Are we living in a baby universe that looks like a black hole to outsiders?
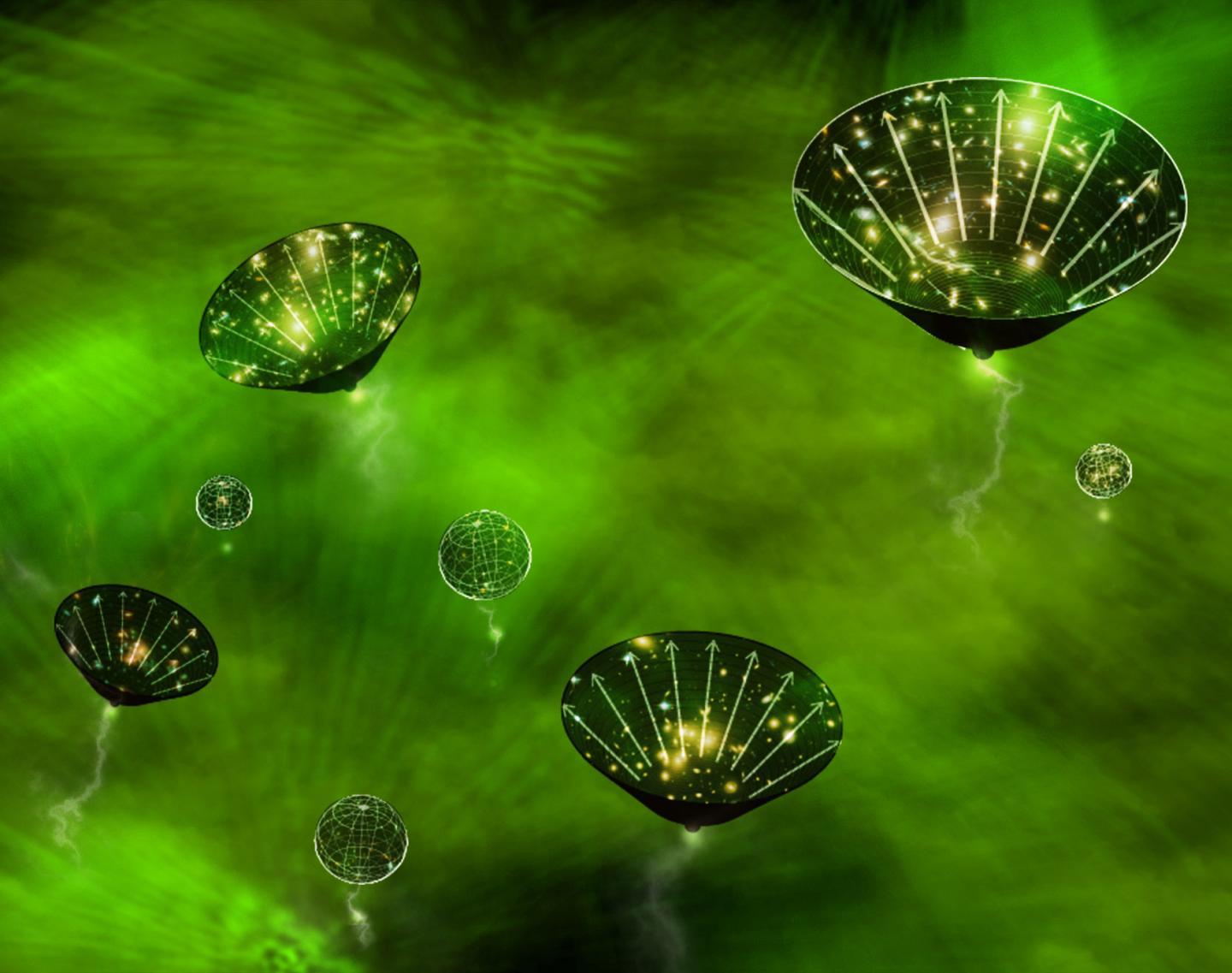
- Our Universe appears to be expanding and cooling, having originated some 13.8 billion years ago in a hot Big Bang.
- However, it’s plausible that what we see from inside our Universe is simply the result of being inside a black hole that formed from some parent Universe.
