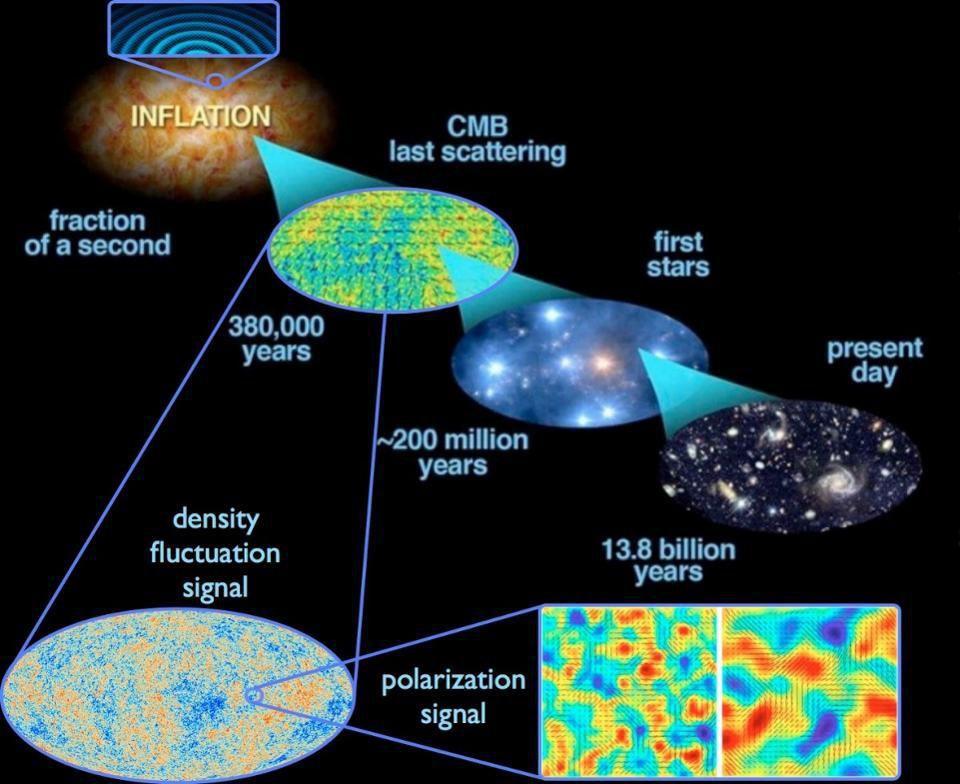
Different assumptions
Anytime you’re given a scientific theory, you have to ask yourself, “What assumptions are behind it?” In the case of the standard model of cosmology, ΛCDM, the assumptions are that:
- the laws of physics are given by General Relativity (for gravity) and the Standard Model of particle physics (for the other three fundamental forces),
- the Universe is roughly the same in all directions (isotropic) and the same at all locations (homogeneous) if you examine it on the largest cosmic scales,
- and that, in addition to the known particles that make up our conventional notions of matter and radiation, there also exists some form of dark matter and some form of dark energy, both of which contribute to the Universe’s expansion and where dark matter not only aids in, but dominates, the formation of large-scale structure.
Within General Relativity and particle physics, however, there are some unstated assumptions that are so fundamental to these theories that they’re never even talked about: that the underlying laws of physics are the same everywhere and at all times, that the fundamental constants are truly constant, and that the only effect empty space has on the light that passes through it is on the light’s wavelength: through a combination of the Doppler shift due to relative velocity, gravitational redshift due to the curvature of spacetime, and cosmological redshift due to the expansion of the Universe.
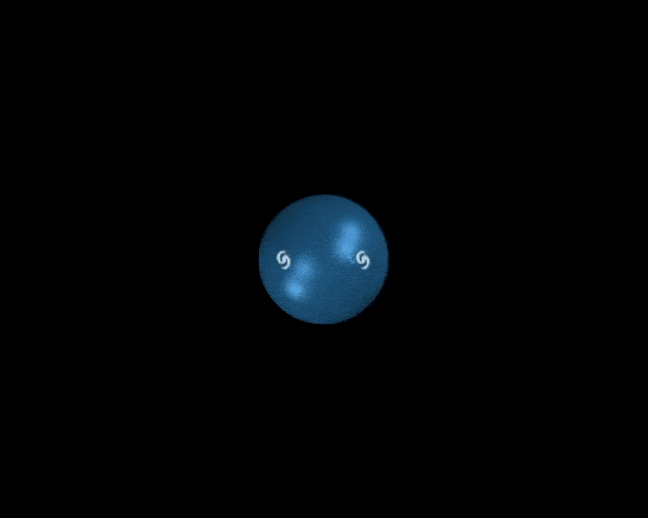
On the other hand, the new idea, put forth by controversial scientist Rajendra Gupta, keeps most of the same assumptions in place, but offers a few subtle-but-important changes.
