How devouring “the Kraken” made the modern Milky Way
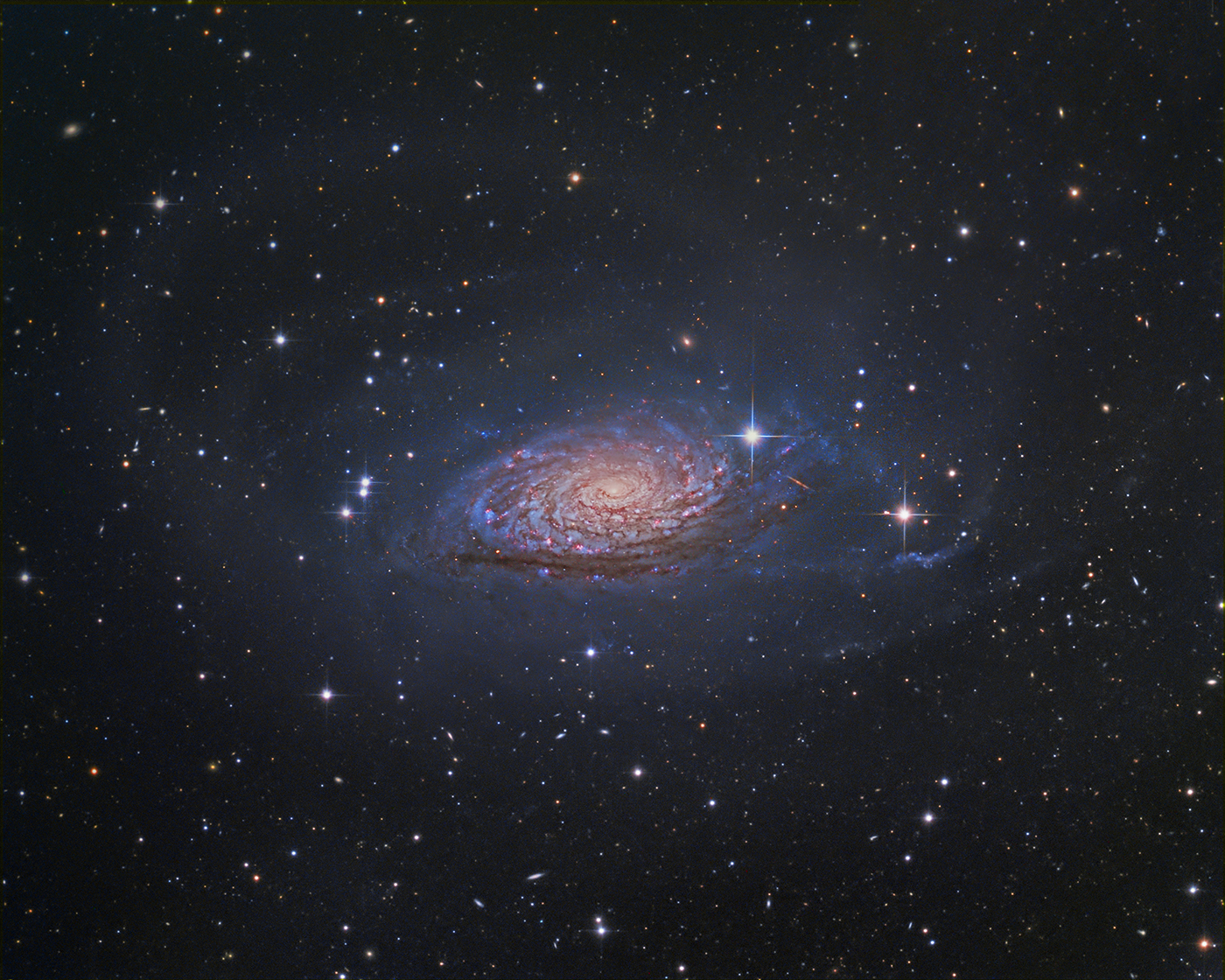
- Although the Milky Way has been around for more than 90% of the Universe’s history, we still don’t know how it grew to be its present size.
- Two processes are both expected to contribute: mergers with other, smaller galaxies and gravitational growth by absorbing intergalactic matter.
