4 unexpected lessons from the Milky Way’s weirdest star
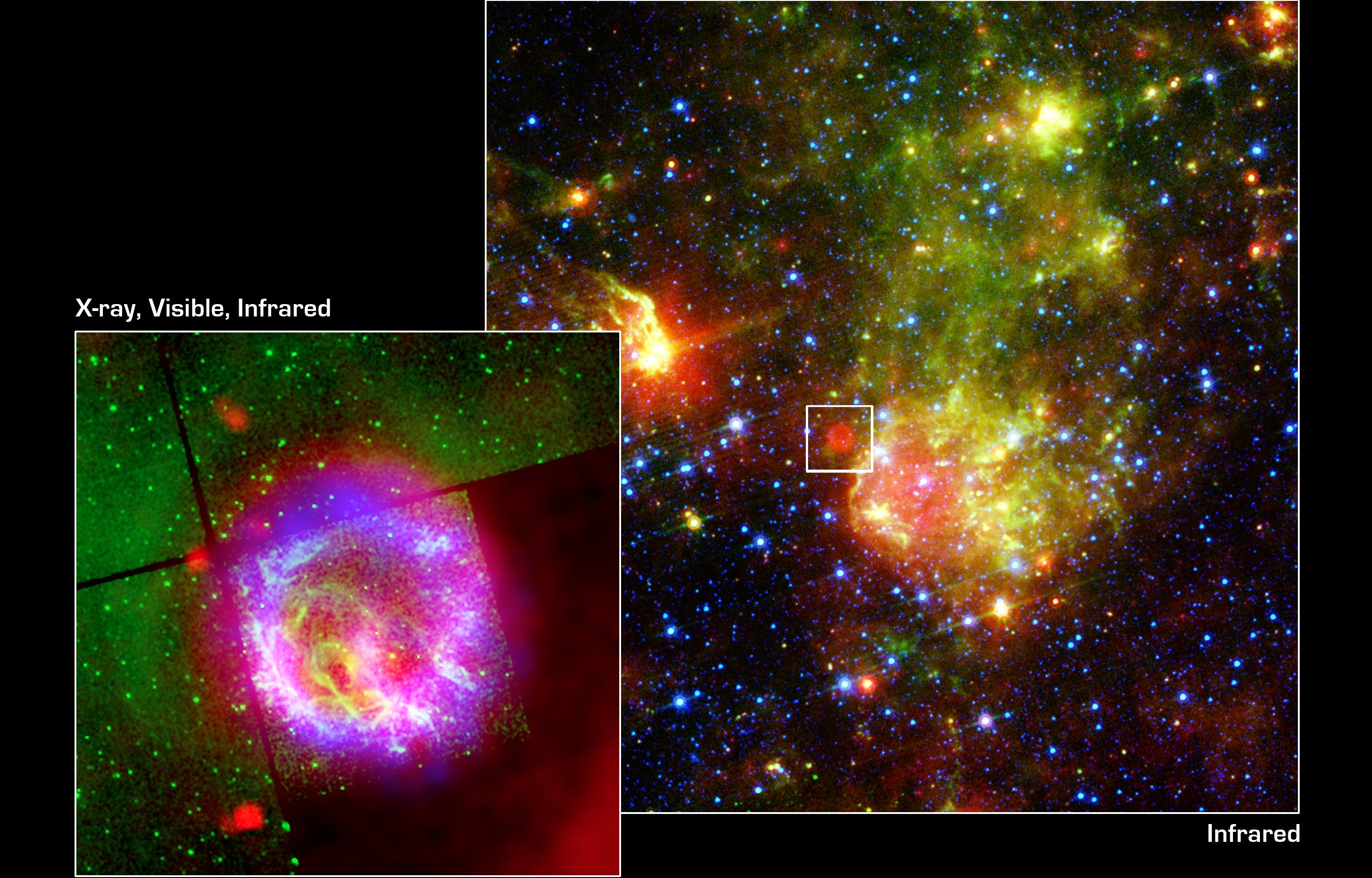
- With modern, large-scale astronomical survey facilities, we’re able to image and analyze more individual objects at once than ever before, with some appearing quite rare and unique.
- One serendipitous discovery, of star J0931+0038, shows properties we’ve never seen before: a population of various elements that doesn’t line up with any other star ever found.
- Asking the question of how this star came to be leads to some fascinating insights, including some that challenge our best models of how massive, young stars actually live and die.
Over the past 13.8 billion years, the Universe has evolved from a hot, dense, largely uniform early state to a clumpy, clustered, star-and-galaxy-rich state, where the typical interstellar and intergalactic distances are absolutely tremendous. The stars that exist today, importantly, are different from the stars that were created in the earliest stages of the Universe. Whereas the stars that are forming today are composed of all the recycled material that was once inside one-or-more stars and returned to the interstellar medium, the stars that were made early on were pristine: made of up primarily of hydrogen and helium alone: the material that existed shortly after the hot Big Bang.
