2019’s Science Breakthrough Of The Year Will Show Us A Black Hole’s Event Horizon
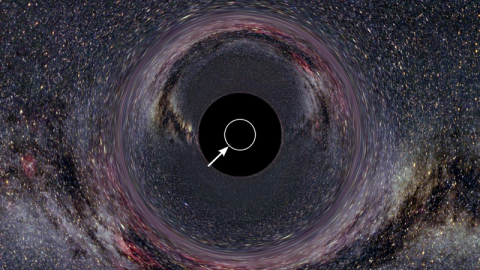
It will be the most extreme test of Einstein’s General Relativity ever. And we already have the data.
With every year that goes by, the total amount of knowledge that humanity accumulates only grows and grows. At the start of 2015, humanity had never detected a gravitational wave; at present, we’ve detected 11, and fully expect to find perhaps hundreds more in 2019. In the early 1990s, we didn’t know whether there were any planets outside our own Solar System; today, we have thousands, some of which are almost good enough to be considered Earth-like.
We’ve found all the particles in the Standard Model; we’ve discovered that the Universe is not only expanding, but accelerating; we’ve determined how many galaxies are in the Universe. But next year, something new and unprecedented is going to happen: we’re going to image a black hole’s event horizon for the first time. The data is already in hand; the rest is just a matter of time.
Black holes are fairly easy objects to detect, once you know what you’re looking for. It may seem counterintuitive, because they emit no light of their own, but they do have three surefire signatures that allow us to know that they’re there.
- Black holes create a tremendous amount of gravity — a distortion/curvature of space — in a very small volume of space. If we can observe the gravitational effects of a large, compact mass, we can infer the existence of a black hole and potentially measure its mass.
- Black holes strongly affect the environment that surrounds them. Any matter nearby it will not only experience intense tidal forces, but will accelerate and heat up, causing it to emit radiation from outside the event horizon. When we detect this radiation, we can reconstruct the properties of the object powering it, which is often only explicable by a black hole.
- Black holes can inspiral and merge, causing them to emit detectable gravitational waves for a brief amount of time. This is only possible to detect with the new science of gravitational wave astronomy.
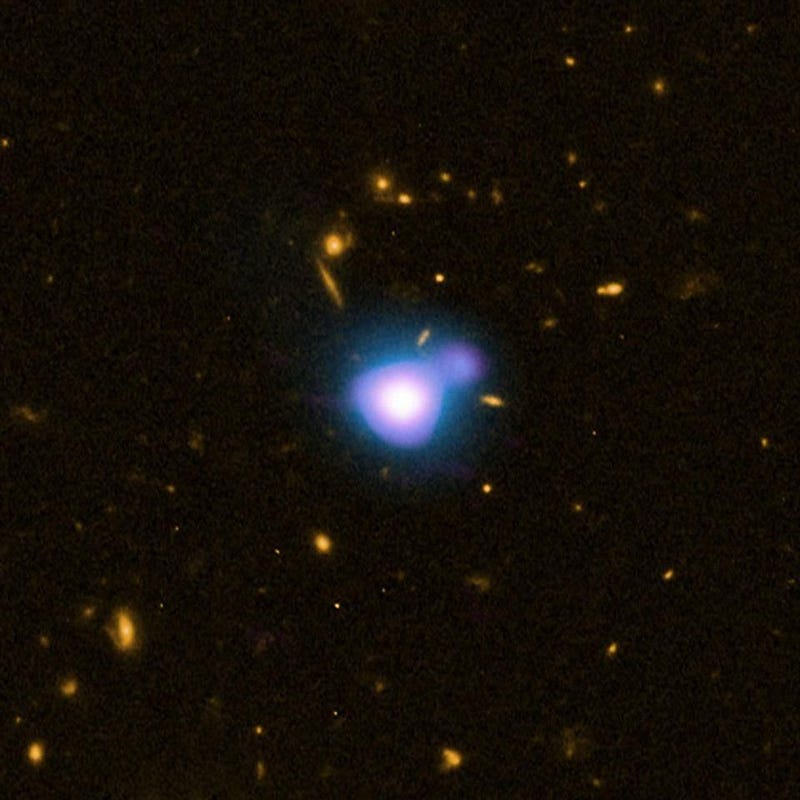
The Event Horizon Telescope, however, aims to go a step farther than any of these methods. Instead of taking measurements that enable us to infer properties of a black hole indirectly, it goes straight to the heart of the matter, and plans to image a black hole’s event horizon directly.