- If the black holes that form in our cosmos give birth to baby Universes, perhaps we arose from the formation of a black hole ourselves.
One of the biggest existential questions that has puzzled humanity for as long as humans have been around is simply, “Where did all this come from?” After countless centuries of wondering and speculating, the 20th century brought with it our first scientific answers to that question. We learned that distant objects in the Universe are speeding away from one another: evidence that our Universe is expanding. We discovered that more distant galaxies appear younger, less massive, and with greater rates of star formation: evidence that our Universe evolves with time. And we discovered a near-uniform background of blackbody radiation: evidence of an early, hot, dense, radiation-dominated state. All of these puzzle pieces, when put together, indicate that our Universe originated from a hot Big Bang some 13.8 billion years ago.
But our Universe has a very curious property that not everyone appreciates. If you add up the mass and energy of all the particles contained within the visible Universe, you can ask the question, “How big would the event horizon of a black hole with this mass be?” And the answer, perhaps surprisingly, is very close to the actual horizon size of the observable Universe. Additionally, there’s another related idea, made famous by Stephen Hawking, that each time we create a black hole in our Universe, it could give rise to a “baby Universe” that’s only accessible to an observer that crosses inside that black hole’s event horizon. Could our Universe, then, actually have been spawned by a black hole that was created in some sort of grand “parent Universe,” and do we give birth to a new Universe each time a new black hole is created?
It’s a fascinating idea worth exploring. Here’s what the science, at present, has to say about it.
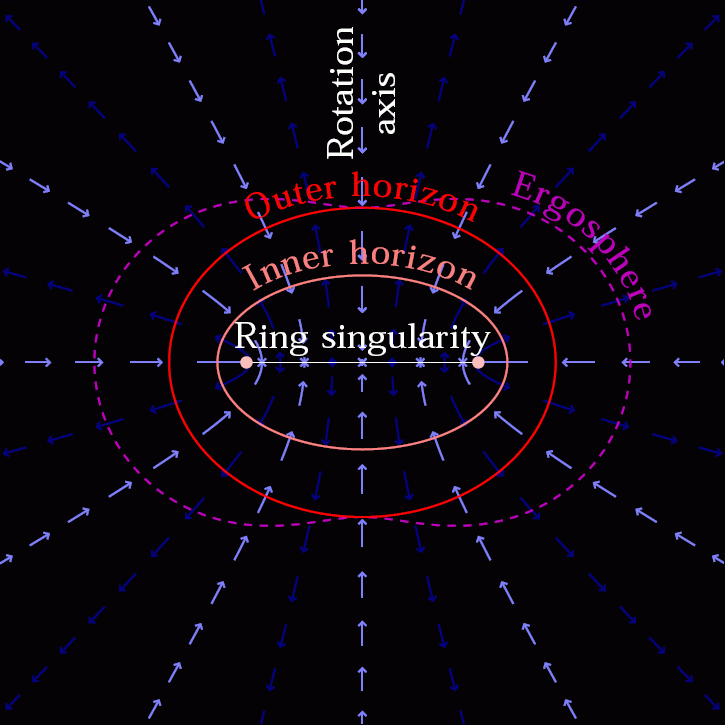
Credit: Andrew Hamilton/JILA/University of Colorado
The defining feature of a black hole is the existence of an event horizon: a boundary that tells a very different story for an object outside of it versus one inside of it. Outside of a black hole’s event horizon, any object will experience its gravitational effects, as the space will be curved by the black hole’s presence, but it can still escape. If it moves fast enough or accelerates quickly enough in the proper direction, it won’t necessarily fall into the black hole, but it could break free of the black hole’s gravitational influence.
Once an object crosses over to the other side of the event horizon, however, it’s immediately doomed to be subsumed into the black hole’s central singularity. Because the fabric of spacetime is severely curved inside a black hole, an infalling object will reach the singularity within seconds of crossing the event horizon, growing the black hole’s mass in the process. To someone located outside the event horizon, the black hole appears to form, gain mass, and grow over time.