Whenever we look at a star, we gain information about the cumulative history of the Universe up until the moment that particular star formed: of all the generations that lived-and-died prior to its formation. But early on, when the first few generations of stars were forming, it’s possible that the chemical enrichment of the “next generation” of stars may have primarily arisen from just one single, massive source. If this is the case, even for a few stars, we should find a population of very old stars with unusual compositions: with highly unusual element ratios compared to the more common varieties that formed from material that was well-mixed within the interstellar medium. With one remarkable discovery, a single star is helping us rewrite our cosmic history.
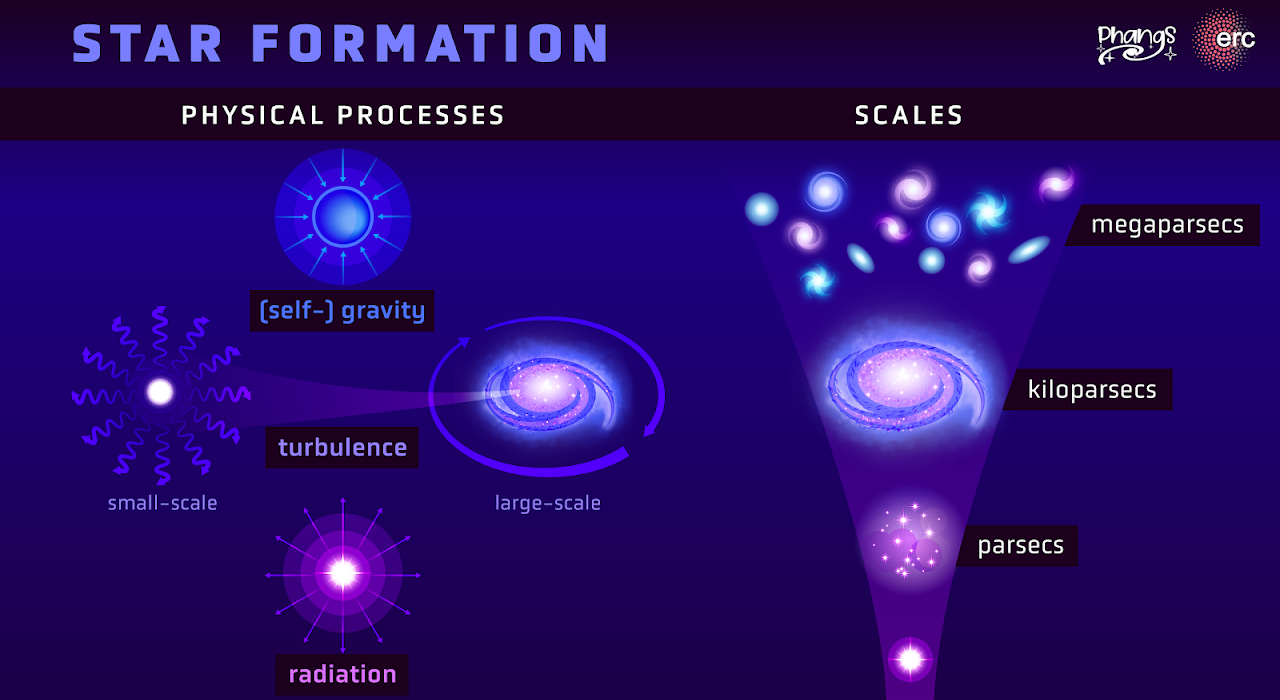
When we tell the history of star-formation in the Universe, it normally proceeds as follows. At the very beginning, there are no stars at all, as protons and neutrons, and then atomic nuclei, and then neutral atoms form. It takes tens or even hundreds of millions of years for these pristine clouds of gas to gravitationally grow massive enough so they can collapse, and when they do, they trigger the formation of the first generation of stars: stars initially made of hydrogen and helium alone.
But then the first generation of stars dies, including many of them in stellar cataclysms like supernovae, and all of the nuclear reactions that occurred within that star – the slow-burning fusion reactions that occurred in the star’s core during its lifetime, plus the rapid reactions that occur during a cataclysmic event – are cumulatively reflected in the material returned by that former star to the interstellar medium.
Over time, that enriched, ejected material then mixes with the remainder of the interstellar medium, which serves as a great cosmic recycling system. From that now-enriched interstellar material, new generations of stars will form, with the interstellar medium gradually getting more progressively enriched with time.