First, instead of assuming that only the Doppler shift (the relative motions of the light-emitting source and the light-absorbing observer), the gravitational shift (the difference in the spacetime curvature between the emitting source and the absorbing observer), and the cosmological shift (as the traveling light gets stretched to longer wavelengths by the expanding spacetime it’s traveling through), Gupta also presupposes an idea first put forth by noted astronomer Fritz Zwicky back in 1929: the tired-light hypothesis, or the notion that light, as it travels through space, inherently “radiates” and loses energy as it travels, becoming “tired” before it arrives at the observer.
And second, instead of the standard assumption that the laws of physics and the fundamental constants behind them are constant with time, Gupta invokes an assumption that others have explored previously: that the fundamental constants c (the speed of light), ℏ (Planck’s constant), and G (the gravitational constant) aren’t actually constant in time, but vary. In particular, they vary in a special way — changing all together — so that the combinations of these constants that govern atomic transitions and the emission/absorption lines that we wind up observing, won’t change as we look to earlier, more distant galaxies within the expanding Universe.

Similarities and differences
One of the remarkable features of science is that, if you have multiple different models that have different underlying assumptions that go into them, there’s a scientific way to tell which one is superior. It isn’t to look at personal preference, elegance, aesthetics, or simplicity. Instead, there are two key questions that we have to evaluate.
- Which theory has fewer free parameters?
- Which theory better fits the full suite of data concerning the Universe?
The reason we look at the number of free parameters is simple: a theory that can make the same predictions as another but with fewer assumptions or required inputs is a superior physical theory to one that requires more assumptions, required inputs, or free parameters. Early on, geocentric and heliocentric models of the Solar System had the same number of free parameters, as both ideas needed to provide a series of orbital parameters to describe each planet; if a new planet would have been discovered, neither model would have been able to predict its motion without adding in those new parameters.
When Newtonian gravity came along, however, the number of “free parameters” plummeted. With an underlying force governing the dynamics of bodies in the Solar System — the force of gravity — a planet’s orbital speed, distance from the Sun, and motion through the sky were all shown to be related. This increase in predictive power with fewer free parameters is always a scientific indicator that we’re on the right track.
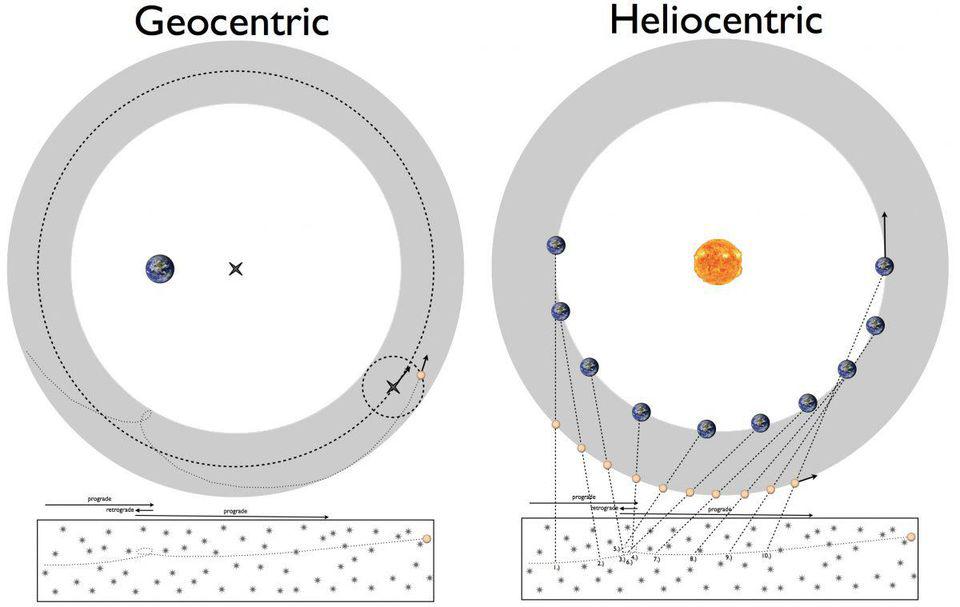
But it’s also vitally important to look at the full suite of data, as opposed to just the pieces of data that are easily fit by your model or preferred theory. In order to be considered a success, you have to consider everything that we observe on all scales, from subatomic ones to cosmic ones, is consistent with — and not in conflict with — your theory for how the Universe works.