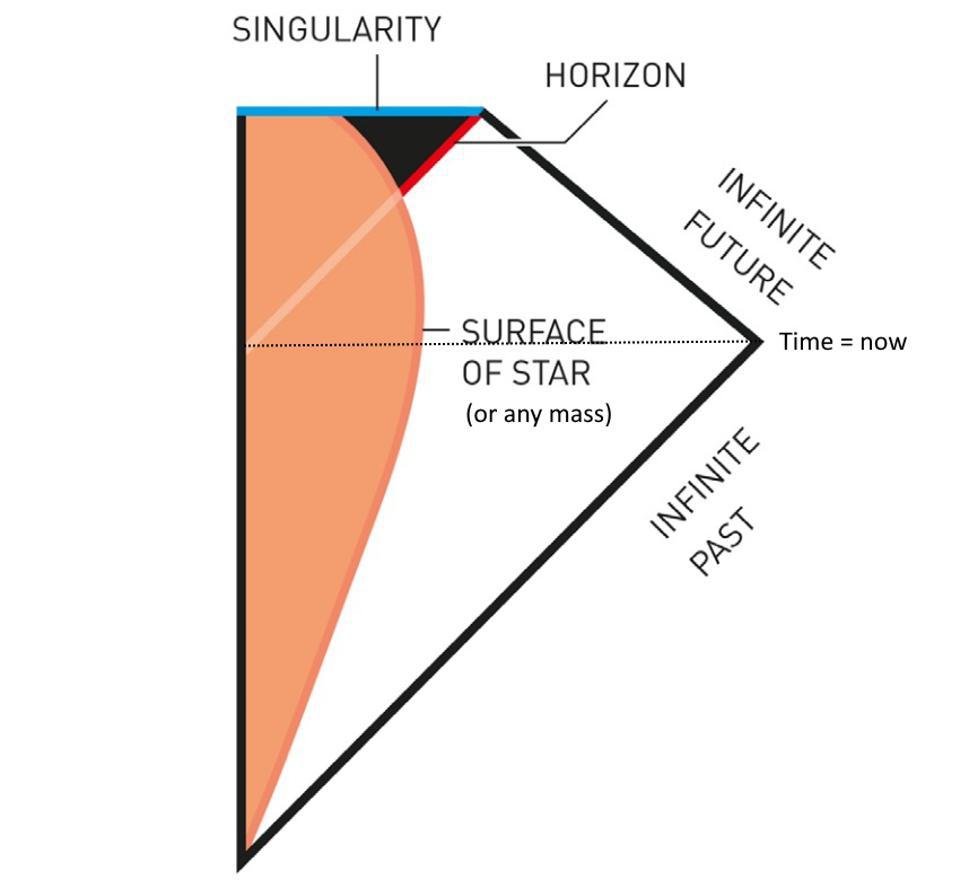
Credit: J. Jarnstead/Royal Swedish Academy of Sciences; annotations by E. Siegel
What does this have to do with our Universe, though? If you were to take all of the known, measurable forms of matter and radiation in the observable Universe, you’d have to add up all of the following:
- normal matter, made from protons, neutrons, and electrons,
- neutrinos, ghostly fundamental particles that rarely interact with normal matter,
- dark matter, which dominates the Universe’s mass but has so far eluded direct detection efforts,
- photons, or particles of light, which carry energy from every electromagnetic event throughout cosmic history,
- and gravitational waves, which are created every time a mass moves and accelerates through the curved fabric of spacetime.
At the farthest limits of what our instruments can possibly detect, we can see up to about 46 billion light-years away in all directions. If you add up all the energy from all of these forms throughout the entire observable Universe, you can arrive at an equivalent “mass” for the Universe using Einstein’s most famous relation: E = mc².
