Ask Ethan #60: Why is the Universe’s energy disappearing?
The cosmic background radiation of the Universe once fried everything, but is now barely above absolute zero. Where did that energy go?
“I think one of the coolest things you can do is disappear for a while, because it gives you the chance to re-emerge.” –Josh Homme
When you think about the Big Bang, it’s one of the most difficult abstractions to fully wrap your mind around. Sure, the Universe is expanding today, which means that things were closer together in the past, and hence our Universe was denser. But it was also hotter, and therefore the particles in it were more energetic, as compared to today, where they’re cooler. This week’s Ask Ethan comes to us courtesy of Barry Pardoe, who wants to know the following:
I understand that the CMB is slowly cooling down as the Universe expands, and that the red-shifted particles of the CMB are moving to longer wavelengths and lower energies. What I was wondering is where the energy of these particles is actually going?
Let’s take this apart, and see why this question is so tremendously profound.
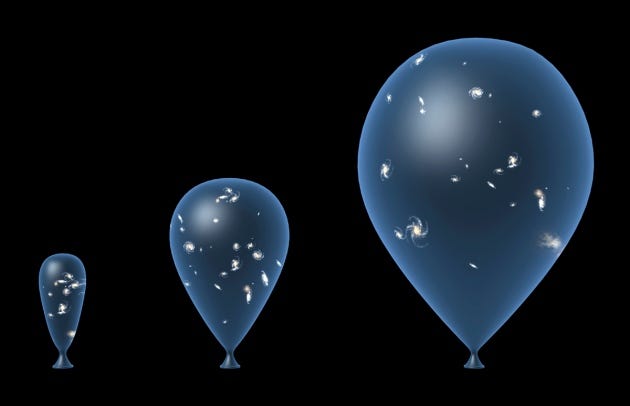
It’s pretty easy to conceive of how density drops as the Universe expands, and how — if it somehow contracted again — its density would begin to rise once more. This is because density is simply the amount of stuff you have in a given region of space: mass density is mass-per-volume, number density is number-per-volume, and energy density is energy-per-volume.
For matter — stuff like atoms, gas, planets, stars and galaxies (and even dark matter) — it’s pretty intuitive to put that in the context of spacetime that evolves over time. If your spacetime expands, your density drops, and if your spacetime contracts, your density rises.
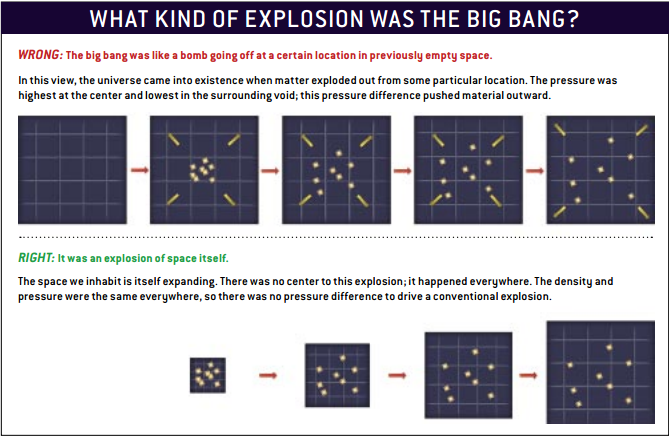
But this is all because the volume is changing. The mass stays the same, the number-of-particles stays the same, and the total energy stays the same. In an expanding Universe filled with matter, the density changes because the Universe expands in a very straightforward fashion.
But in a Universe that’s also filled with radiation — photons, or particles of light, in our Universe’s case — a change in the volume of the Universe does something else that we might not expect.
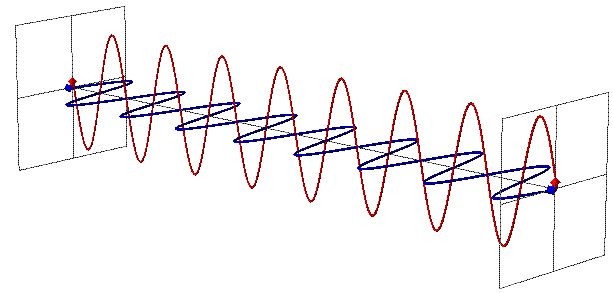
You see, you’re used to thinking of particles as, well, particles, which is to say, points in space. You’re used to thinking of them as entities without a malleable size, so that as the Universe does its thing — expanding or contracting, as it’s wont to do — the particles stay the same. But photons aren’t like that at all.
