Ask Ethan: How Big Was The Universe When It Was First Born?
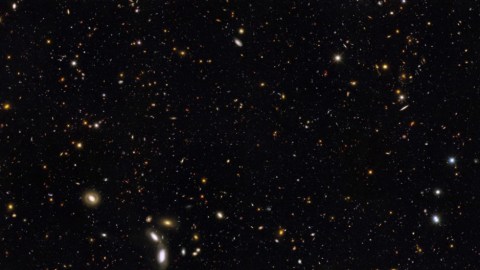
What we can see extends for 46.1 billion light years in all directions today. So how big was it at birth?
“They say it all started out with a big bang. But, what I wonder is, was it a big bang or did it just seem big because there wasn’t anything else drown it out at the time?” –Karl Pilkington
You might think of the Universe as infinite, and quite honestly, it might truly be infinite, but we don’t think we’ll ever know for sure. Thanks to the Big Bang — the fact that the Universe had a birthday, or that we can only go back a finite amount of time — and the fact that the speed of light is finite, we’re limited in how much of the Universe we can see. By time you get to today, the observable Universe, at 13.8 billion years old, extends for 46.1 billion light years in all directions from us. So how big was it all the way back then, some 13.8 billion years ago? Joe Muscarella wants to know:
I have read very different explanations about the size of the universe immediately after cosmic inflation ended. One source says it was about 0.77 centimeters, another says about the size of a soccer ball, while yet another says larger than the size of the observable universe. So which is it, or is it something else in between?
It’s been a very good year for questions about Einstein and the nature of space and time; since this is the 100th anniversary of General Relativity, that’s quite fitting. Let’s start by talking about the Universe we can see.
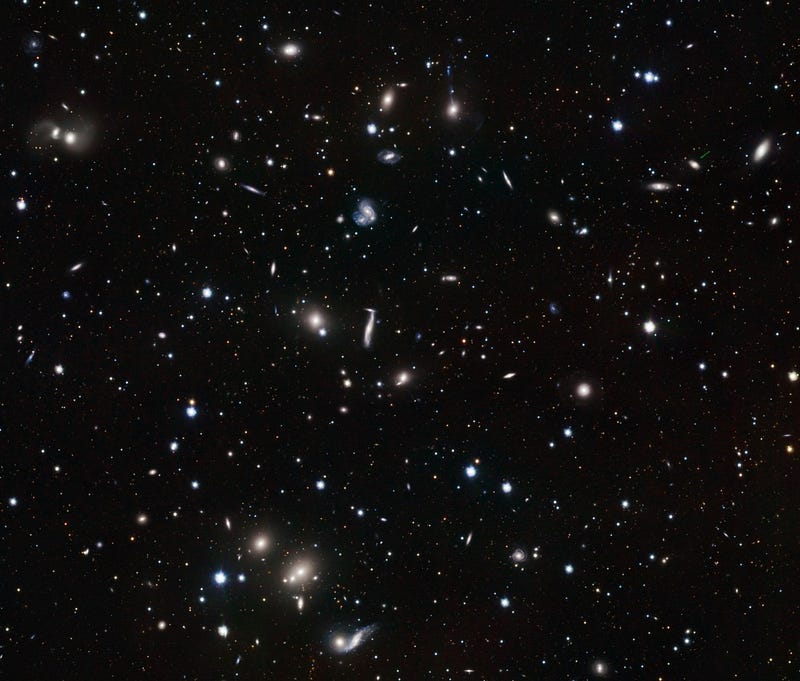
When we look out at the distant galaxies, as far as our telescopes can view, there are some things that are easy to measure, including:
- what its redshift is, or how much its light has shifted from an inertial frame-of-rest,
- how bright it appears to be, or how much light we can measure from the object at our great distance,
- and how big it appears to be, or how many angular degrees it takes up on the sky.
These are very important, because if we know what the speed of light is (one of the few things we know exactly), and how intrinsically either bright or big the object we’re looking at is (which we think we know; more in a second), then we can use this information all together to know how far away any object actually is.