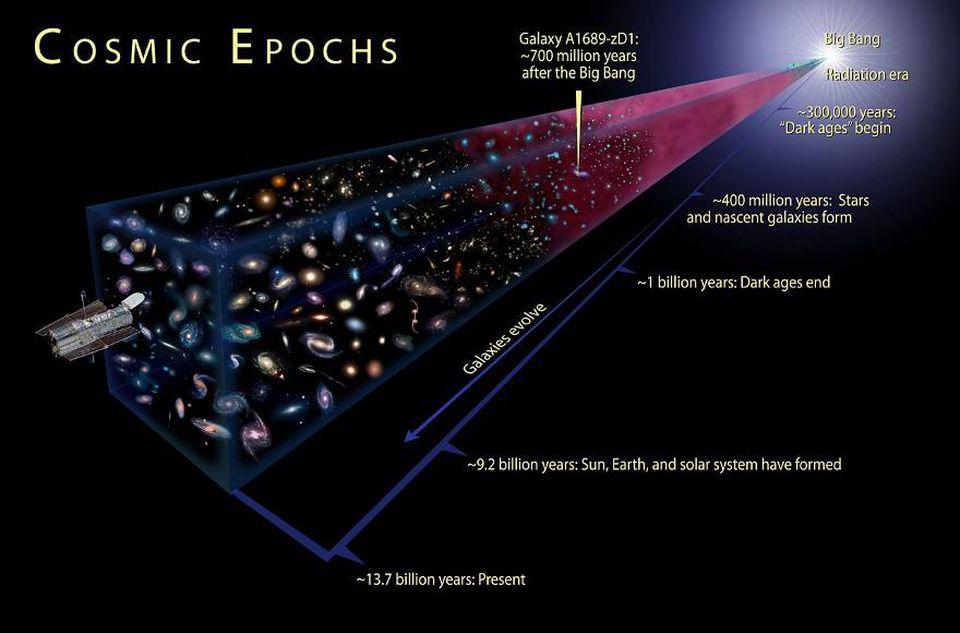
Credit: NASA/ESA/A. Feild (STScI)
Then, if you like, you can ask a rather profound question: if the entire Universe were compressed into a single point, what would happen? The answer is the same as it would be if you compressed any large-enough collection of mass or energy into a single point: it would form a black hole. What’s remarkable about Einstein’s theory of gravity is that if this collection of mass-and/or-energy isn’t charged (electrically) and isn’t rotating or spinning (i.e., without angular momentum), the total amount of mass is the only factor that determines how large the black hole is: what astrophysicists call Schwarzschild radius.
Remarkably, the Schwarzschild radius of a black hole with the mass of all the matter in the observable Universe is almost exactly equal to the observed size of the visible Universe! That realization, on its own, seems like a remarkable coincidence, raising the question of whether our Universe might actually somehow be the interior of a black hole. But that’s only the beginning of the story; as we dive deeper, things get even more interesting.
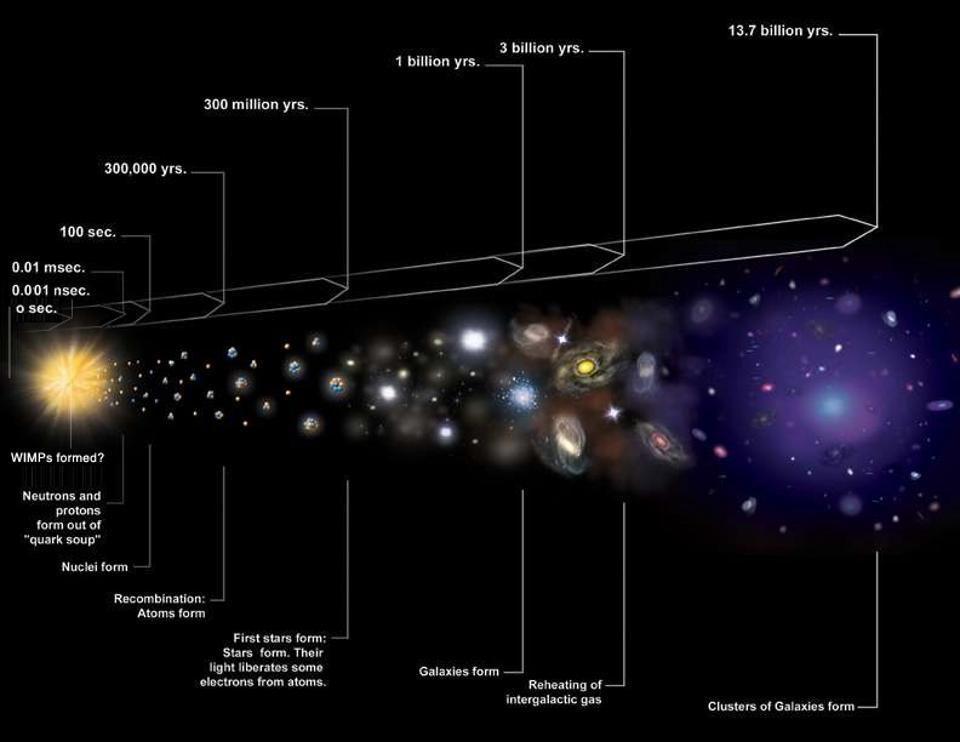
In the mid-1960s, a discovery revolutionized our concept of the Universe: a uniform, omnidirectional bath of low-energy radiation appeared from all locations in the sky. This radiation had the same temperature in all directions, now determined to be 2.725 K, just a few degrees above absolute zero. The radiation had a practically perfect blackbody spectrum, as though it had a hot, thermal origin, and appeared identical to within 1-part-in-30,000 no matter where you looked in the sky.