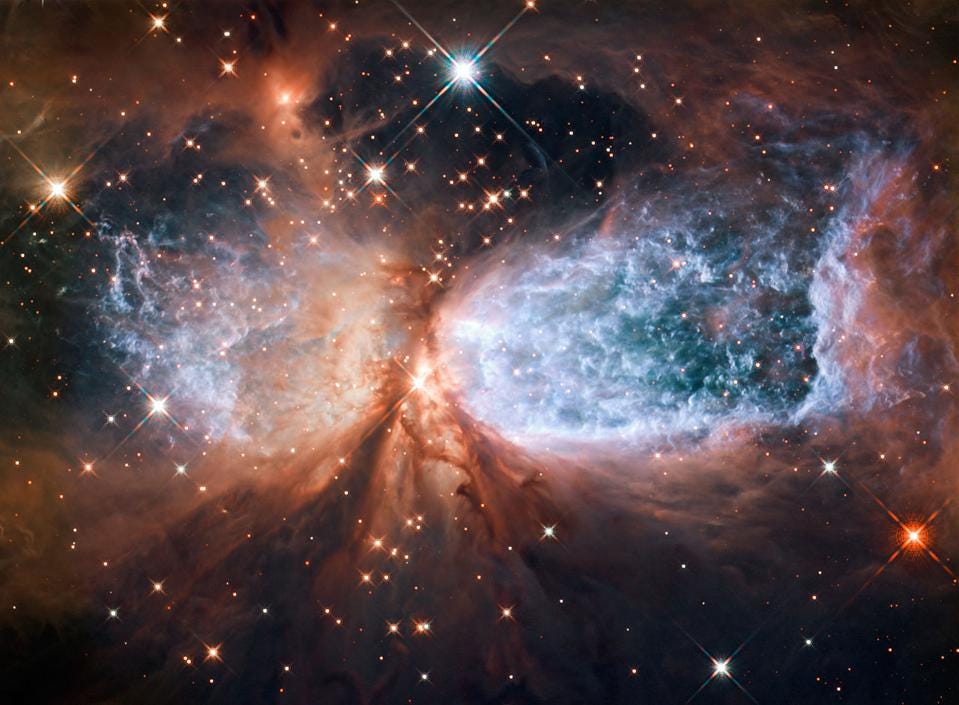
This is interesting from the perspective of learning how the Universe, and the stars within it, evolved and grew up: from early times until the present day. We exist today in the here-and-now, but the stars that we can see aren’t only the stars that are forming right now; they’re all the stars formed throughout the Universe’s history that are still burning and shining with respect to our observing perspective. If our goals include gaining an understanding of the earliest stars in the Universe, we have a couple of options:
- we can look to the greatest distances possible, and try to detect the earliest stellar populations back at the epochs when those stars were just in the process of forming,
- or we can look around us, nearby, at the oldest, most pristine relic stars that we can find, and try to learn what the Universe was like at the time of their formation by examining their properties today.
While the JWST era has given us an incredible wealth of data about the early Universe, it’s only in a few rare instances, such as the stars Quyllur and Earendel, that individual stars can even be resolved at all. Looking nearby, instead, has huge advantages from an observational perspective because of how much more you can measure as far as properties of that star is concerned. Instead of aggregate properties of a population of stars, you can make detailed measurements of individual stars themselves.
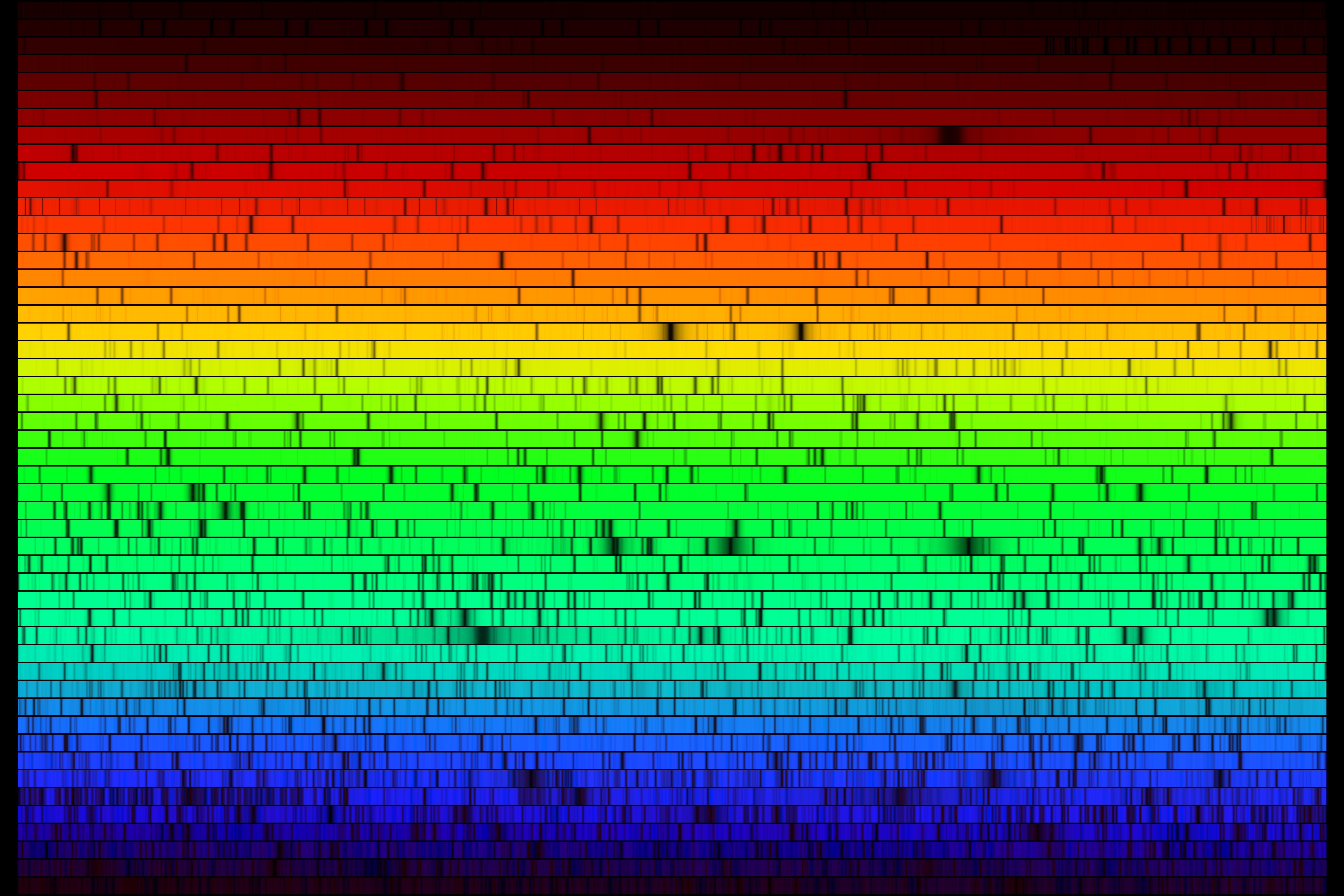
In general, there’s a single property you can measure to determine whether a star is “more pristine” versus “more enriched” in terms of the elements that make them up: metallicity. To an astronomer, any element that’s heavier than either hydrogen or helium is known as a metal, even supremely non-metallic elements such as oxygen, chlorine, and heavy noble gases. Because we’ve exquisitely measured the presence of practically all elements found in the Sun, we generally compare a star’s metallicity to that of the Sun in terms of its element ratios: iron (Fe) to hydrogen (H), oxygen (O) to hydrogen, or carbon (C) to hydrogen, for example.