A photon, remember, is not just a particle (although it can collide and interact like one), but it also behaves as an electromagnetic wave. And one of the most important, defining features of any wave is its wavelength, which in the case of a photon, determines its energy.
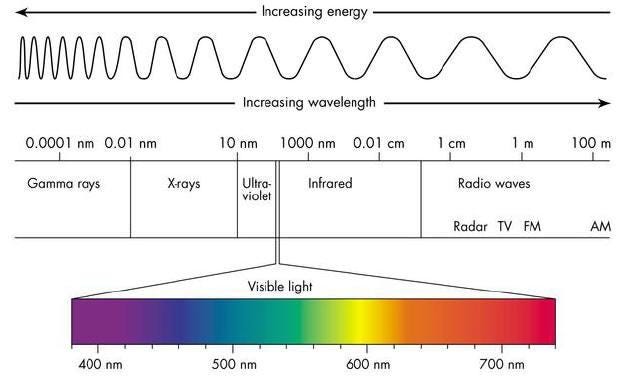
The longer your wavelength, the less energy you have, and the shorter your wavelength, the more energy you have. Right now, with the Universe at its present size, a typical photon left over from the earliest stages of the Universe has an energy that corresponds to a temperature of 2.725 degrees (Kelvin) above absolute zero. We can convert that into a wavelength using a combination of fundamental constants — Boltzmann’s contast, Planck’s constant and the speed of light — and find that this is a wavelength of about 5.28 millimeters, or about the length of the whites of your fingernails when it’s time to cut them.
You can fit about 189 waves of this light across a meter of space. But in the past, because the Universe is expanding, every meter in intergalactic space was smaller!
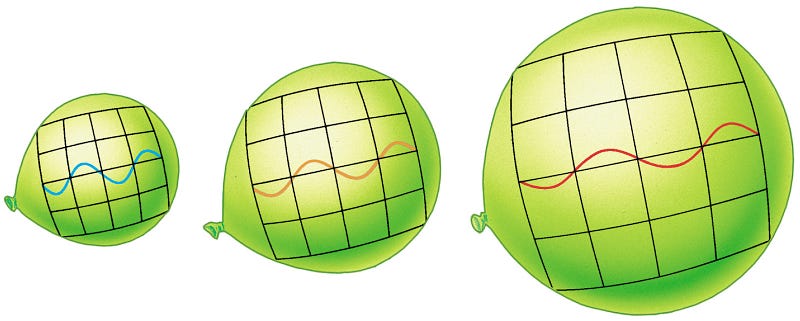
This doesn’t mean that fewer waves would fit in that same space. Remember, instead, that the number density per unit volume stays the same in an expanding Universe. So what happens, then? You’d be able to fit 189 waves of this light across whatever distance back then expanded, over time, to correspond to one meter today!
- When the Universe was half the size it is today? 189 waves per half-meter, or a wavelength of 2.64 millimeters.
- When the Universe was 10% the size it is today? 189 waves per decimeter, or a wavelength of 528 microns.
- When the Universe was 0.01% the size it is today? 189 waves per tenth-of-a-millimeter, or a wavelength of 528 nanometers: visible light! (And a yellowish-green color at that.)
The farther back in the past you go — to when the Universe was smaller — the more energetic your radiation was. The radiation we see today from the Big Bang comes from the time where neutral atoms formed: the cosmic surface of last scattering.