- By examining the Milky Way’s globular clusters, we can identify at least 5 galactic mergers in our past. “The Kraken,” 11 billion years ago, was the largest.
When it comes to any aspect of the Universe, there are two questions we always attempt to answer: “What is it like today?” and “how did it become the way it is?” From atoms to planets to stars to galaxies, we seek to both understand what things are like today and to gain an understanding of how they evolved from their precursor ingredients into their present state. It’s tremendously difficult. However, in astronomy, we cannot perform experiments at will: We only have the Universe as it exists today — a momentary snapshot of the cosmos — to observe. At this moment, all that remain are the survivors of a cosmically violent past.
But just as a good detective can use the scant evidence that exists to reconstruct what occurred at a crime scene, astronomers can use the various pieces of evidence that remain in the Universe, along with the known laws of physics that govern all objects, to reconstruct as much of our cosmic history as possible. Our Milky Way galaxy, most assuredly, wasn’t always the way it is today: large, massive, and filled with hundreds of billions of stars. Instead, we grew up via a combination of gravitational growth and mergers with other smaller galaxies. But which effect was more important and how “major” were the mergers that did occur? At long last, we’ve uncovered a significant piece of the answer: The act of devouring a galaxy known as the Kraken some 11 billion years ago led, at least in part, to our modern Milky Way. Here’s how we know.
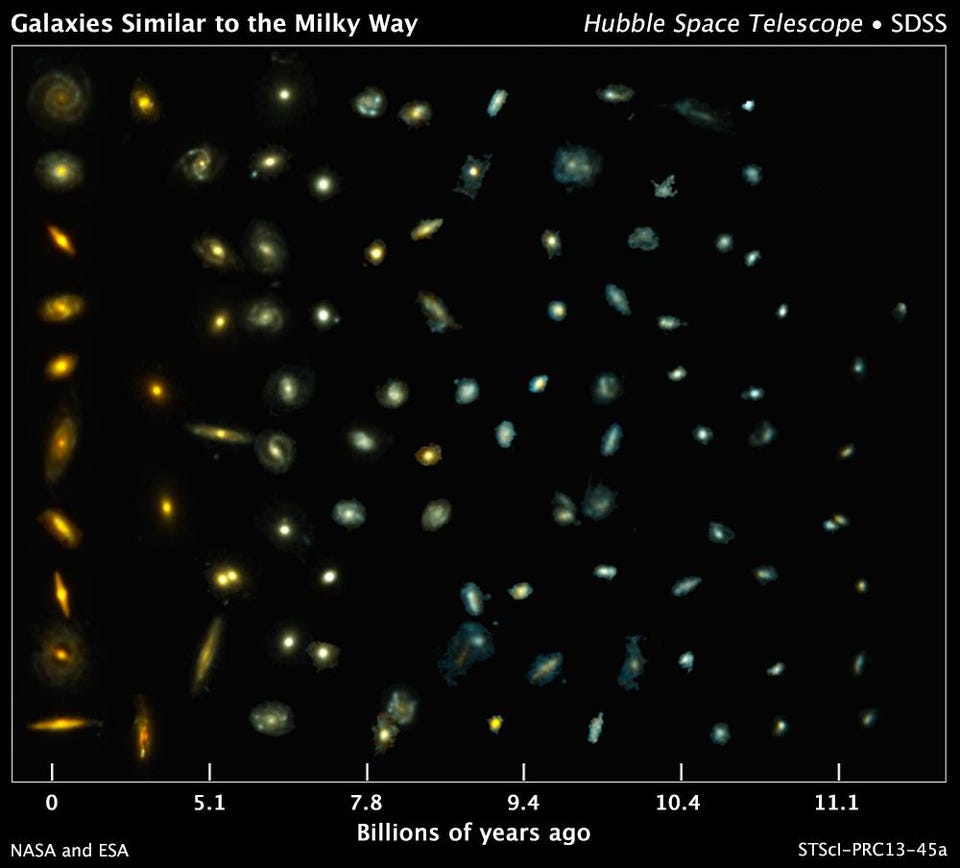
Credit: NASA, ESA, P. van Dokkum (Yale U.), S. Patel (Leiden U.), and the 3-D-HST Team
There are two very strong pieces of evidence that teach us that the Milky Way, as it is today, is not as it’s always been. The first is the stars that we see. While they come in a great variety of colors and masses, and have different proportions of heavy elements, the most important difference between the stars we see are their ages. The fact that many of the stars in the Milky Way are relatively young — along with the fact that star-formation is still ongoing in our gas-rich galaxy — demonstrates that stars formed at a variety of times, and that long ago, many of today’s stars didn’t exist.