However, where will the most enriched stars be found? And where will the most pristine stars be found? The highest-metallicity stars, the most enriched ones, are going to be found where the greatest number of previous generations of stars have formed, lived, and died: toward the centers and in the disks of the most massive, gas-rich galaxies. On the other hand, the lowest-metallicity stars, the most pristine ones, will be found where the fewest generations of stars have formed: in galactic halos, in ancient globular clusters, and in the far-flung outskirts of space. For the Milky Way, our home galaxy, we’ve even mapped out stellar metallicities as a function of their location in space.

At the present time, a suite of ground-based and space-based surveys have brought our astronomical knowledge to new, unprecedented heights. With tremendous sky coverage and impressive resolution, as well as wide-field cameras and optical setups, astronomers have surveyed not only large areas of the sky to very faint magnitudes, but have used these novel capabilities to measure many properties of enormous numbers of stars: hundreds of thousands in some surveys, millions in others, and even more than a billion stars in the case of the European Space Agency’s Gaia mission.
The reason you want to examine so many stars is because if you’re looking for outliers – for stars that don’t fit into the same broad categories that the vast majority of their brethren do – you have to survey a great number of them to even have a chance of finding the rare ones. The Sloan Digital Sky Survey (SDSS), for example, has been observing large areas of the sky for two decades now, with increasingly updated cameras and instruments. One of the stars it identified has properties that really stick out as unusual: J0931+0038, or J0931 for short, which was first identified by SDSS-V Milky Way Mapper data, which itself was designed to measure and analyze data from around 5 million separate Milky Way stars.
