The Bullet Cluster Proves Dark Matter Exists, But Not For The Reason Most Physicists Think
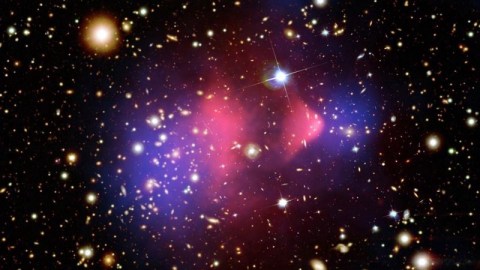
If the gravity isn’t where the matter is, things get into trouble very, very quickly.
The above image, a composite of optical data, X-ray data, and a reconstructed mass map, is one of the most famous and informative ones in all of astronomy. Known as the Bullet Cluster, it showcases two galaxy clusters that have recently collided. The individual galaxies present within the clusters, like two guns filled with bird shot fired at one another, passed right through one another, as the odds of a collision were exceedingly low. However, the intergalactic gas within each cluster, largely diffuse and making up the majority of the normal matter, collided and heated up, emitting X-rays that we can see today. But when we used our knowledge of General Relativity and the bending of background light to reconstruct where the mass must be, we found it alongside the galaxies, not with the intra-cluster matter. Hence, dark matter must exist.
According to the standard line of reasoning, the clusters are composed of dark matter and normal matter in a 5:1 ratio. When they collide, the diffuse normal matter collides, sticks together, and heats up, while the clumps (galaxies) and dark matter pass through, creating the observed effects.
But, as with any great idea, all the alternatives must be considered. The X-rays don’t lie: there really is that much matter in between the two separated clusters today, so any argument to the contrary must be discarded. The idea that there are ultra-compact, invisible clumps of normal matter within the clusters is intriguing, but a systematic set of observations and analyses indicate that it can’t be nearly enough to explain the observed effects. And the idea that this is unique to this one cluster in the Universe is disproven by the large number of other colliding clusters that have since been found, and observed to exhibit the same effects.
Perhaps, then, there’s an intriguing alternative that we have to consider: that under the right conditions, gravity exhibits non-local effects. This sounds crazy, but it’s a hallmark of many physically important processes, such as the quantum Universe. The basic idea is that the effects of gravitation occur in different locations from where the majority of the matter is. Non-local gravity theories are excellent at reproducing the successes of modified gravity ideas, such as the rotation curves of galaxies. While a recent paper used constraints from gravitational waves and gamma rays to rule out some variants of modified gravity where they travel along different paths, one of the survivors is MOG: a theory of gravity with non-local effects. (Non-local means its effects are not wholly located where the sources are located.)
But as compelling as this is, it cannot be right, and it only requires a thought experiment to understand why.
Imagine what it would take to have two galaxy clusters, post-collision, exhibit an effect where most of the matter is in the central region where the collision took place, but where most of the gravitational effects are located centered elsewhere. It would require that gravity and mass not line up together. This is, in fact, what we see when we look at the normal matter alone in galaxy clusters: the places where we see/trace the gas and where we reconstruct the mass, from gravitational lensing, don’t perfectly align.
Either gravity behaves non-locally, or there’s some unseen form of mass: dark matter. But there’s an easy way to tell these two apart! Simply take a look at galaxy clusters that aren’t in the process of colliding, or look at two nearby clusters that are headed towards one another, but haven’t merged yet. If dark matter is the correct explanation, the gravitational lensing signal should trace the matter distribution: everything should be local. But if non-local gravity is the answer, there should be gravitational effects seen where the matter isn’t located.
Thankfully, we have that data, and we have an answer.
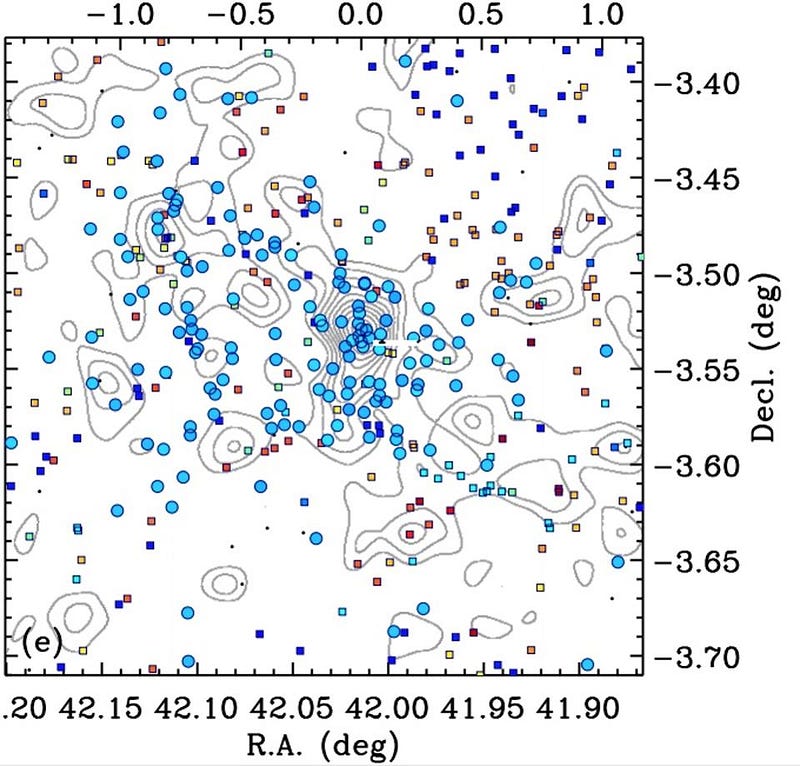
When your cluster is undisturbed, the gravitational effects are located where the matter is distributed. It’s only after a collision or interaction has taken place that we see what appears to be a non-local effect. This indicates that something happens during the collision process to separate normal matter from where we see the gravitational effects. Adding dark matter makes this work, but non-local gravity would make differing before-and-after predictions that can’t both match up, simultaneously, with what we observe.
Interestingly, this argument has been made for over a decade, now, with no satisfactory counterargument coming from detractors of dark matter. It isn’t the displacement of gravitation from normal matter that “proves” dark matter exists, but rather the fact that the displacement only occurs in environments where dark matter and normal matter would be separated by astrophysical processes. This is a fundamental issue that must be addressed, if alternatives to dark matter are to be taken seriously as complete theories, rather than ideas in their infancy. That time is not yet at hand.
Ethan Siegel is the author of Beyond the Galaxy and Treknology. You can pre-order his third book, currently in development: the Encyclopaedia Cosmologica.