The second piece of evidence is what we see when we look back across cosmic time at the plethora of galaxies we can observe and characterize. What we find is that many of today’ galaxies are like the Milky Way: similar in size, mass, and both the number and colors of stars that are present. As we look farther away in space, and hence farther back in time, we see that the galaxies are different:
- smaller in size
- lower in mass
- fewer stars
- mostly bluer in color and younger in age.
There can be no question that the Milky Way, and all modern galaxies in the Universe, have grown up in some way. The big question is: how?
One major idea is that galaxies form via a scenario known as monolithic collapse. In this scheme, we start with an initially overdense region in the Universe and allow it to gravitationally grow over time. As the Universe evolves, the matter from the surrounding regions get preferentially attracted to the densest, most massive “clump” around. More and more mass gets attracted to the same region of space, and when a critical threshold is crossed, this large collection of neutral matter will begin to gravitationally collapse, triggering the formation of stars.
This initial star cluster, if it’s large and massive enough, will continue to accrue additional mass from the surrounding regions of space, growing into a proto-galaxy and becoming even more massive. Since gravitation is a runaway force — attracting more matter into your clump makes you likelier to attract even more matter into you — the young Milky Way clearly did an excellent job of that compared to its peers; within our Local Group, only Andromeda is comparable in mass to the Milky Way. The idea that the Milky Way formed from a large clump, and then accumulated the rest of its matter via gravitational infall, is an important perspective to consider in how our home galaxy formed.

Credit NASA; Z. Levay and R. van der Marel, STScI; T. Hallas; A. Mellinger
The other major idea is that galaxies might form their seeds via the gravitational collapse of an initial overdensity, but then grow largely through major and minor mergers: where it isn’t gas and individual atoms that gravitationally fall in, but entire proto-galaxies and even full-fledged galaxies that merge together, giving rise to the modern Milky Way. We have lots of evidence that this happens all across the Universe, as a tremendous number of mergers, between both equal-mass galaxies and from galaxies with large differences in mass, have been observed with our present “snapshot” of the cosmos.
There’s another compelling reason to consider this scenario seriously: we know that mergers and “galactic cannibalism” are occurring even today, and they’re destined to continue far into the future. Our Milky Way has recently devoured the Sagittarius dwarf galaxy; is potentially in the process of devouring the Large and Small Magellanic Clouds (which are already gravitationally disrupted); and is on a collision course with the other major galaxy in our Local Group: Andromeda.
There’s little doubt that both a series of mergers and the gradual attraction of intergalactic matter are dually responsible for the formation of most modern-day galaxies. The specific question of how the Milky Way came to be, however, remains quite an open question.
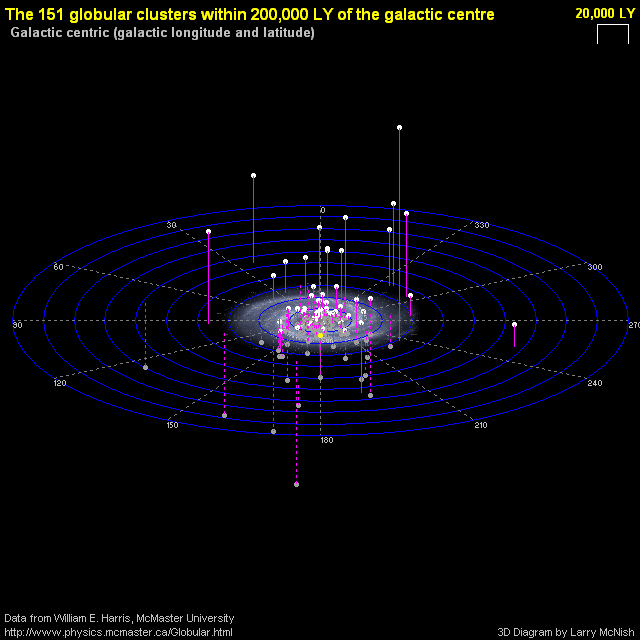
That said, there’s a remarkable set of objects that were formed long ago. These objects can reveal a plethora of information about our own galaxy’s history: globular clusters. The Milky Way contains somewhere in the neighborhood of about ~150 globular clusters, which is relatively typical — although perhaps somewhat towards the low end — of what’s expected for a galaxy of our size and mass. The largest cosmic behemoths, like the galaxy at the core of the Virgo cluster, M87, can have up to tens of thousands of globular clusters, while small, satellite dwarf galaxies might have only a handful.