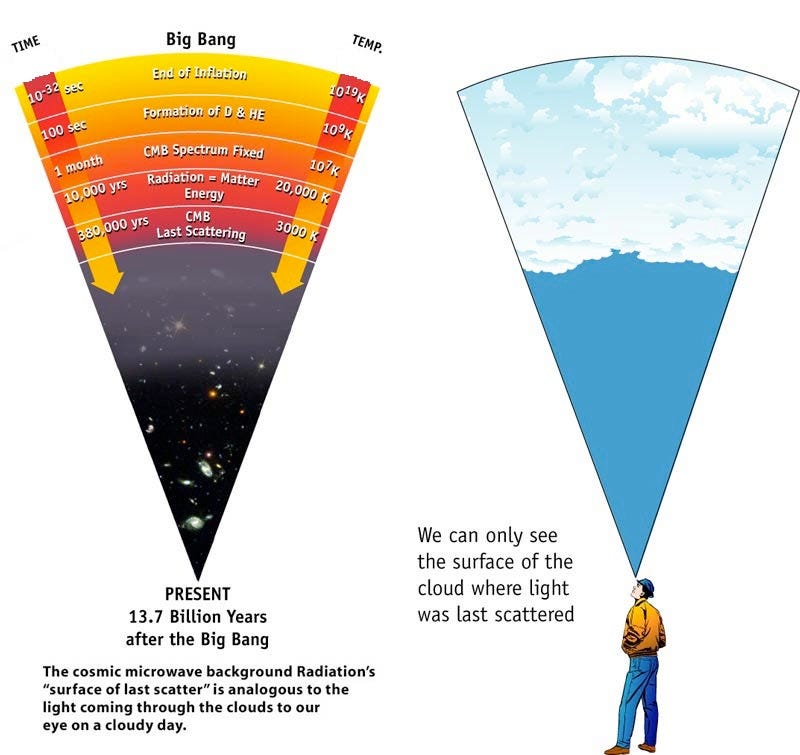
This explains why there was a time in the past where there weren’t neutral atoms (where the cosmic microwave background is emitted from), where there weren’t atomic nuclei (because they were blasted apart; immediately after that was the time when the light elements of the Universe were synthesized), where protons and neutrons were blasted apart into a quark-gluon plasma, and even earlier where things were so hot that exotic matter-antimatter pairs were spontaneously being created from the incredibly high-energy gamma rays populating the Universe.
This also explains why that leftover radiation, today, appears shifted all the way down to microwave wavelengths. These are simple, basic predictions that emerge from this kind of physics and the concept of the Big Bang.
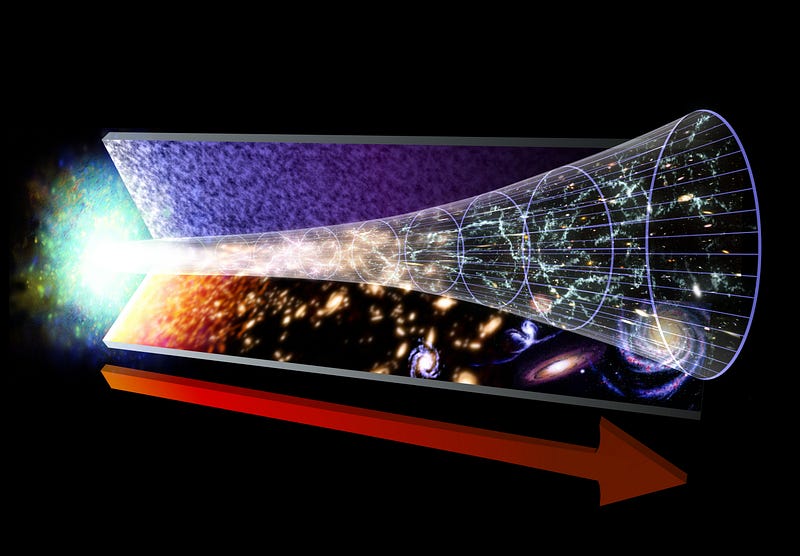
But this might bother you, just as it bothers Barry. Isn’t energy conserved? And if there’s less energy now, wouldn’t that mean energy has just been lost, and therefore not conserved? (In the strictest sense in General Relativity, there is no definition of energy, but we don’t need to weasel our way out of this one with excuses like that.)
This radiation’s energy didn’t just “get lost” as you might assume; what I’d like you to do is think of an analogy here. Imagine you’ve got a balloon that you’ve blown up and tied off, and it’s now nice and inflated, and in equilibrium with its surroundings. I can measure the total amount of energy that’s in the air inside the entire system of the balloon, and I’ll be satisfied.
