This Is How Astronomers Will Finally Measure The Universe’s Expansion Directly
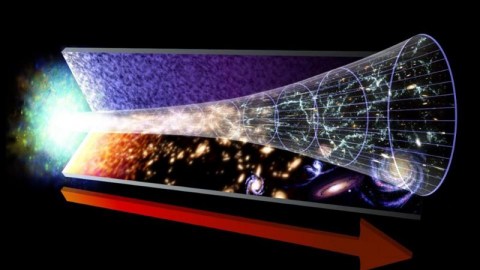
And if the data is good enough, we can determine that it’s accelerating directly, too, silencing the last remaining doubters.
If you want to understand what the Universe is made of, what its fate is, or how long ago the Big Bang occurred, there are just two pieces of information you need. According to the science of physical cosmology, all you need to measure is:
- how quickly the Universe is expanding today, and
- how the expansion rate changes over time,
and that information allows you to reconstruct the Universe’s composition, history, and evolution as far into the future as you like.
Up until now, there’s been a tremendous amount of controversy surrounding all of these issues, as different teams using different methods arrive at different answers. But they all have one thing in common: all of their measurements rely only on indirect methods of determining how the Universe has expanded over time. But with a new generation of telescopes arriving in the 2020s, astronomers will at last gain the capability to measure the expansion rate directly. Here’s the incredible science behind it.
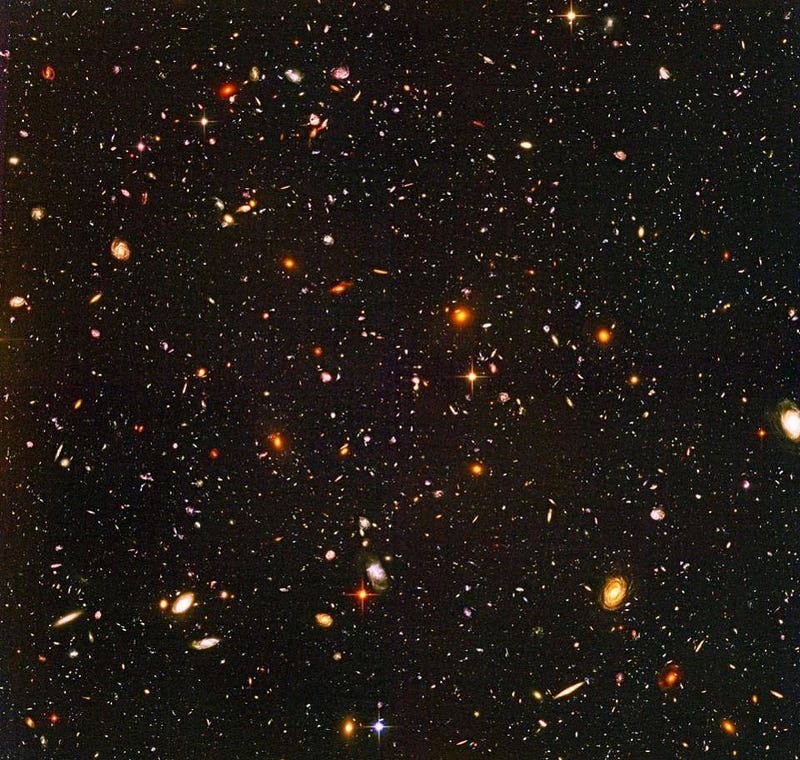
In an expanding Universe, the light that a distant galaxy emits will appear different than the light received by a faraway observer. At any particular instant, the light emitted by stars and galaxies will have certain properties. In particular, that light will behave like it’s a sum of many different blackbodies — the way perfectly dark objects radiate when they’re heated to a certain temperature — superimposed atop one another.
If this were the only light the Universe gave us to observe, measuring how the Universe expands would be extremely challenging. Even if we discovered clever methods to measure the distances to these far-flung objects, we still wouldn’t be able to accurately measure the effects of the expanding Universe. As the Universe expands, the emitted light stretches as it travel from the source to the observer, but without knowing the intrinsic properties of that light, we couldn’t measure the amount of stretching to any reasonable precision.