The reasons globular clusters are so fascinating are as follows.
- These are isolated systems, where practically all of the stars inside formed in one gigantic burst: all at once.
- They’re pretty much all very old, with the “young ones” somewhere around 7-to-10 billion years old and the “old ones” somewhere between 12-to-13.5 billion years old.
- They come in a tight range of masses, typically containing anywhere from a few hundred thousand to a few tens of millions of stars.
- They’re also all very small in physical extent: with all of their stars contained within a few dozen light-years of the cluster’s center.
- They come with a broad range of stellar concentrations, with some of them being diffuse and almost “coreless,” while others are extremely dense in the center and sparse towards the outskirts.
- Perhaps most importantly, they’re mostly metal-poor entities, where the stars inside all have the same rough percentage of elements other than hydrogen and helium as one another, but can vary from globular cluster to globular cluster, even within the same galaxy.
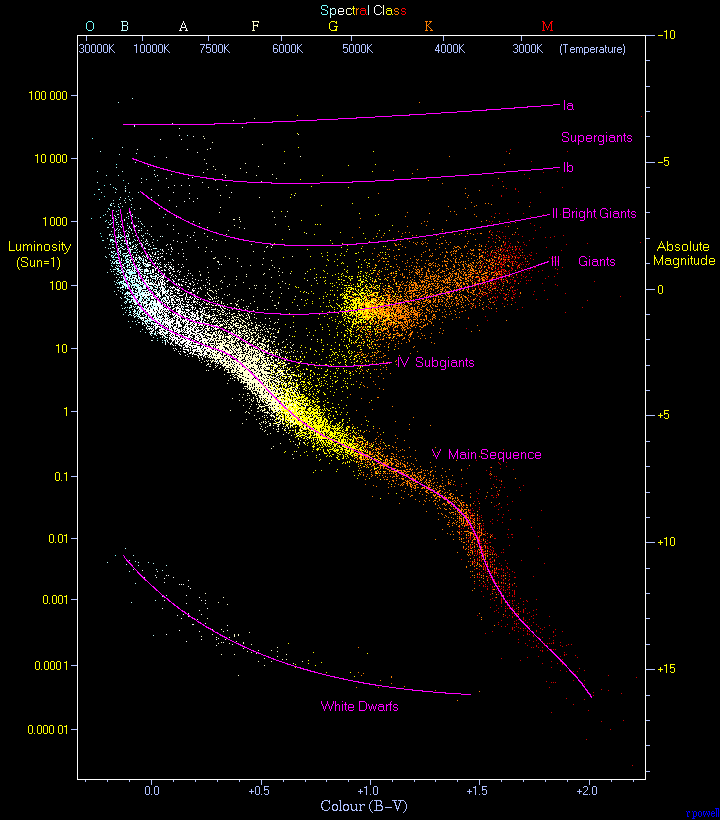
Credit: Richard Powell/Atlas of the Universe
The way we determine the ages of globular clusters is fascinating and tremendously instructive. Every star has two properties to it that are quite easy to measure: color and brightness. If we take all the stars within a cluster, whether a globular cluster or an open star cluster, we can plot them together on a color-magnitude diagram, known as a Hertzsprung-Russell (HR) diagram in astronomer-jargon. Typically, the brightness/magnitude is on the y-axis, with brighter stars being higher up, and the color is on the x-axis, with bluer stars on the left and redder stars on the right.
When stars are born, they follow a “snake-like” curve from the upper-left, where you get hot, bright, blue stars, down to the lower-right, where you get cool, faint, red stars. Now, here’s the kicker: the reason stars have different properties along this curve is because they have different masses. Mass, it turns out, is the primary determining factor in a star’s color and brightness for as long as it’s fusing hydrogen into helium in its core.