This radiation — originally called the primeval fireball and now known as the cosmic microwave background — represented critical evidence that our Universe is expanding and cooling because it was hotter and denser in the past. The farther back we extrapolate, the smaller, more uniform, and more compact things were. Going all the way back, this picture of the hot Big Bang appears to approach a singularity, the same condition found at the central interiors of black holes: a location where densities, temperatures, and energies are so extreme that the laws of physics themselves break down.
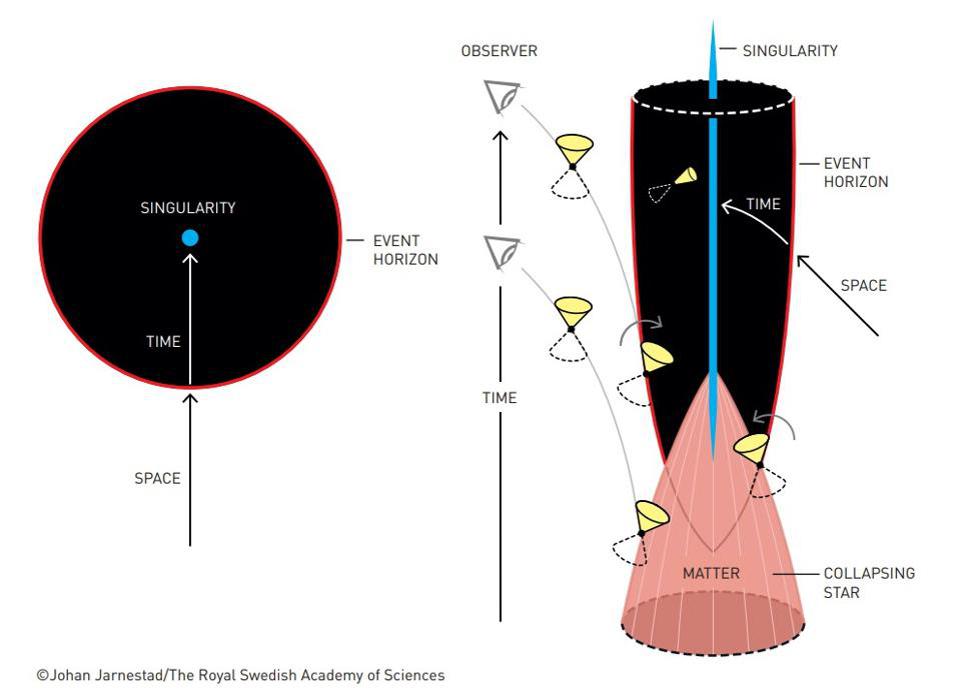
Credit: J. Jarnstead/Royal Swedish Academy of Sciences
Something remarkable happens when you look at the equations that govern a black hole as well. If you start just outside the event horizon and escape to an infinite distance away from the black hole, you’ll find that your distance (r) goes from R, the Schwarzschild radius, to infinity: ∞. On the other hand, if you start just inside the event horizon and track your distance from the black hole to the central singularity, you’ll find that same distance (r) instead goes from R, the Schwarzschild radius, to zero: 0.
Big deal, right?
No, it actually is a big deal, for the following reason: if you examine all the properties of space outside of a black hole’s event horizon, from R to ∞, and compare them to all the properties of space inside the black hole’s event horizon, from R to 0, they are identical at every single point. All you have to do is replace the distance, r, with its reciprocal, 1/r (or, more accurately, to replace all instances of r/R with R/r), and you’ll find that the black hole’s interior is mathematically identical to the black hole’s exterior.