Fortunately, our Universe isn’t simply composed of stars and galaxies that radiate at a specific temperature; it’s also made of atoms. Atoms have the spectacular property that they only absorb or emit radiation of extraordinarily specific wavelengths: wavelengths that correspond to the atomic and molecular transitions inherent to those specific atoms.
By taking the light from all objects, from our Sun to nearby stars to even the most distant galaxies and quasars, we can identify those absorption and emission features caused by the atoms within those objects. There are two effects — the motion of the light source relative to the observer and the expansion of space over the course of the light’s journey — that combine to determine the amount that the distant light shifts by over the time that it travels to our instruments.
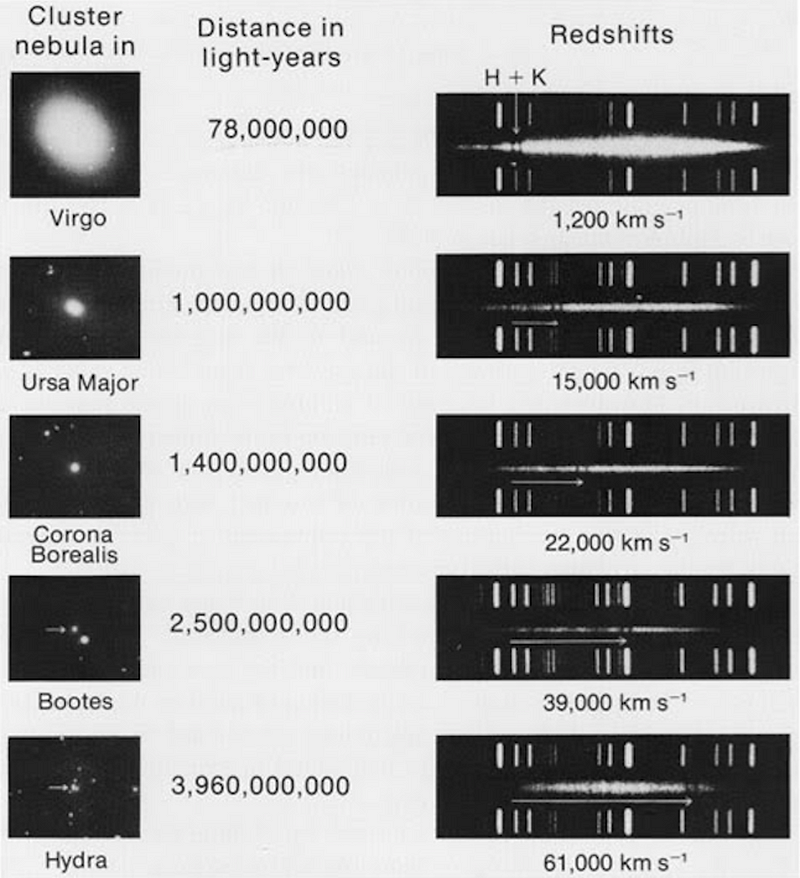
By combining distance measurements with redshift measurements, we can reconstruct the expansion of the Universe. That’s one of the major classes of methods used to measure how quickly the Universe expands, and it encompasses all sorts of different ways to measure the distance to a variety of objects.
When we combine all the data from the full suite of objects we can reliably measure both distances and redshifts to, we come up with some very tight constraints on how the Universe has expanded over time. Because matter and radiation dilute in specific fashions as the Universe expands, while dark energy remains indistinguishable from a cosmological constant (with a constant energy density), we can use all of the information, combined, to learn what the Universe is made of, how fast it’s expanding today, and how that expansion rate has evolved over time.