Mass is also the primary determinant of a star’s lifetime, meaning that as the stars in a cluster all age, it’s the most massive ones that run out of fuel in their core first. As these clusters age, the most massive stars evolve off of this snake-like line first. Therefore, if we can identify the “turn-off” point of a cluster, we can determine its age to a rather high precision.
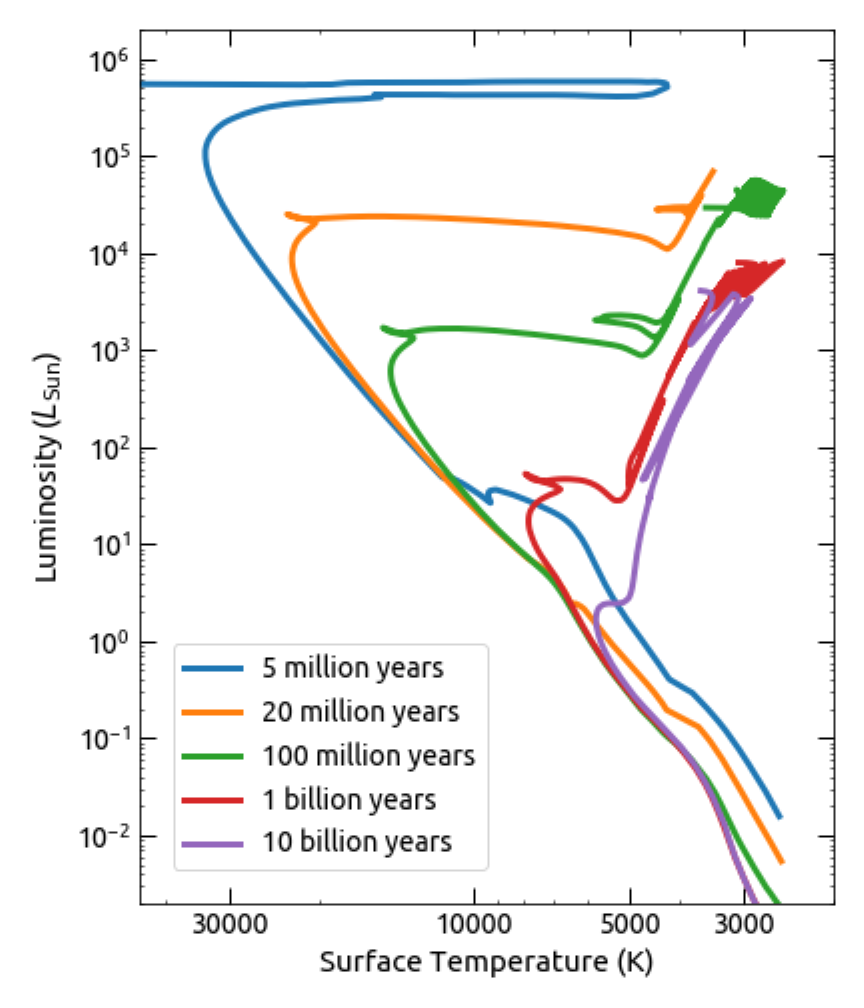
One of the interesting things we see when we look at the Milky Way’s globular clusters — clusters that are, by and large, close enough that we can easily measure the color and magnitudes of individual stars inside — we find something interesting. Yes, most of the clusters consist of very old stars: stars that are 12 billion years old or more. And most of the ones concentrated toward the galactic center have greater amounts of heavy elements than the ones concentrated farther away, toward the galactic outskirts.
Now, here’s where things get interesting. If your galaxy forms globular clusters because it drew matter in, and in a region of space, a large amount gathered in one place and led to the formation of a globular cluster, you’d expect that globular cluster would remain roughly in the same orbit where it formed. Yes, it would pass through the galactic plane, experience gravitational interactions, and lose mass (and stars) over time, but the ones that remain intact would keep their overall orbital properties with respect to the galaxy.
However, if your galaxy eats other galaxies, which possess their own globular clusters, their orbits should be — at least, on average — extremely different. If we could identify these types of differences between different populations of globular clusters within our own galaxy, we might even be able to determine whether they’re Milky Way originals, in some sense, or whether they’re the result of devouring a companion galaxy.