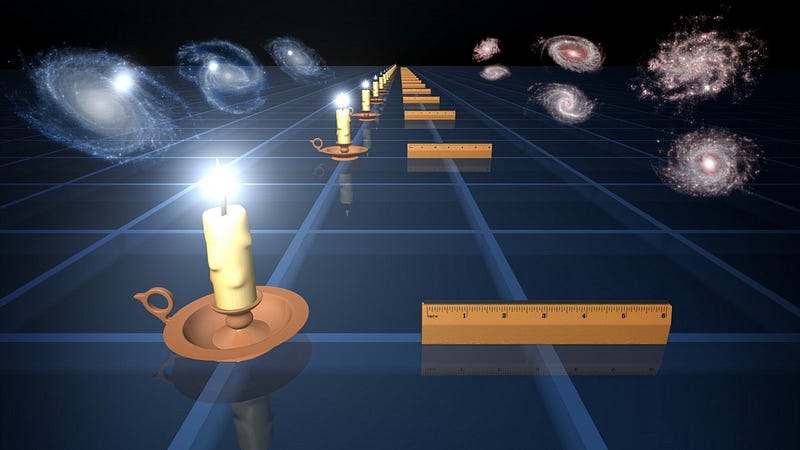
In reality, we can only make estimates of how bright or big an object truly is, because there are assumptions that go into this. If you see a supernova go off in a distant galaxy, you assume that you know how intrinsically bright that supernova was based on the nearby supernovae that you’ve seen, but you also assume that the environments in which that supernova went off was similar, the supernova itself was similar, and that there was nothing in between you and the supernova that changed the signal you’re receiving. Astronomers call these three classes effects evolution (if older/more distant objects are intrinsically different), environmental (if the locations of these objects differ significantly from where we think they are) and extinction (if something like dust blocks the light) effects, in addition to the effects we may not even know are at play.
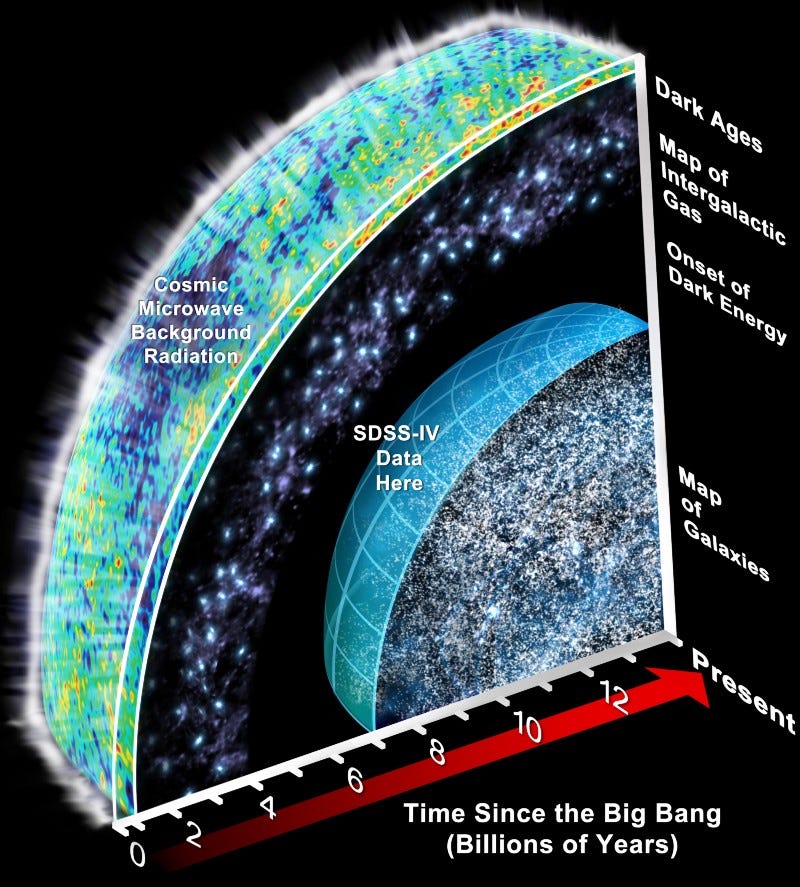
But if we’re right about the intrinsic brightness (or size) of an object we see, then based on a simple brightness/distance relation, we can determine how far away those objects are. Moreover, by measuring their redshifts, we can learn how much the Universe has expanded over the time the light has traveled to us. And because there’s a very well-specified relationship between matter-and-energy and space-and-time — the exact thing Einstein’s General Relativity gives us — we can use this information to figure out all the different combinations of all the different forms of matter-and-energy present in the Universe today.