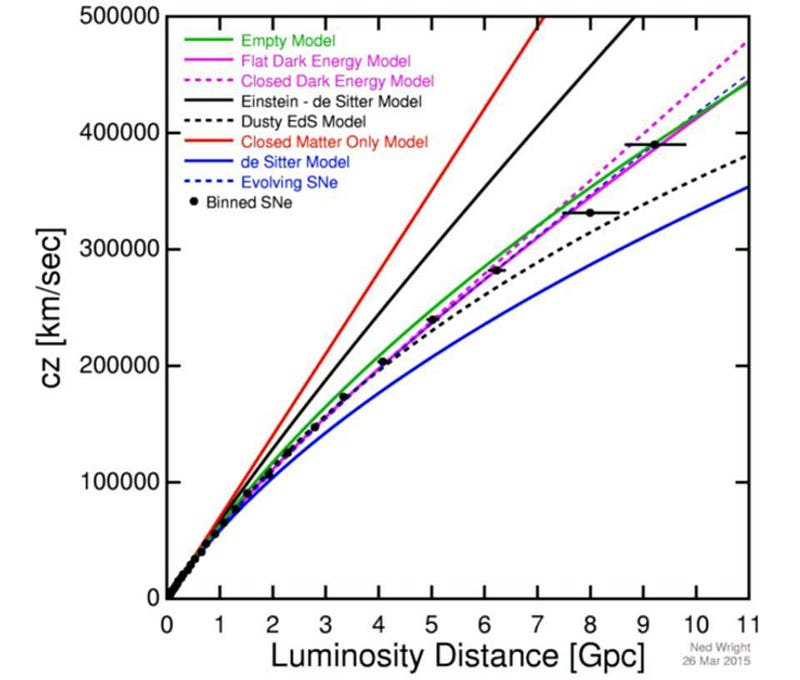
It’s a monumental achievement for cosmology, and has given us answers (albeit, with uncertainties and controversies associated with them) to all of these questions to unprecedented precision. However, there’s only so much confidence one can have in these indirect measurements. In astronomy, the objects we see are often so far away and so grand in scale that, on human timescales, we have no way to measure how they change in real-time.
If the fabric of space is like a ball of dough, and the individual galaxies within the Universe are like raisins, then the expanding Universe is like the dough when it leavens. The raisins (galaxies) all appear to move away from one another, with more distant raisins (galaxies) appearing to recede more quickly. But this observation is primarily due to the fact that the dough (Universe) is expanding. The raisins (galaxies) are actually stationary with respect to their local position; it’s just that the dough (space) between them is expanding over time.
This is why, by measuring the redshifts and distances to a slew of objects — objects at a variety of different distances and redshifts — we can reconstruct the expansion of the Universe over its history. The fact that a whole slew of disparate data sets are all consistent with not only one another but with an expanding, evenly filled Universe in the context of relativity, that gives us the confidence we have in our model of the Universe.
But, just as we didn’t necessarily accept gravitational waves before they were directly measured by LIGO, there’s still the possibility that we’ve made a mistake somewhere in inferring the properties of the Universe. If we could take a distant object, measure its redshift and distance, and then come back at a later time to see how its redshift and distance had changed, we’d be able to directly (instead of indirectly) measure the expanding Universe for the first time.
Given that our best model of the Universe is that it’s 13.8 billion years old, it’s easy to see how it could be challenging to measure an appreciable amount of expansion over timescales that human beings are capable of measuring. If we were to take the most distant galaxies and quasars we can measure — objects that are tens of billions of light-years away — we’d predict that the expected change in redshift-over-time is the equivalent of 1 cm/s per year.
Even with today’s most powerful telescopes, we can only measure redshifts to a resolution of about 100-to-200 cm/s, which means we’d have to wait centuries to even begin to measure changes in how we view these distant objects. Despite the discovery of a large number of distant objects, we simply don’t have the technological capabilities of making astronomical measurements to the needed precisions.