The method for doing so is simply and straightforward, but hasn’t been possible from a technological perspective until extremely recently. The reason why is a combination of two important factors that normally go hand-in-hand in astronomy: resolution and light collection.
Because black holes are such compact objects, we have to go to extraordinarily high resolution. But because we’re looking not for light itself, but the absence of light, we need to collect large amounts of light extremely carefully to determine where the shadow of the event horizon truly lies.
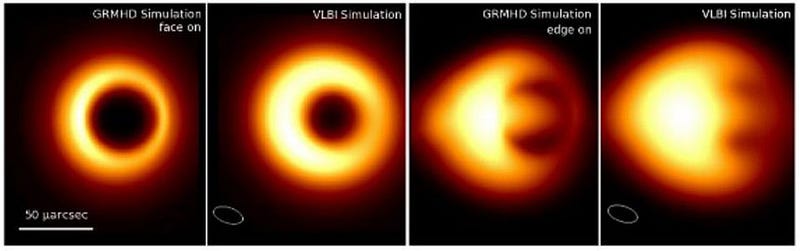
Conventionally, a telescope with better resolution and a telescope with better light-gathering power should be the same telescope. The resolution of your telescope is defined by the number of wavelengths of light that fit across your telescope’s dish, so larger telescopes have higher resolution.
By the same token, the amount of light you can gather is determined by the area of your telescope. Any photons that strike the telescope will get collected, so the larger your telescope area is, the more light-gathering power you have.
The reason the technology has been a limiting factor is resolution. The size that a black hole appears to be is proportional to its mass, but inversely proportional to its distance from us. To see the largest black hole from our perspective — Sagittarius A*, the one at the center of the Milky Way — requires a telescope approximately the size of planet Earth.
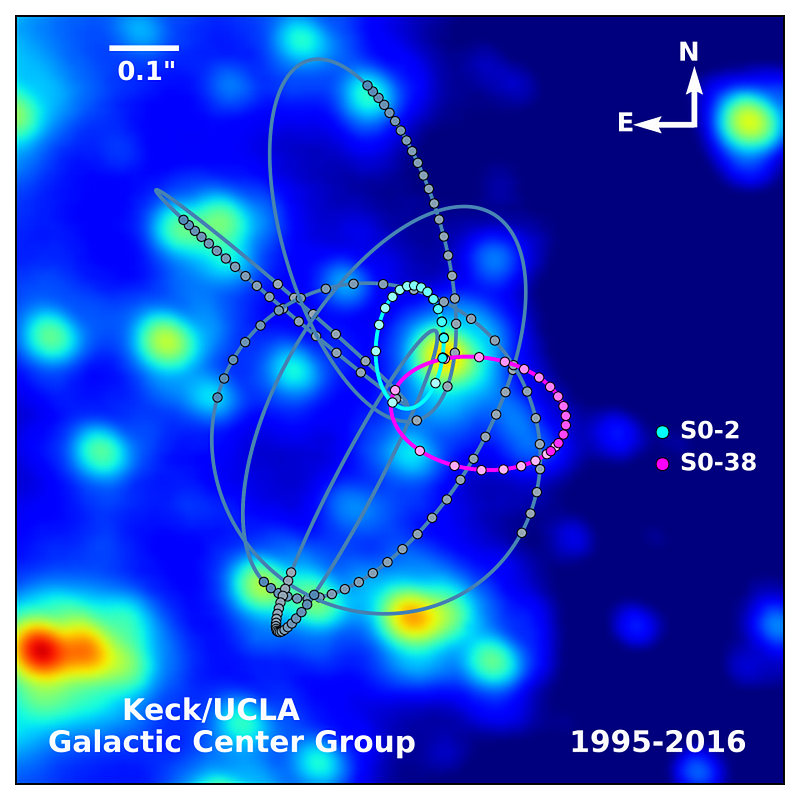
Obviously we don’t have the resources capable of building such a device! But we do have the next best thing: the capability of building an array of telescopes. When you have an array of telescopes, you only get the light-gathering power of the individual telescopes all summed together. But the resolution, if it’s done right, will enable you to see objects as fine as the spacing between the most distant telescopes.