It’s almost like taking a spherical orb that’s 100% reflective — a perfect mirror — and noticing that the entirety of the Universe that’s located outside of that sphere is now contained, albeit distorted, in the mirror image that’s reflected on the sphere’s surface.
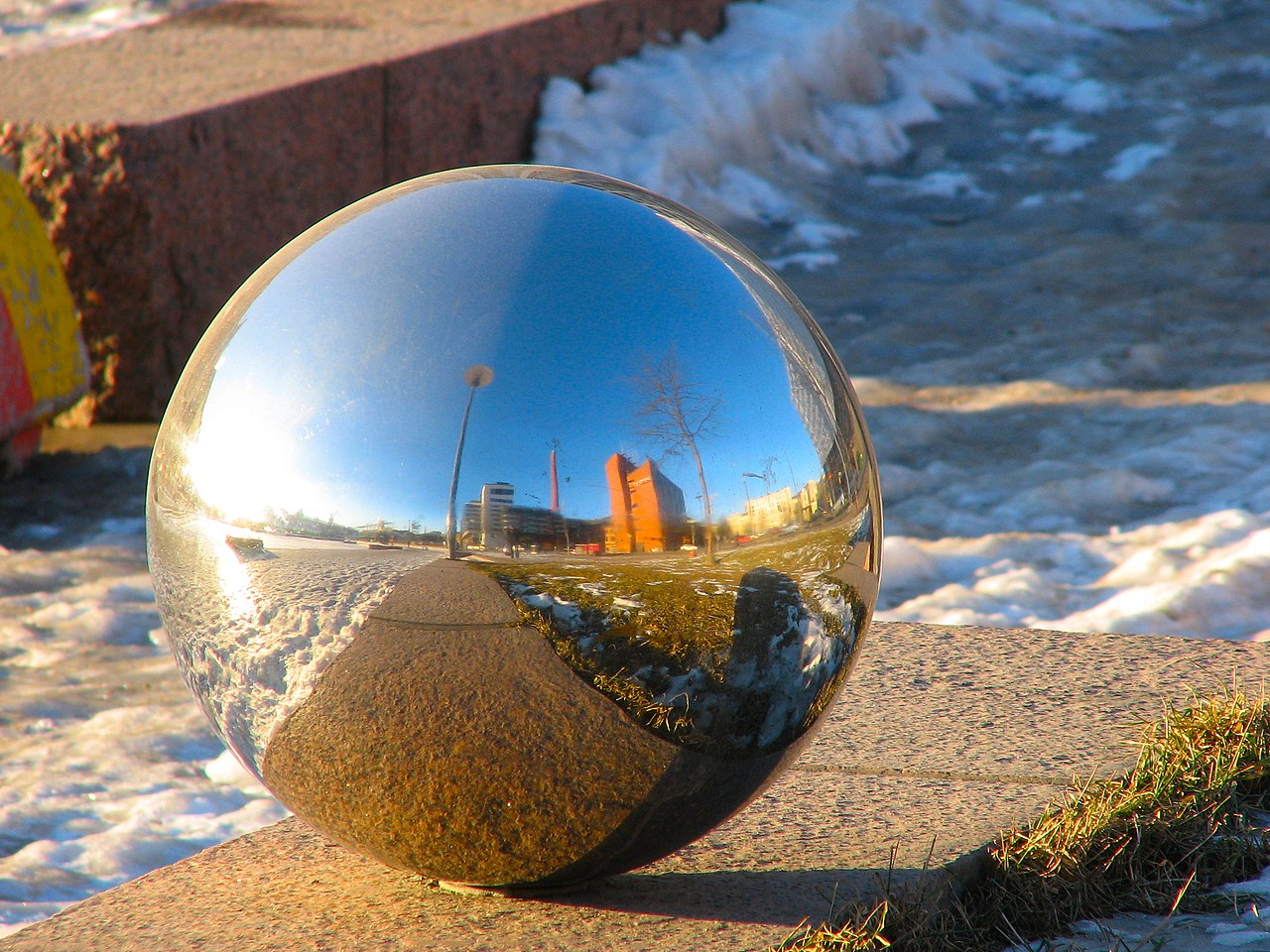
Credit: Antti T. Nissinen/flickr
As our understanding of the Universe has improved and been refined over the past few decades, two new discoveries have rocked the foundations of cosmology. The first was cosmic inflation: instead of arising from a singularity, it now appears that the Universe was set up by a rapid, relentless state of constant, exponential expansion that preceded the hot Big Bang. It’s as though there were some sort of field that provided an energy inherent to space itself, causing the Universe to inflate, and only when inflation ended did the hot Big Bang begin.