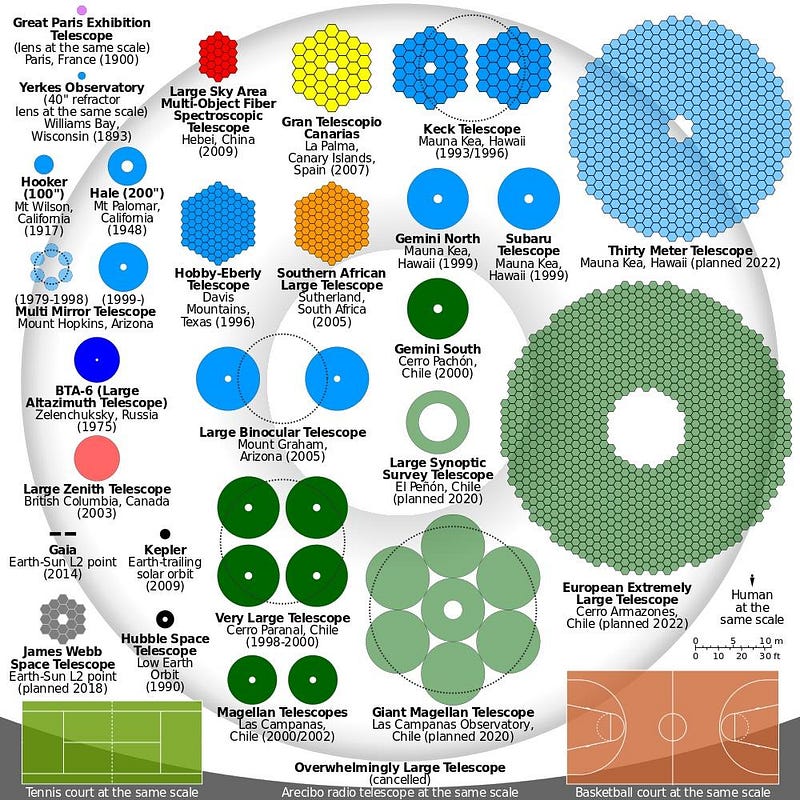
But when we move from having 10-meter class telescopes to 30-meter class telescopes, with approximately:
- 3-to-4 times the resolution,
- around 10 times the light-gathering power,
- advances in adaptive optics that compensate for the atmosphere,
- and new developments in quantum optics that enable us to record ultra-stable spectra,
the European Extremely Large Telescope (ELT) will likely be the first to make this measurement directly. With the recent new discoveries of many new ultra-distant quasars at a variety of redshifts (a trend that’s expected to increase when the Large Synoptic Survey Telescope becomes operational), the ELT should be able to detect the expansion directly.
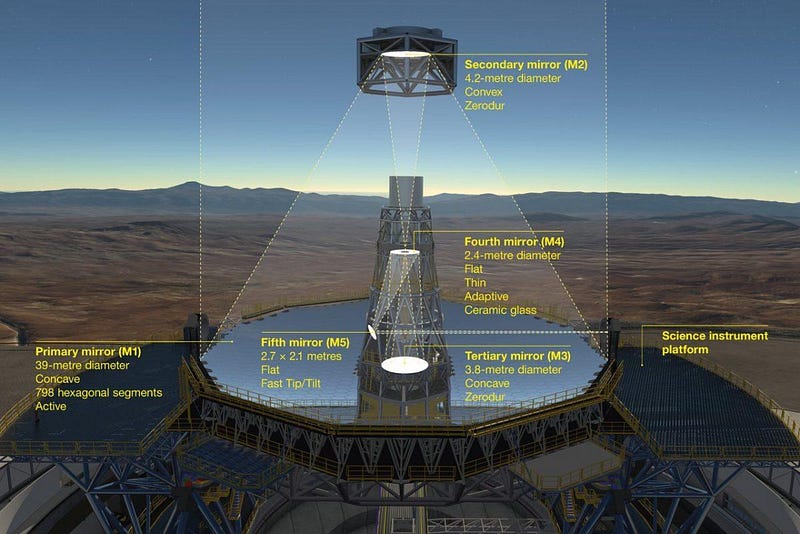
The ELT is expected to come online in the mid-2020s, and should be capable of measuring the redshifts of individual objects with about a factor of 10 improvement in precision over today’s best instruments. With thousands to tens of thousands of quasars expected to be discovered and well-measured at the large distances needed to see this effect, the ELT should be sensitive to changes in redshift that correspond to additional shifts of just 10 cm/s in overall magnitude.
This represents an improvement of a factor of 10-to-20 over existing telescopes, and means that if we wait just a decade (or perhaps a decade-and-a-half) once the ELT comes online at full power, we should be able to measure the expansion of the Universe directly.