In other words, the light-gathering is truly limited by telescope size. But resolution, if we use the technique of long-baseline interferometry (or its cousin, very long-baseline interferometry), can be vastly improved by using an array of telescopes with a large amount of space between them.
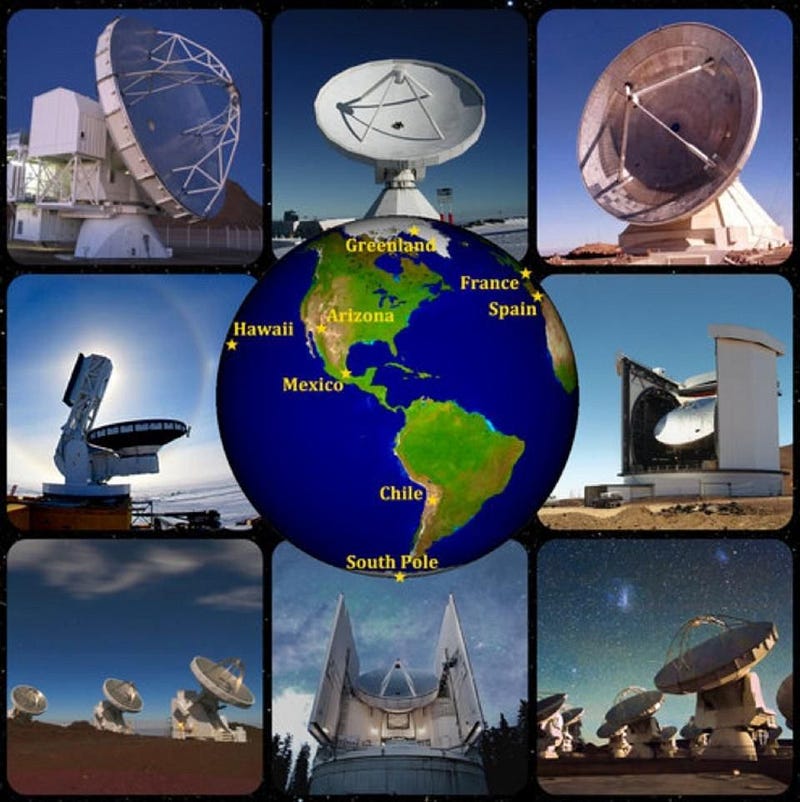
The Event Horizon Telescope is a network of 15–20 telescopes located across many different continents on Earth, from the South Pole to Europe, South America, Africa, North America, Australia, and a number of islands in the Pacific Ocean. All told, up to 12,000 kilometers separates the most distant telescopes that are part of the array.
This translates into a resolution as small as 15 microarcseconds (μas), which is how small a fly would appear to us here on Earth if it were located 400,000 kilometers away: on the Moon.
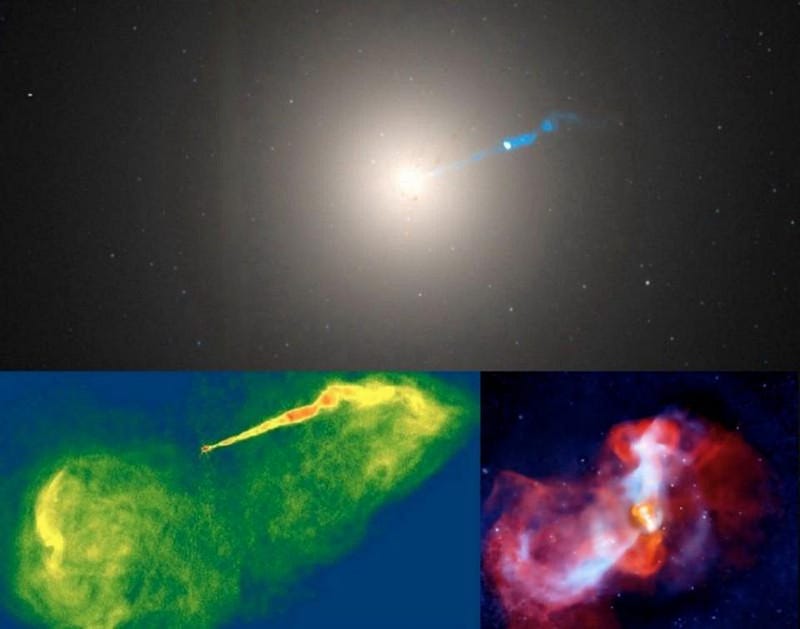
There may not be any flies on the Moon, of course, but there are black holes out there in the Universe with angular sizes that are larger than 15 μas. There are two of them, in fact: Sagittarius A* at the center of the Milky Way, and the black hole at the center of M87. The black hole in the center of M87 is located some 50–60 million light-years away, but comes in at over 6 billion solar masses, making it more than 1,000 times larger than our galaxy’s giant black hole.