In his paper, to his credit, Gupta looks at a few important pieces of the puzzle. He looks at the inferred distance to supernovae seen at a wide variety of cosmic distances, and shows that not only are they consistent with the standard cosmological model (ΛCDM), but also with a version of ΛCDM that includes “tired light” (what he calls ΛCDM+TL), with a model with covarying coupling constants (what Gupta calls CCC), and with a model with covarying coupling constants and tired light included (CCC+TL).
While yes, he’s including two extra free parameters in his theory as opposed to standard ΛCDM, in the form of a “tired light” component to the Universe and also in the form of a set of covarying coupling constants, this remains consistent with what we’ve observed for how distances, redshifts, and brightnesses appear in the expanding Universe.
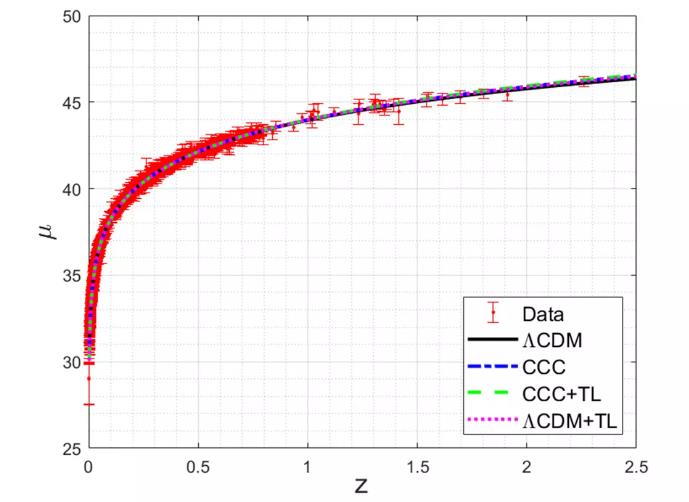
In addition, Gupta also notes that, by introducing tired light on its own in addition to the standard ingredients in a ΛCDM cosmology, he arrives at a Universe that ages much more slowly at very high redshifts (corresponding to great distances). Whereas a standard ΛCDM universe has experienced only 13.8 billion years since the hot Big Bang, a ΛCDM universe with tired light would be about 6 billion years older: up to about 19-and-change billion years old. Additionally, much of that aging would come early on. Whereas galaxies seen at the limit of Hubble and near the edge of JWST’s capabilities, at a redshift of z = 10, would be only ~400 million years old in ΛCDM, they would be about 2 billion years old in ΛCDM with tired light.
Furthermore, by introducing both covarying coupling constants and tired light, he can increase the total age of the Universe to be a whopping 26.7 billion years. At a redshift of z = 10, instead of ~400 million or even ~2 billion years, the Universe would already be about ~6 billion years old: an impressively large figure.
Gupta contends that, whereas JWST has shown us galaxies that appear brighter, more massive, and more evolved than had been expected to be seen so early on, his modified cosmology, with tired light and varying coupling constants, these galaxies suddenly fall into line with expectations.
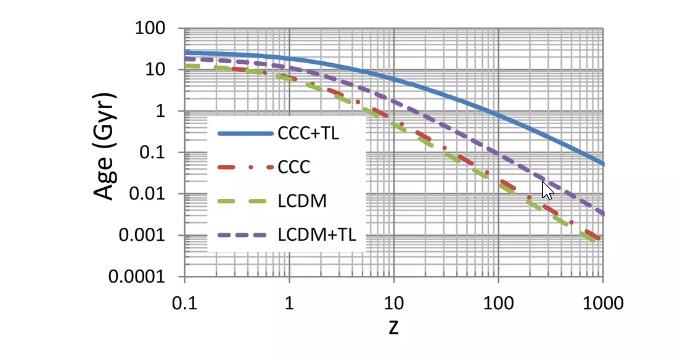
What would need to be true in order for Gupta to be proven right?
But as we said earlier, science isn’t about solely looking at the data points that favor your explanation. That’s what we call “cherry-picking,” and that’s a surefire way to lead us toward biased conclusions. There are key pieces of evidence that would show up if either “light got tired” as it traveled through the Universe and/or if “the fundamental constants have changed” as the Universe has evolved. They would show up in extremely telling ways, and we can actually list a few of them off before looking at the evidence that the Universe itself presents on these fronts. Here are four of the most major ones.