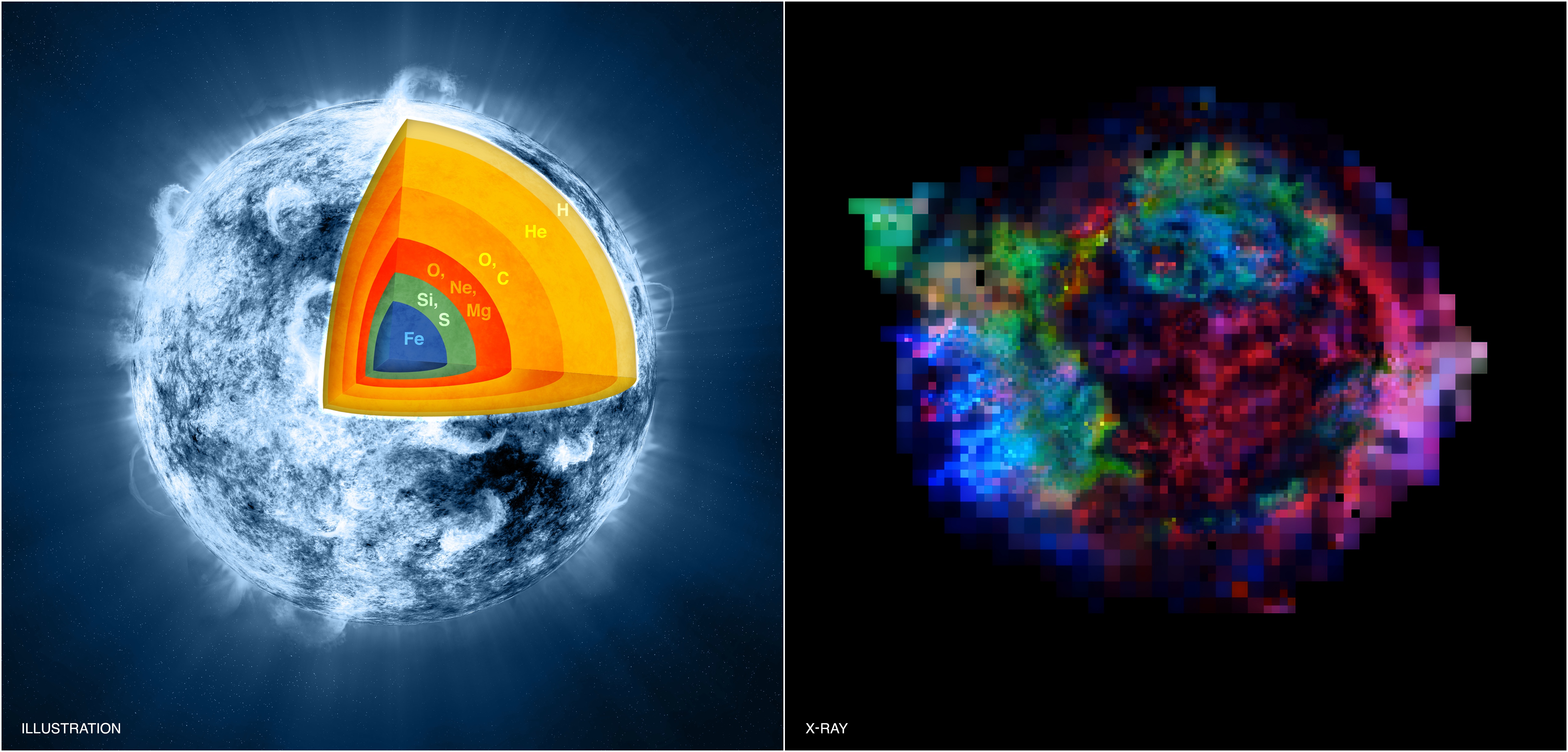
This star, J0931, was initially flagged by SDSS for having a low metallicity: or a very low abundance of heavy elements. The amount of iron in the star, relative to the amount of hydrogen in the star, indicates that there’s only about ~1.7% of the ratio of iron found in the Sun: a low abundance of heavy elements, but not quite pristine. This led to astronomers following up on it, spectroscopically and with high resolution, by the large and powerful Magellan telescope: a 6.5 meter diameter telescope.
Surprisingly, the elements found within this star – if you look at them element-by-element – didn’t exhibit the typical abundances we normally find in stars when we looked at their abundances relative to one another. Some elements were greatly abundant compared to the amount of iron found, including enhanced abundances of strontium (Sr), Yttrium (Y), Manganese (Mn), Nickel (Ni), and Zinc (Zn), among others. Other elements, such as sodium (Na), titanium (Ti), scandium (Sc), and barium (Ba), showed tremendous deficiencies compared to most other typical stars. It’s almost as though this one star, J0931, is telling us multiple different cosmic stories at once.
Lesson #1: All stars aren’t enriched uniformly; at least some stars are partially enriched in certain elements and partially deficient in others.
