What’s so special about special relativity?
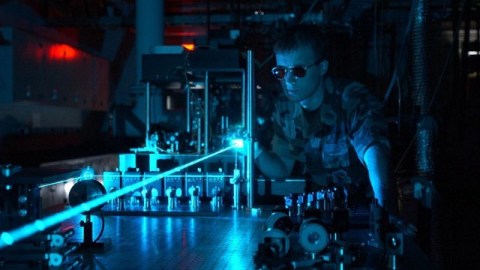
Einstein’s first great revolution happened way back in 1905. It still puzzles many amateurs and professionals alike even today.
“Each ray of light moves in the coordinate system ‘at rest’ with the definite, constant velocity V independent of whether this ray of light is emitted by a body at rest or a body in motion.” –Albert Einstein, 1905
There are only a few ideas that are powerful enough to shape our entire picture of the Universe and how it works: gravitation, the laws of motion, electricity and magnetism, quantum mechanics. Yet a little over 100 years ago, the laws of motion — first set out by Newton, who built on ideas from Galileo — were running into trouble. Galileo had stated, back in the early 1600s, that there’s no absolute and constant state of rest; no one observer would have a “privileged” position. But it was also discovered that the speed of light was constant, no matter who the observer was or how they were moving. These two ideas might seem compatible, but Newton’s laws of motion couldn’t fit them together. It took a new view of the Universe, and Einstein’s relativity, to make it work. Here’s how.
Imagine you’re on a train, moving at, say, 100 miles per hour (45 m/s), and you shoot a cannonball from it at an additional 200 mph (89 m/s). From your perspective, on the train, you see the cannonball move at 200 mph (89 m/s). From someone else’s perspective, on the ground, they’ll see the cannonball move at 300 mph (134 m/s), since the speeds of the train and the cannonball should add. Galileo predicted this much, and the results still hold up today. But if you replace the cannonball with light, everything goes wonky. Light travels at 670,616,629 mph (299,792,458 m/s), and if you shoot a beam of light out from the train, you, a person on the ground, a person in an airplane, a rocket, or someone moving at any other speed will see the same thing: light traveling at that same universal speed, the speed of light.
The way this was discovered wasn’t easy. Back in the late 1800s, the fastest thing we knew of in constant, controlled motion was the Earth itself. It rotates on its axis at about 465 m/s at the equator, but it orbits the Sun at about 30,000 m/s as it moves through space. It’s fast enough that this second speed is approximately 0.01% the speed of light. That might not seem like a lot, but it’s fast enough that there are experiments we can perform to see if the speed of light changes by that tiny amount.
If you fly from Paris to New York and back in an airplane into a headwind followed by a tailwind of equal magnitude, it takes slightly longer for that plane to arrive than if there were no wind at all. If light obeyed this same principle, it would take slightly longer for a light wave to travel in the direction of the Earth’s orbital motion around the Sun than for a direction perpendicular to that. In the 1880s, Albert A. Michelson constructed a series of ultra-sensitive interferometers set up to exploit exactly this fact. As the interferometer rotated into, perpendicular to, and against the Earth’s direction of motion, there should have been shifts in the interference pattern produced by the beams of light as they moved through space. But no shift was ever observed; this experiment returned a null result.
This was perhaps the most important null result in the history of physics, since it meant that the speed of light was constant to any and all observers. As Chad Orzel says, the big advance of Einstein’s relativity was to state that the laws of physics do not depend on how you’re moving, and that one of those laws is the fact that the speed of light is a constant to everybody! The thing that changes for different observers moving at different speeds is not how fast a light beam appears to move, but rather how fast one another’s clocks appear to run and how long distances appear to be between objects moving at various speeds. These transformations of length contraction and time dilation — known as the Lorentz transformation — have been borne out by experiment after experiment.
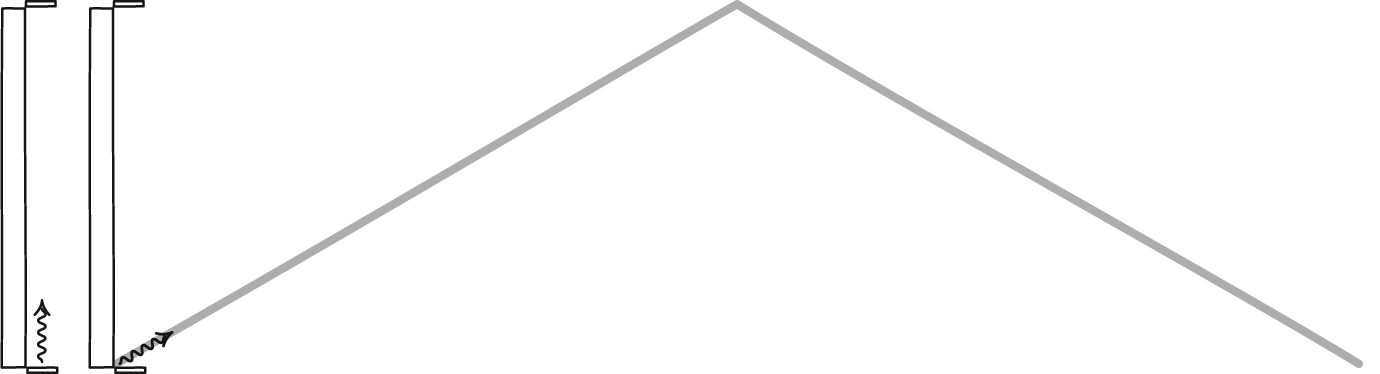
The part that makes special relativity so “special” is because these laws apply to everyone, everywhere at every time, including deep inside gravitational fields of all magnitudes. But to explain that, you need a more general theory: Einstein’s theory of general relativity. The rules of special relativity are a special case of general relativity, where you can ignore the gravitational fields. Special relativity was discovered first, by Einstein, in 1905. Two years later, in 1907, Michelson was awarded the Nobel Prize for his interferometer experiments proving the constancy of the speed of light. It wasn’t until 1915 that Einstein completed his general theory of relativity, which was verified by the gravitational bending of starlight observed during a solar eclipse in 1919.
The special advance of special relativity was combining the fact that the speed of light is constant with the fact that observers in all reference frames perceive the same laws of nature. This still holds up today! So rest assured, no matter how you’re moving or where you are, no matter when you look or how you do it, the laws of physics are the same for you as they are for anyone and everyone else. And that’s a fact of the Universe that’s pretty special, even 111 years later.
This post first appeared at Forbes, and is brought to you ad-free by our Patreon supporters. Comment on our forum, & buy our first book: Beyond The Galaxy!