#1: Tired light would add a “blurring effect” to distant galaxies.
When Zwicky first proposed the tired light idea in 1929, it was one of the few astronomical ideas that wasn’t viable even at the moment it was proposed. The reason? Zwicky himself recognized, even prior to publishing the idea, that if there were something causing the light to get tired — i.e., something for it to interact with and that caused it to lose energy — then more distant objects wouldn’t just appear redder, as their light’s lost energy would de-boost it to longer wavelengths, but would also appear blurrier. In fact, those more distant objects would be blurred by a greater amount than observations would have permitted.
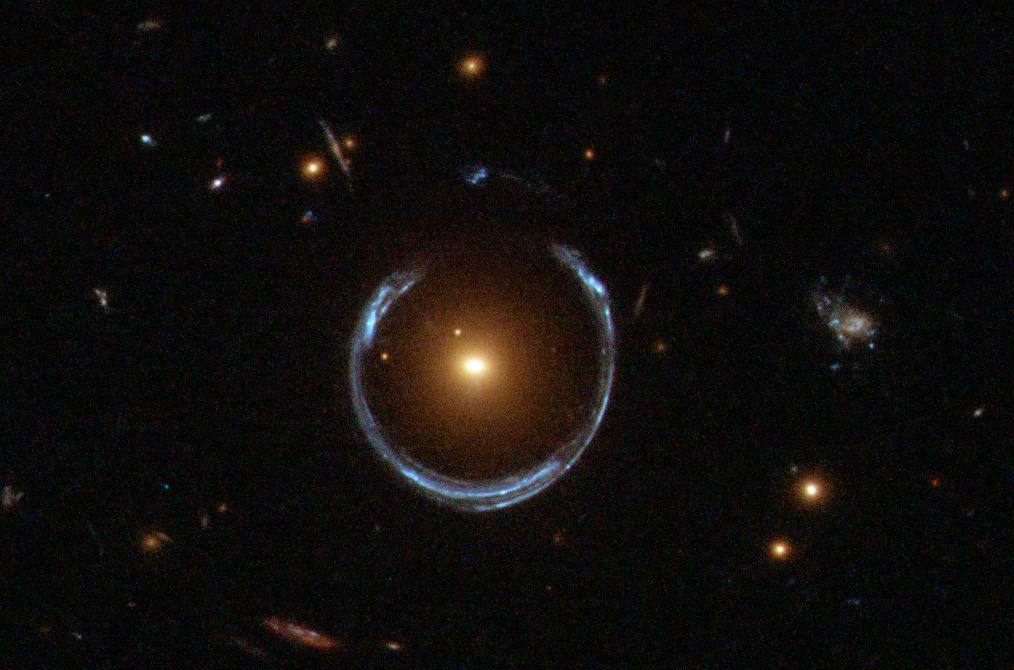
In the image above, you can see two objects: one that’s a massive foreground object at a relatively shallow redshift, and one that’s a much more distant background object at a much higher redshift. If the “tired light” scenario played any role in causing the redshift of these objects, the more distant objects would be more severely blurred. Yet in this, as well as other images that show nearby and high-redshift objects together, no such blurring has ever been observed. The Universe doesn’t get “blurrier” the farther away we look; the optical limits of our telescopes and observatories shows that ~100% of the observed redshift is cosmic.
#2: Tired light would eliminate cosmological time dilation.
Here’s a fact that we don’t often think about: the more redshifted an object’s light is, the more time it takes for the same number of emitted wavelengths to be seen by the distant observer. An object at a redshift of z = 1 would have its wavelength stretched by 100% over an object that was in the here-and-now: at a redshift of z =0. In order for the same number of “crests” and “troughs” of a wave to pass us by, because they only arrive half-as-frequently, we’d have to wait twice as long. This results in a very interesting implication: that when we look out at the distant, high-redshift Universe, we should see those distant objects exhibit a cosmological time dilation: where their clocks appear to run slow from our perspective.