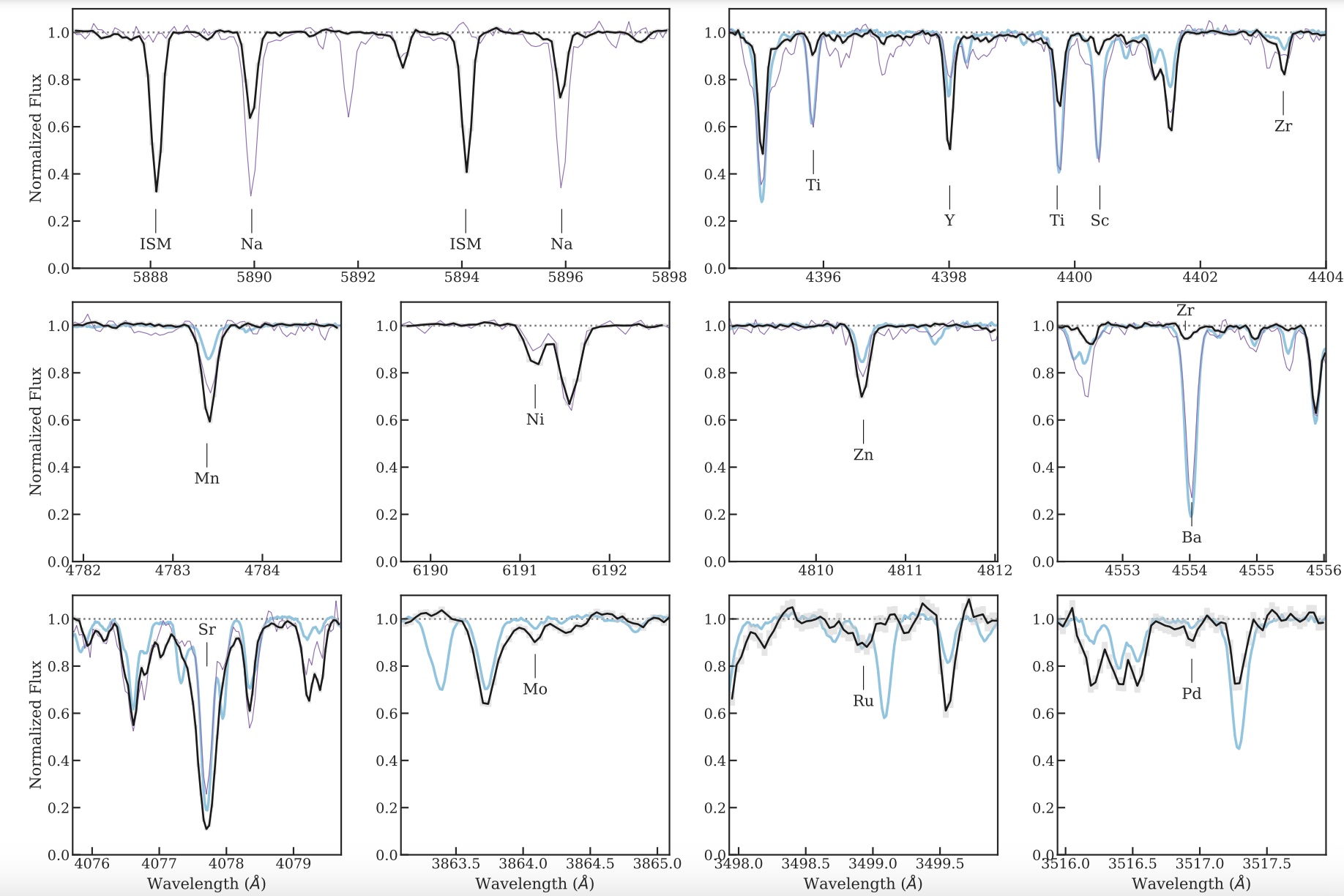
However, we have to accept that this star exists, and even if there are multiple contributing factors to how it obtained the properties it now displays, there’s only one cosmic story that led to its existence. In a new paper just accepted into the Astrophysical Journal, astronomer Alex Ji and his collaborators put forth a fascinating potential explanation that could account for this system with just a single cosmic story: this star formed very early on in the Universe’s history, from nearly-pristine material that had only been slightly enriched.
But nearby, shortly before this star formed, a lone, early, and asymmetric supernova event occurred, seeding the interstellar medium irregularly, with some elements preferentially becoming enriched in certain directions over others, while some failed to become as enriched in those same directions. If there was some sort of directional emission of the detritus arising from the supernova, and then stars swiftly formed from the material that included this irregularly enriched and recycled stellar debris, it would be possible to form stars such as J0931: stars that were not formed from a well-mixed sample of the interstellar medium, but from an unusual sample with some enrichments and some deficiencies over the cosmic average at the time.