But that’s not all!

If you know what your Universe is made out of, which is:
- 0.01% — Radiation (photons)
- 0.1% — Neutrinos (massive, but ~1 million times lighter than electrons)
- 4.9% — Normal matter, including planets, stars, galaxies, gas, dust, plasma, and black holes
- 27% — Dark matter, a type of matter that interacts gravitationally but is different from all the particles of the Standard Model
- 68% — Dark energy, which causes the expansion of the Universe to accelerate,
you can use this information to extrapolate backwards in time to any point in the Universe’s past, and find out both what the different mixes of energy density were back then, as well as how big it was at any point along the way.
So for you, Joe, I went and did these things. (And plotted them on logarithmic scales, where they’re more informative.)
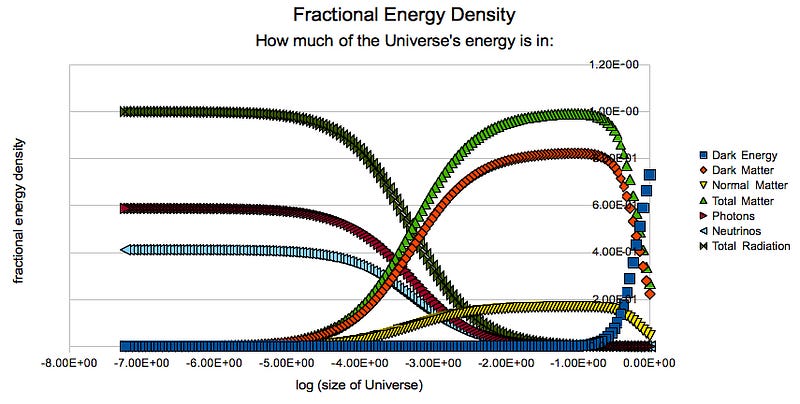
As you can see, dark energy may be important today, but this is a very recent development. For most of the first 9 billion years of the Universe’s history, matter — in the combined form of normal and dark matter — was the dominant component of the Universe. But for the first few thousand years, radiation (in the form of photons and neutrinos) was even more important than matter!
I bring these up because these different components, radiation, matter and dark energy, all affect the expansion of the Universe differently. Even though we know that the Universe is 46.1 billion light years in any direction today, we need to know the exact combination of what we have at each epoch in the past to calculate how big it was at any given time. Here’s what that looks like.
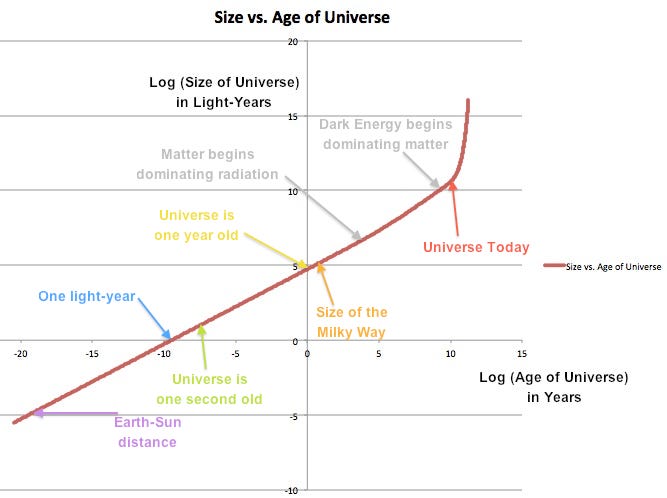
Here are some fun milestones, going back in time, that you may appreciate:
- The diameter of the Milky Way is 100,000 light years; the observable Universe had this as its radius when it was approximately 3 years old.
- When the Universe was one year old, it was much hotter and denser than it is now. The mean temperature of the Universe was more than 2 million Kelvin.