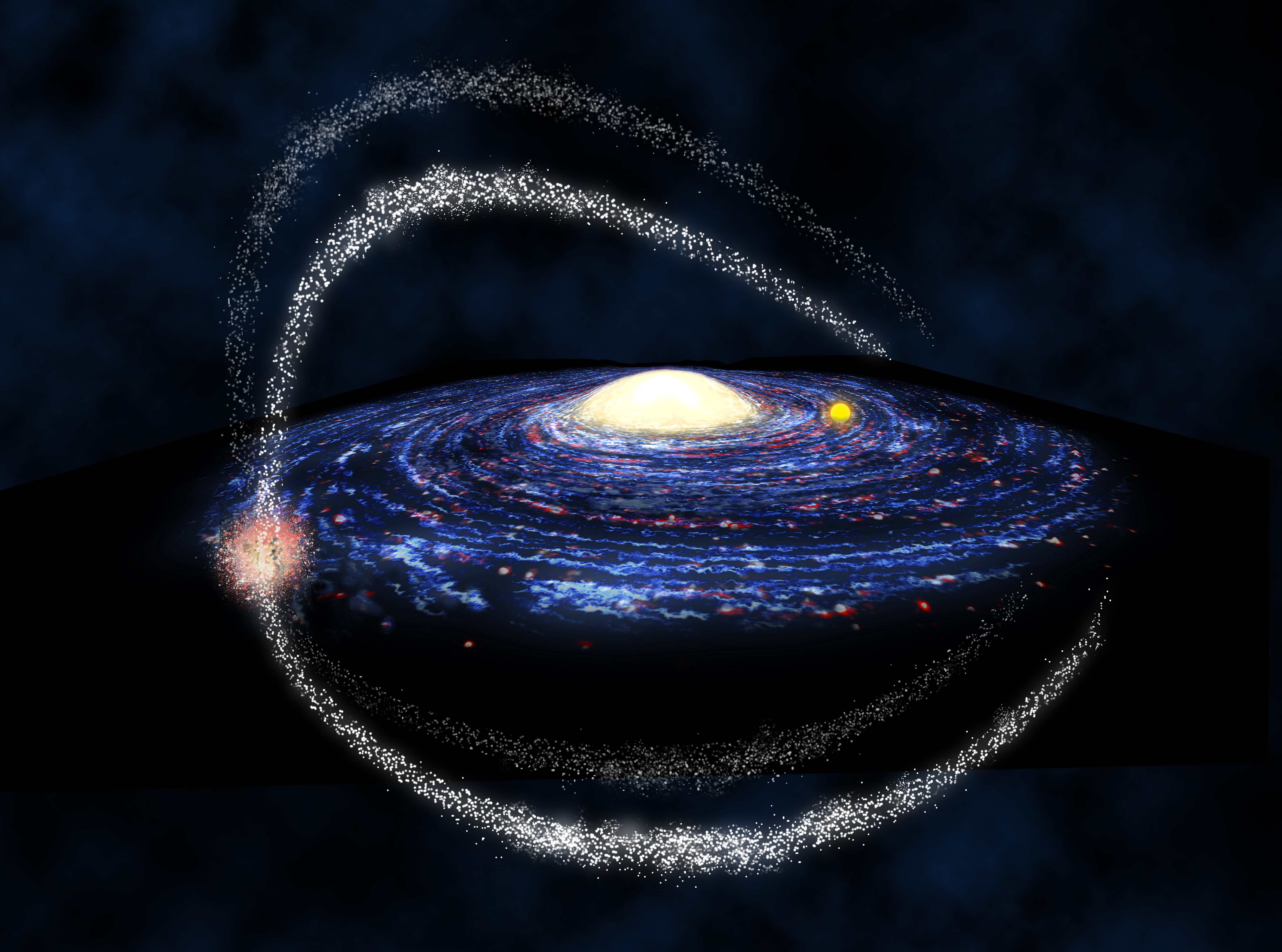
(Credit: Amanda Smith, Institute of Astronomy, University of Cambridge)
Of the “devourings” that have taken place, we can still see evidence of one of the most recent ones: the Milky Way’s cannibalism of the Sagittarius Dwarf Galaxy. The core of this galaxy still exists, although most of what remains has been stretched out into an enormous set of streams of stars. Importantly, though, it still possesses a series of globular clusters that must have formed with it, rather than with our own Milky Way.