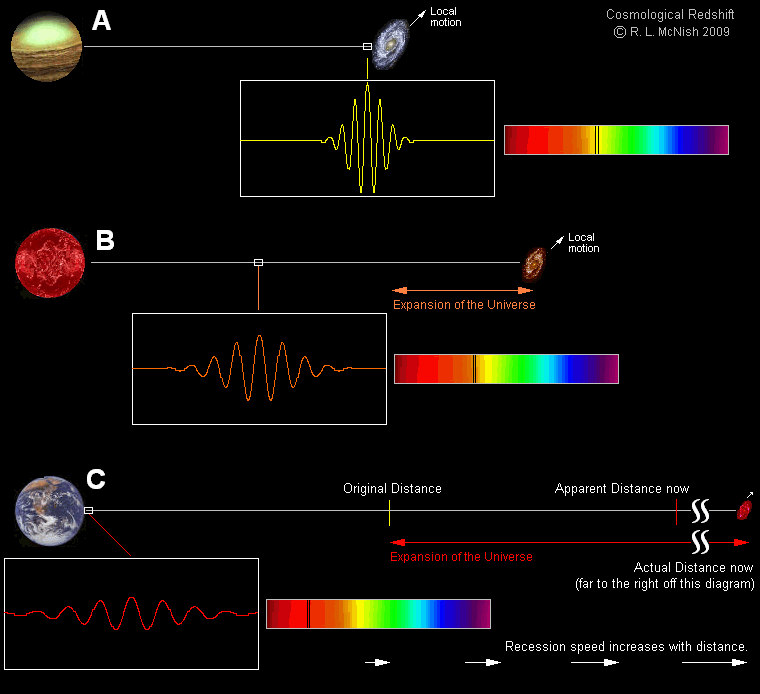
We’ve seen this for a variety of cosmic objects, including for distant supernovae, where the more redshifted a supernova is, the more its light-curve gets “stretched out” in time. This was recently confirmed at greater redshifts by looking at a class of objects, quasars, that appear to “tick” with a regular periodicity. Going all the way back to when the Universe was under 1 billion years old, 100% of the redshift, again, appears to be cosmological, and these quasars show a time dilation of exactly the amount predicted by our standard ΛCDM cosmology. With no difference observed, none of the redshift can be chalked up to “tired light.”
#3: Tired light would change the (thermal, blackbody) spectrum of the Cosmic Microwave Background.
This is a very, very big one. Whereas the galaxies we’re looking at go up to a “modest” redshift of z = 13, which is where the current cosmic record-holder presently sits, the Cosmic Microwave Background was emitted back at a whopping redshift of z = 1089, when the Universe was only 380,000 years old (according to standard ΛCDM). When light redshifts due to cosmological expansion, it maintains its blackbody character: the spectrum of how photons are distributed remains in thermal equilibrium. The number density of photons, however, has to drop to match that of a “cooler” blackbody, and in ΛCDM, it does.
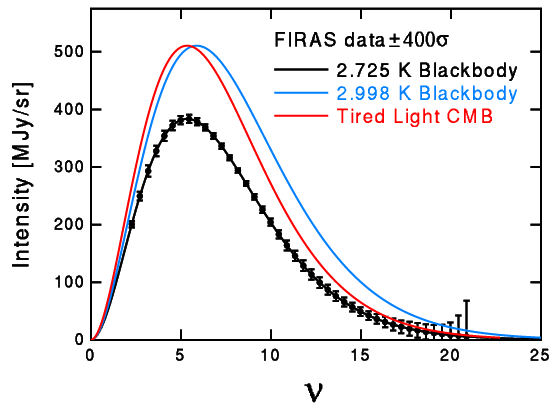
But if light were to get tired, instead, the energy of the individual photons that compose the Cosmic Microwave Background would still drop, but the number density wouldn’t change. As a result, the spectrum of “tired light” that appears as a cosmic background would not obey a blackbody spectrum.
And yet, as the above image shows (with data from NASA’s old COsmic Background Explorer mission), the Cosmic Microwave Background is the most perfect blackbody ever measured! The only way to save this aspect of “tired light” cosmology would be to observe some sort of non-blackbody component to the Cosmic Microwave Background, but to date, none has ever been observed.
#4: Evolving coupling constants wouldn’t just affect the distant Universe, but would appear in laboratory experiments here on Earth.
But there’s an independent set of constraints we can put on Gupta’s second idea: the notion that the coupling constants change over time. Atomic transitions are governed by changes in two of the fundamental constants, c (the speed of light) and ℏ (Planck’s constant), while cosmological changes are sensitive to G (the gravitational constant) as well as c and ℏ. But on Earth, we have independent ways to check these constants independently, including how they’ve evolved over time. While laboratory measurements of the electron magnetic moment, the spin-flip transition of hydrogen, and the equivalence of inertial to gravitational mass all provide good constraints, we have a far stronger one that proves the constancy of these fundamental constants over time: Earth’s only natural nuclear reactor.