- When the Universe was one second old, it was too hot to form stable nuclei; protons and neutrons were in a sea of hot plasma. Also, the entire observable Universe would have a radius that, if we drew it around the Sun today, would enclose just the seven nearest star systems, with the farthest being Ross 154.
- The Universe was once just the radius of the Earth-to-the-Sun, which happened when the Universe was about a trillionth (10^–12) of a second old. The expansion rate of the Universe back then was 10^29 times what it is today.
If we want to, we can go back even farther, of course, to when inflation first came to an end, giving rise to the hot Big Bang. We like to extrapolate our Universe back to a singularity, but inflation takes the need for that completely away. Instead, it replaces it with a period of exponential expansion of indeterminate length to the past, and it comes to an end by giving rise to a hot, dense, expanding state we identify as the start of the Universe we know. We are connected to the last tiny fraction of a second of inflation, somewhere between 10^–30 and 10^–35 seconds worth of inflation. Whenever that time happens to be, where inflation ends and the Big Bang begins, that’s when we need to know the size of the Universe.

Again, this is the observable Universe; the true “size of the Universe” is surely much bigger than what we can see, but we don’t know by how much. Our best limits, from the Sloan Digital Sky Survey and the Planck satellite, tell us that if the Universe does curve back in on itself and close, the part we can see is so indistinguishable from “uncurved” that it much be at least 250 times the radius of the observable part.
In truth, it might even be infinite in extent, as whatever the Universe did in the early stages of inflation is unknowable to us, with everything but the last tiny fraction-of-a-second of inflation’s history being wiped clean from what we can observe by the nature of inflation itself. But if we’re talking about the observable Universe, and we know we’re only able to access somewhere between the last 10^–30 and 10^–35 seconds of inflation before the Big Bang happens, then we know the observable Universe is between 17 centimeters (for the 10^–35 second version) and 168 meters (for the 10^–30 second version) in size at the start of the hot, dense state we call the Big Bang.
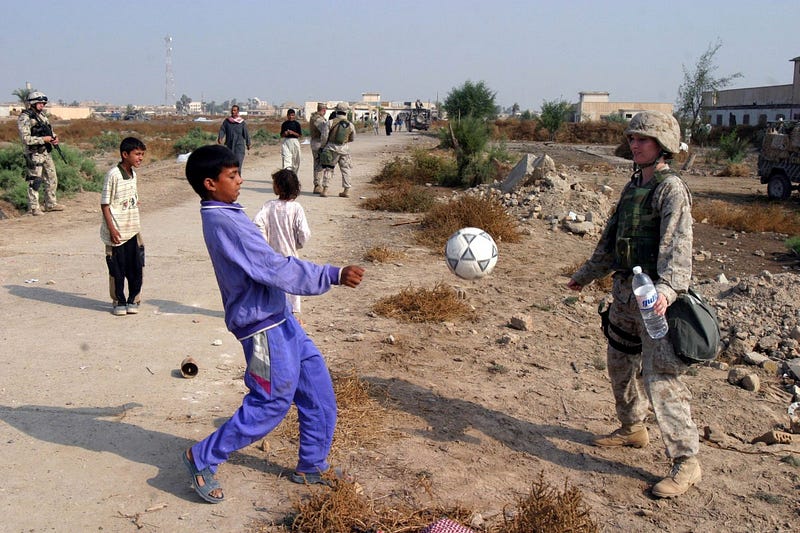
The 17 centimeters answer, by the way, is about the size of a soccer ball! So if you just wanted to know which of those estimates was closest to right, based on what we know, go with that one. The less-than-one-centimeter estimate is too small; we have constraints from the cosmic microwave background that inflation couldn’t have ended at energies that high, meaning that a size for the Universe at the start of the “bang” is ruled out. The larger-than-the-Universe-today version must be talking about the unobservable Universe, which is probably right, but which doesn’t offer any hopes of being measured in any foreseeable way.
So how big was the Universe when it was first born? If the best models of inflation are right, somewhere between the size of a human head and a skyscraper-filled city block. Just give it time — 13.8 billion years in our case — and you wind up with the entire Universe.
You can submit your questions and suggestions for the next Ask Ethan here.
Leave your comments on our forum, and check out our first book: Beyond The Galaxy, available now, as well as our reward-rich Patreon campaign!