By leveraging simulations and modern computational techniques, researchers in 2020 made a groundbreaking effort to use our globular cluster population to attempt to reconstruct the Milky Way’s merger history. Globular clusters form and evolve along with their galaxies, obtaining properties that imprint the host galaxy’s history upon them. When mergers between galaxies occur, the globular clusters get strewn about the new galaxy, with orbital properties that should differ substantially from the globular clusters than formed within the main galaxy itself.
In particular, what they found was fascinating: there were a series of (at least) five minor mergers that happened throughout the Milky Way’s history, and these mergers brought in approximately one-third of the globular clusters presently known within the Milky Way.
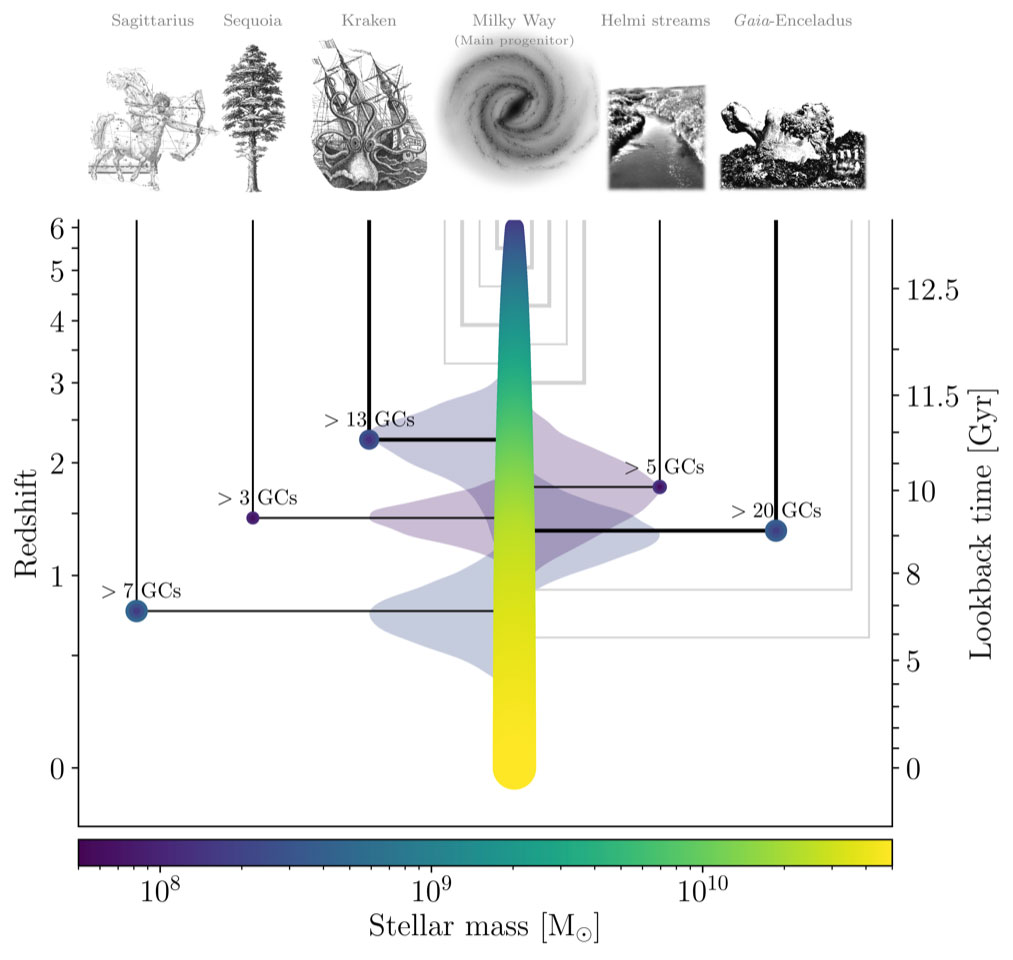
Credit: J. M. Diederik Kruijssen et al., MNRAS, 2020
Going back in time, the following events occurred:
- The Sagittarius dwarf galaxy joined ours about 7 billion years ago, bringing with it at least 7 globular clusters.