The Event Horizon Telescope works by taking this enormous array of radio telescopes and observing these black holes simultaneously, which enables us to reconstruct an ultra-high-resolution image of whatever we’re looking at, so long as there’s enough light collected to see it. This concept has been demonstrated before with a variety of observatories, such as the Large Binocular Telescope, which managed to image erupting volcanoes on Jupiter’s moon, Io, while it got eclipsed by another of Jupiter’s moons!
The key to making the Event Horizon Telescope work, then, is to make sure we gather enough light to see the shadow cast by the black hole’s event horizon, while successfully imaging the light coming from around and behind it. Black holes accelerate matter, remember, and the acceleration of charged particles both creates magnetic fields and — if charged particles accelerate in the presence of magnetic fields — the emission of radiation.
The safest bet is to look in the radio portion of the spectrum, which is the lowest-energy portion. All black holes that accelerate matter are expected to emit radio waves, and we’ve seen them from both our Milky Way’s center and from M87’s center. The difference is, at these new, high resolutions, we should be able to spot the “void” where the event horizon itself lies.
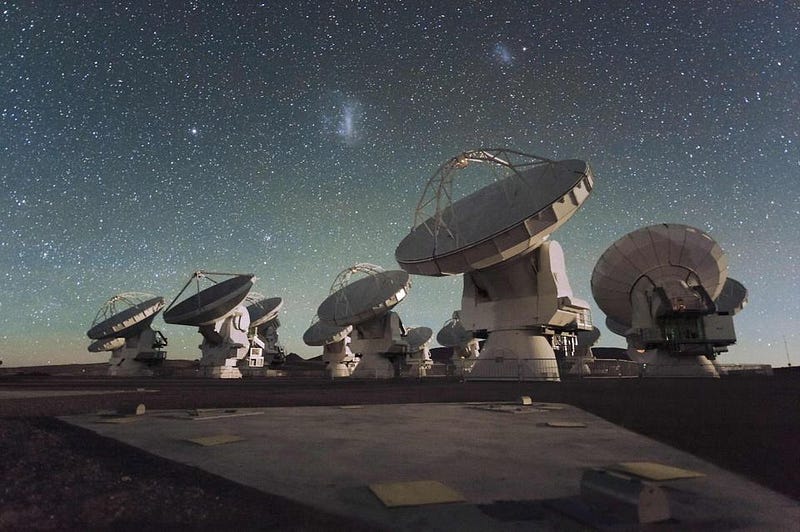
The technological revolution that should enable these images to be constructed is ALMA*: the Atacama Large Millimeter/Submillimeter Array. An incredible network of 66 radio telescopes, all of which themselves are huge (see above), measure this long-wavelength light to reveal astronomical details as never before. Already ALMA has shown us images of the dusty disks around newly forming stars, with evidence for the infant planets (as ring-like gaps in the disk) forming inside. ALMA can image ultra-distant galaxies in a superior fashion to what even Hubble can reveal, and has found molecular gas signatures and internal rotations.
But perhaps its biggest scientific gift will be all the information it gathers from the light surrounding these supermassive black holes. Writing down enough (and the right kinds of) data, fast enough, and then bringing them together with enough computational power to analyze them, is only now, for the first time, possible.