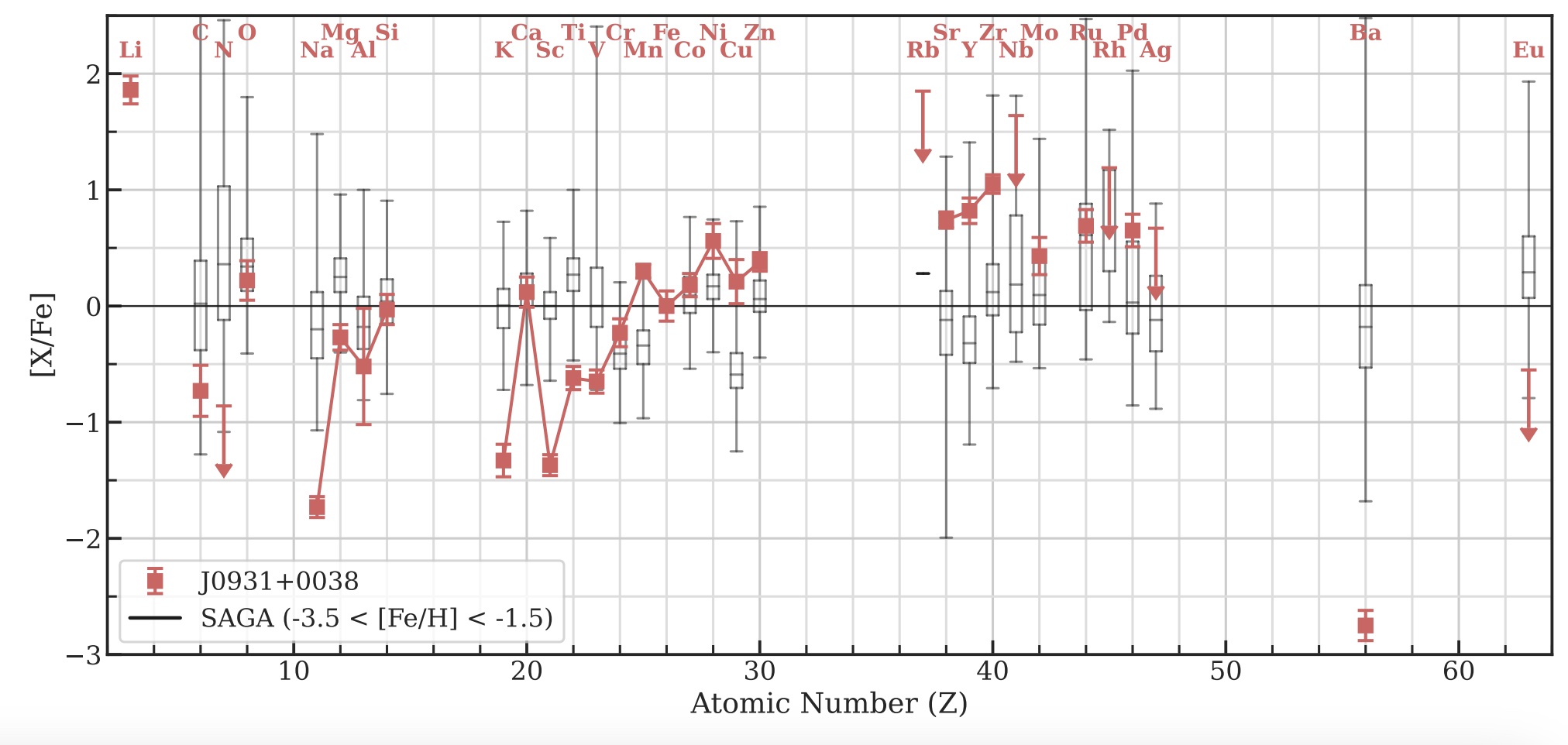
The reason so few stars are expected to display properties like J0931 is because, over time, three things happen.
- Ejecta from various cataclysmic events, even highly asymmetrical ones, will eventually become well-mixed with the rest of the interstellar medium over time.
- Most of the stars that do form in the Universe do so in the aftermath of many violent events, and also form in preferentially enriched areas of the Universe.
- And that even initially pristine regions that have never formed stars, over time, will get “mixed up” with the recycled, polluted material.
Therefore, there’s only a brief window of time where these heavily, asymmetrically enriched/enhanced stars can form, and only the earliest of these windows will give rise to low-metallicity stars like J0931 that also display these properties.
Moreover, whenever stars form, they form with a wide variety of masses, and with higher mass comes a shorter lifetime. It’s only the least massive stars – and if we’re talking about stars that formed 12-13 billion years ago, that’s only the stars that are less massive than the Sun – which will still persist even today.
Lesson #2: Supernova events can produce an asymmetric distribution of elements, and new stars can then form with that unusual distribution imprinted onto them.