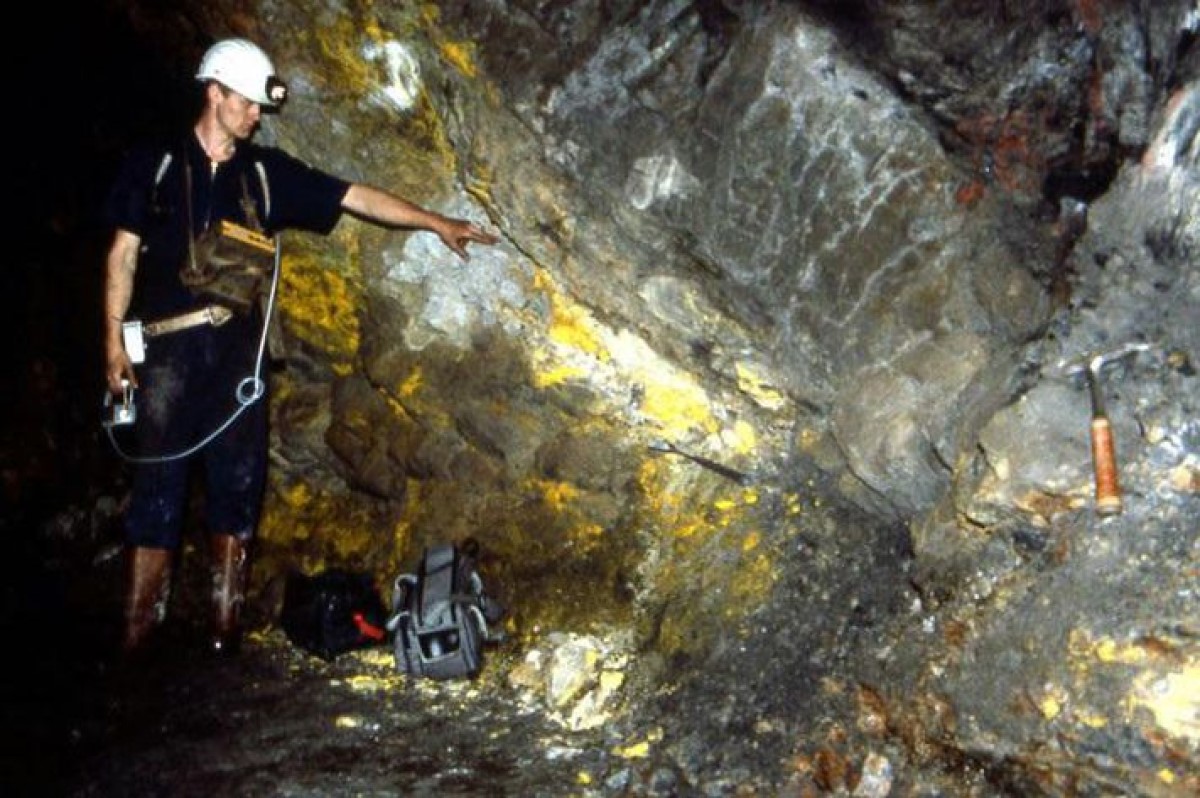
By looking at how the nuclear reactions occurred under the natural conditions that existed on Earth 1.7 billion years ago, we can determine that the fine-structure constant, which depends on the electron charge, the speed of light, and Planck’s constant, changes by less than ~0.3 parts in 10 quadrillion (1016) per year. That constraint is, quite literally, billions of times stronger than what Gupta’s “varying fundamental constant” explanation would require.
It is for these reasons, among others, that we can overwhelmingly conclude that even though Gupta’s toy model of the Universe may be fun to play with, it has no basis in reality as far as either “tired light” or “covarying fundamental constants” are concerned. Observations of the Universe, from in-focus distant galaxies to cosmologically time-dilated events to a blackbody Cosmic Microwave Background spectrum to nuclear reactors right here on Earth all show that these ideas do not correspond to our actual reality.
It might be fun to explore or theorize about, but at the end of the day, the Universe is our laboratory, and whatever it reveals to us about how nature actually behaves is what we have to go with. The Universe might not be fully understood, but its age is definitely 13.8 billion years old, and absolutely cannot be 26.7 billion years old based on the evidence at hand.