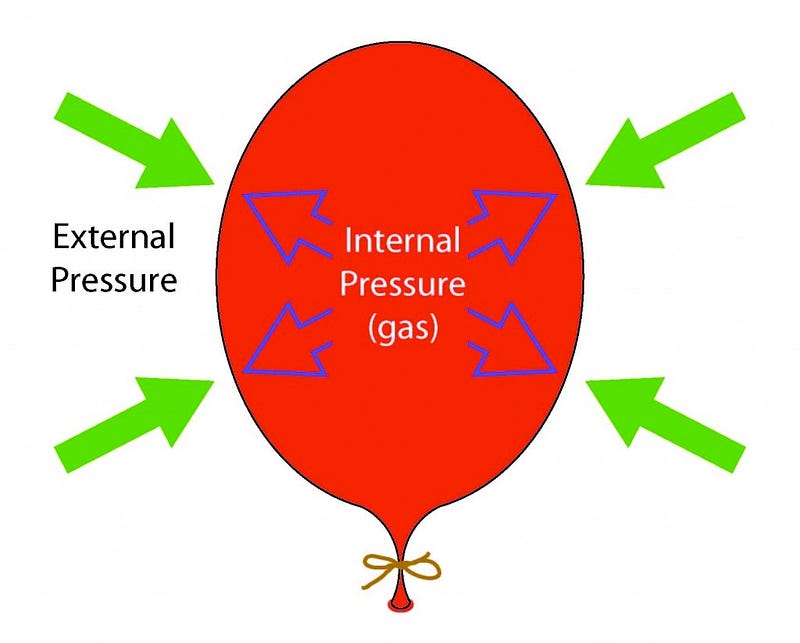
Then I do something super cruel to the molecules inside the balloon, and dunk the entire thing into liquid nitrogen at a miserable 77 K. The liquid nitrogen sucks the heat right out of the molecules in the balloon (and the balloon itself), and the volume inside the balloon drops.
But that’s not the entire story. There’s something else at play here as well: the molecules were exerting the outward force that kept the walls of the balloon from collapsing inwards, and when they lost their energy, the outward force they exerted became insufficient, and the balloon walls moved inwards. If you pull your balloon out of the liquid nitrogen, now, and let the warm air outside warm up the air inside again, it gains energy, and re-inflates the balloon, pushing the balloon walls out while exerting an outward force.
That whole idea — of exerting a force in a certain direction while something moves either in that direction or the opposite direction — is what the physical concept of work is. You push outward while something move inwards, and you’re doing negative work, taking energy out of the system. You push outward while something moves outward, and you’re doing positive work, adding energy to the system. This is what blowing up a balloon is, perhaps the simplest example of this type of force / distance / work combination.
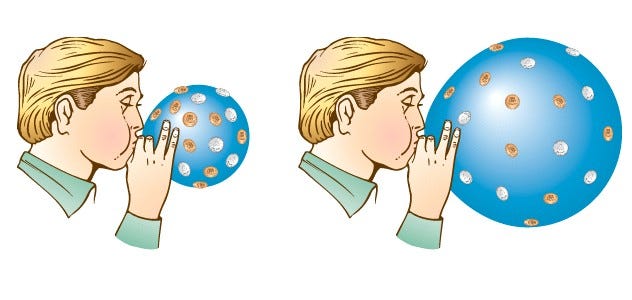
In the case of the expanding Universe, the photons act like the air inside the balloon: they push outward while the Universe expands outward, doing positive work on the Universe. The photons lose energy, but that energy gets transferred into the Universe itself, in a completely reversible way! (In other words, if the Universe ever contracted or recollapsed, the energy that the photons added into the Universe would go right back into the photons.)
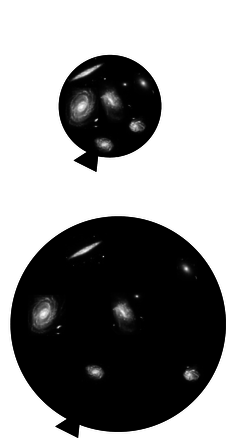
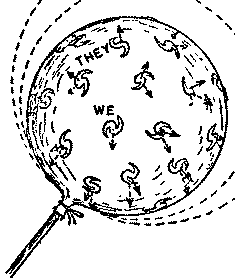
So where does the energy from photons go in the expanding Universe? The energy from the photons do work, transferring it into the Universe itself.
Thanks for an outstanding question, Barry, and I hope this helps explain it in a way that you (and many others) can understand! Send in your questions and suggestions here, and who knows: your entry just might be the subject of our next Ask Ethan!
Leave your comments at the Starts With A Bang forum on Scienceblogs!