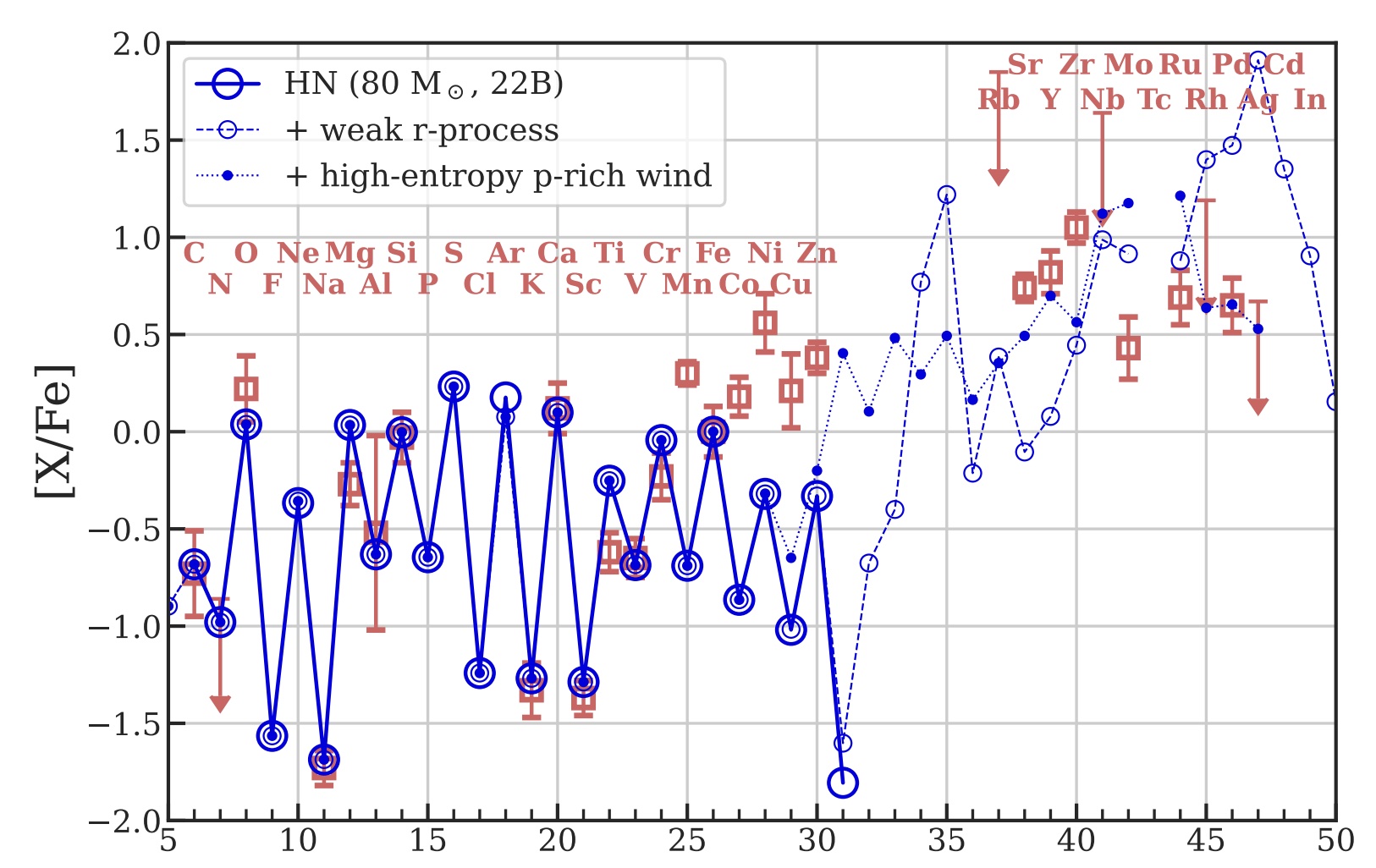
When we look at the precise distribution of elements that exists within J0931, the situation gets even more troubling, as we find very quickly that we don’t have a supernova model that can predict all of the observed elemental abundances simultaneously, alongside one another. A supernova or hypernova that arose from an ~80 solar mass star could do the trick for many of the elements, but not all of them. When you view the data all together, it is suggestive of a scenario where the material that formed this star was composed of:
- a mix of previously enriched, well-mixed, nearly-but-not-quite pristine material,
- that was augmented by an asymmetric predecessor supernova,
which could then explain the odd-even element relative observed abundances of the different elements.
However, there’s again a puzzle that emerges with this scenario, as our best models for stellar evolution indicate that stars of between about 40 and 140 solar masses shouldn’t go supernova at all, but would rather directly collapse to black holes without a stellar cataclysm of any type. It’s possible that this star has something new to teach us: which is that nature, at least sometimes for stars in this mass range, does something different than our naive expectations would lead us to believe.
Lesson #3: Our simplest models for when stars undergo direct collapse versus when they explode in a supernova do not match up with all of our observations, and will need refinement/improvement moving forward.

There are other lines of evidence that suggest that a star’s fate is not exclusively determined by factors like mass and metallicity, which is commonly assumed. We observed a star of 25 solar masses simply appear to “wink out” of existence, with no remnant, cataclysm, or emissions of any type left behind, even though stars of that mass should go supernova, not directly collapse. In the early Universe, we have evidence from observed black holes that there are probably clumps of matter in the 10,000-100,000 solar mass range that simply collapse to form the seeds of supermassive black holes, without any sort of intermediate stage where they formed stars.
It’s possible, perhaps even probable, that what we are seeing is hinting to us that our present understanding of stellar lives and deaths, particularly at high masses and in the more-pristine past, is far too naive and requires significant refinement. Perhaps much of the astrophysics behind the massive objects created in the early Universe is determined by factors we have not been modeling correctly, and that asymmetric enrichment of early stars was more common than we presently realize. Regardless of that, identifying J0931, and hopefully more stars like it, truly is shining a light onto how heavy elements were created at incredibly early times in our cosmic history, which leads to the final unexpected lesson.
Lesson #4: By identifying the oldest, lowest-metallicity stars and measuring them with high-resolution spectroscopy, covering a wide variety of elements, we can learn lessons about how nucleosynthesis worked in the most massive stars from the early Universe.