- About 9 billion years ago, the Gaia-Enceladus galaxy joined us, bringing at least a whopping 20 globular clusters along with it. Of all the galaxies that merged with the Milky Way in the past, this one was the most massive.
- Just slightly earlier, about 9.3 billion years ago, the Sequoia galaxy joined us, adding at least 3 globulars into the Milky Way.
- A little over 10 billion years ago, a galaxy known only by its faint remnant stellar streams, the Helmi streams, entered the Milky Way, bringing at least 5 globulars with it.
- But the oldest merger that we can identify was a galaxy that joined us approximately 11 billion years ago: the Kraken. It brought with it at least 13 globular clusters which are still present in our galaxy today.
Although the Gaia-Enceladus galaxy was the most massive of the five, the fact that it entered 9 billion years ago means that the Milky Way itself had grown substantially — by about a factor of 4 or 5 — since the merger of the Kraken. Compared to the size of our growing Milky Way, the Kraken was likely the largest merger our galaxy experienced, as the Kraken likely contained 3-4% of the Milky Way’s stars and 9-20% of the Milky Way’s mass at the time. Of all the galaxies we’ve identifiably merged with, the early Kraken galaxy likely played the largest role in shaping the evolutionary history of our own cosmic home.
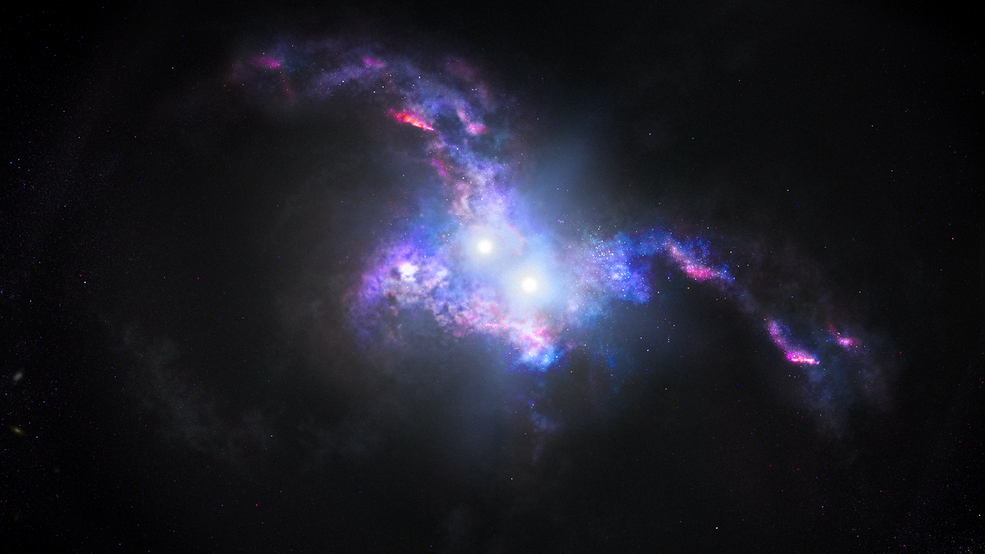
All told, studying the globular clusters of the Milky Way has, for the first time, revealed the merger history of our own galaxy. The stellar streams and globular clusters that remain from these events can be linked together, revealing which globulars came from which merger. In addition, all five identifiable mergers, cumulatively, likely brought a total of around 1 billion solar masses worth of stars into the galaxy.
This is remarkably informative, teaching us that while the merger of ancient galaxies with our Milky Way likely brought in one-third of our globular clusters, they added only 1% of our stars. The largest possible merger, at most, was still under ~20% of the mass of the Milky Way at the time. And, most importantly, it provides the first answer to one of the ultimate cosmic questions: How did our Milky Way grow up? Although mergers certainly occurred and played a role in shaping and growing our galaxy, most of the growth that’s happened was simply a result of the gradual, gravitational accumulation of matter. While major mergers may have been responsible for the growth of the most massive galaxies of all, the Milky Way holds a remarkable lesson for our cosmic perspective. When it comes to our home galaxy, slow and steady really did win the race.