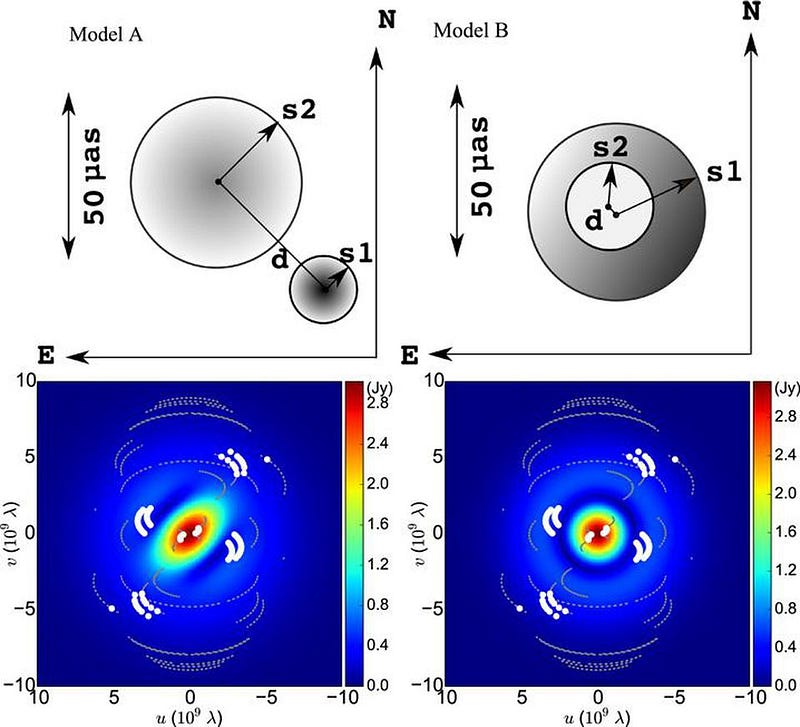
So what will 2019 bring, when all 27 Petabytes of data (from all the different observatories viewing these black holes), having been brought together, are fully analyzed? Will the event horizon appear as General Relativity predicts? There are some incredible things to test:
- whether the black hole has the right size as predicted by General Relativity,
- whether the event horizon is circular (as predicted), or oblate or prolate instead,
- whether the radio emissions extend farther than we thought,
- or whether there are any other deviations from the expected behavior.
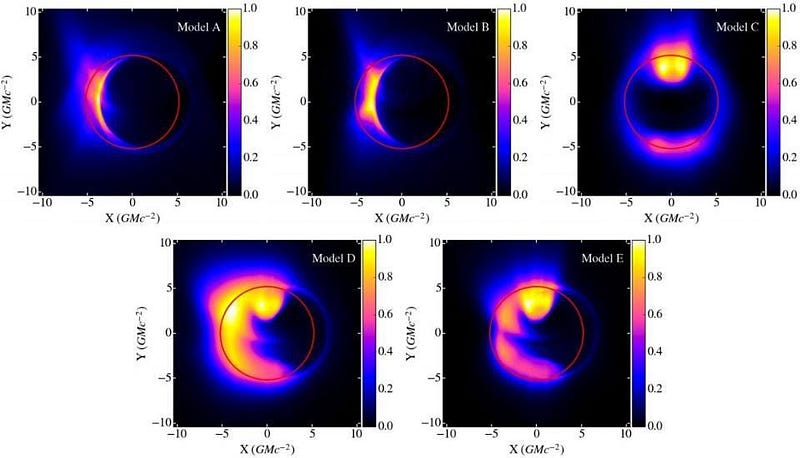
Although the Event Horizon Telescope team has detected structure around the black hole at our galaxy’s center, we still don’t have a direct image. This requires understanding our atmosphere and the changes occurring within it, combining the data, and writing novel algorithms to co-process them. It’s a work in progress, but the first half of 2019 is when the final, first images ought to arrive. Some of us were hoping for the images this year or even last year, but it’s most important that we take the time and the care to get it right.
When these images finally do arrive, there will no longer be any doubt as to whether black holes exist, and whether they exist with the properties that Einstein’s greatest theory predicts. 2019 will be the year of the event horizon, and for the first time in all of history, we’ll finally know, conclusively, what they look like.
* — Full disclosure: the author will be leading a limited-space tour to Chile which includes a visit to ALMA, the telescope array instrumental in gathering the data for this image, in November of 2019. (Spaces still available.) He received no outside compensation for this piece.
Ethan Siegel is the author of Beyond the Galaxy and Treknology. You can pre-order his third book, currently in development: the Encyclopaedia Cosmologica.