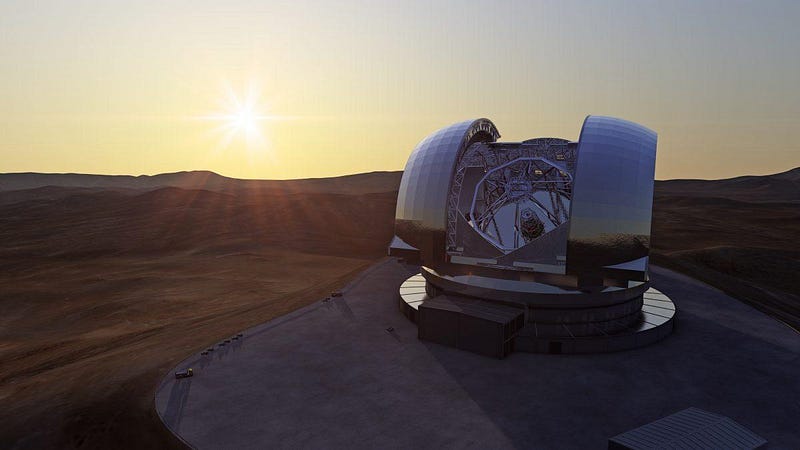
The key term you’ll want to remember as we move into the mid-2030s, the earliest possible time this detection could robustly be made, is redshift drift. By measuring how cosmic redshifts change over time — something we’ve never been able to do to date — we’ll be able to test a magnificent array of aspects about our Universe. This includes:
- whether the cosmic expansion follows the predictions of theoretical cosmology for an evenly filled Universe governed by General Relativity,
- whether dark energy is truly a cosmological constant or whether it changes in strength over time/distance,
- whether these changes favor a faster (73 km/s/Mpc) or a slower (67 km/s/Mpc) expansion rate,
- and whether the flux coming from these distant objects is stable to the necessary precisions (with changes no more than 0.0001% over a decade) to enable the detection of flux drift as well.
By 2040 at the latest, we should be able to directly confirm the expansion of the Universe, putting our understanding of the cosmos to the ultimate test.
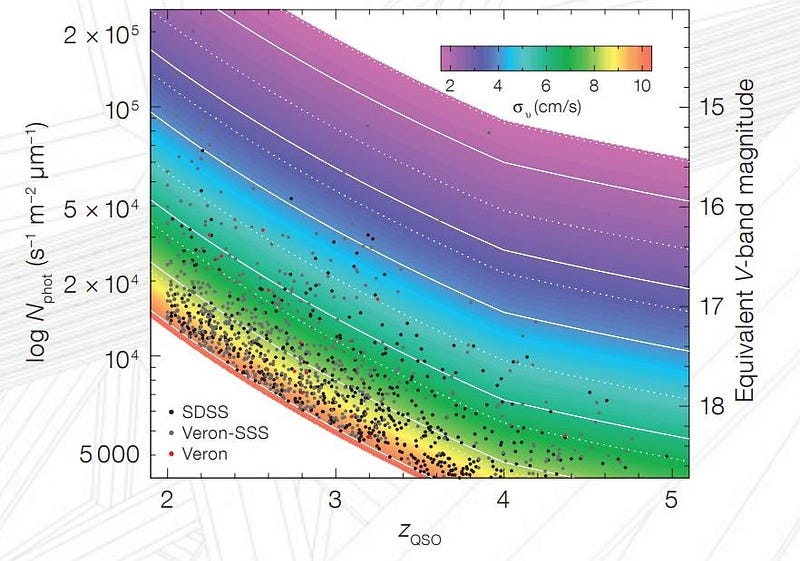
There’s a terrible myth about science that is pervasive among the general public: that it’s very risky to build a bigger, larger, more powerful apparatus to probe the Universe as never before. That if we go to higher energies, lower temperatures, larger apertures, or other scientific extremes, that our searches might be fruitless and we’ll have wasted a tremendous amount of time, money, and effort that could be better spent.
The truth of the matter is that pushing the boundaries of what we’re capable of discovering is how we gain the new knowledge that enables us to develop tomorrow’s technologies. Whether we discover something new or not is for nature to decide; we have no control over that. What we do have control over is whether we invest in going where no human has ever gone before, in learning what humans have only speculated about, and in expanding the frontiers of what’s possible on Earth.
For nearly a century, we’ve known that the Universe is expanding. In 20 years, tops, we’ll have the direct evidence to know exactly how it’s happening.
Ethan Siegel is the author of Beyond the Galaxy and Treknology. You can pre-order his third book, currently in development: the Encyclopaedia Cosmologica.