The second was dark energy: as the Universe expands and becomes less dense, distant galaxies start to recede from us at an accelerating rate. Once again — albeit, with a much smaller magnitude — the Universe behaves as though there’s some sort of energy inherent to space itself, refusing to dilute even as the expansion of space continues. People have speculated that there might be a connection for as long as inflation and dark energy have both been around.
The fact that there’s a fundamental difference between the expansion rate of the Universe that you infer depending on which of the two classes of methods you use to measure it only strengthens that conjecture. One potential explanation that stubbornly persists for reconciling this discrepancy is that there was a stronger form of dark energy early on: one that existed after the end of inflation but decayed away before the cosmic microwave background scattered off of the primeval plasma for the final time. Perhaps inflation and dark energy have more in common than we realize, and perhaps black holes will provide a critical insight into the nature of that link.
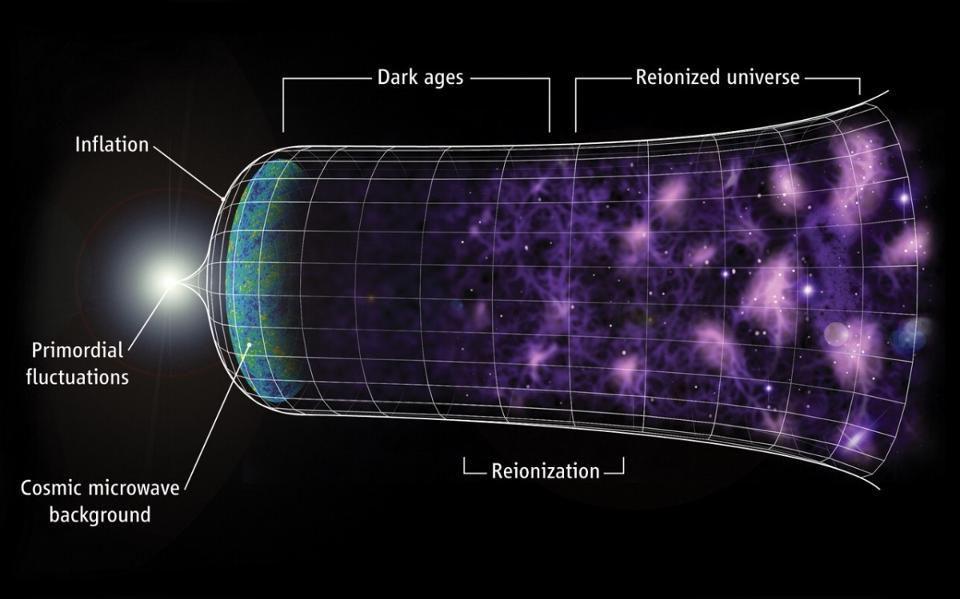
Credit: C.-A. Faucher-Giguere, A. Lidz, and L. Hernquist, Science, 2008
What might that connection be? Once again, black holes could be the answer. Black holes gain mass as material falls into them, and decay, losing mass, via Hawking radiation. As the size of the event horizon changes, is it possible that this changes the “energy” inherent to the fabric of space to an observer located inside the event horizon? Is it possible that what we perceive as cosmic inflation marks the creation of our Universe from an ultramassive black hole? Is it possible that dark energy is somehow connected to black holes as well?
And does this mean that, as astrophysical black holes have formed within our Universe, that each one gives rise to its own “baby Universe” somewhere inside of it? These speculations have been around for many decades, and although we lack a definitive or provable conclusion, there are certainly some mathematically compelling pieces of evidence that suggest a link. Nevertheless, many models and ideas abound, and this line of thought continues to be compelling to many who research black holes, thermodynamics and entropy, General Relativity, as well as the beginning and end of the Universe.
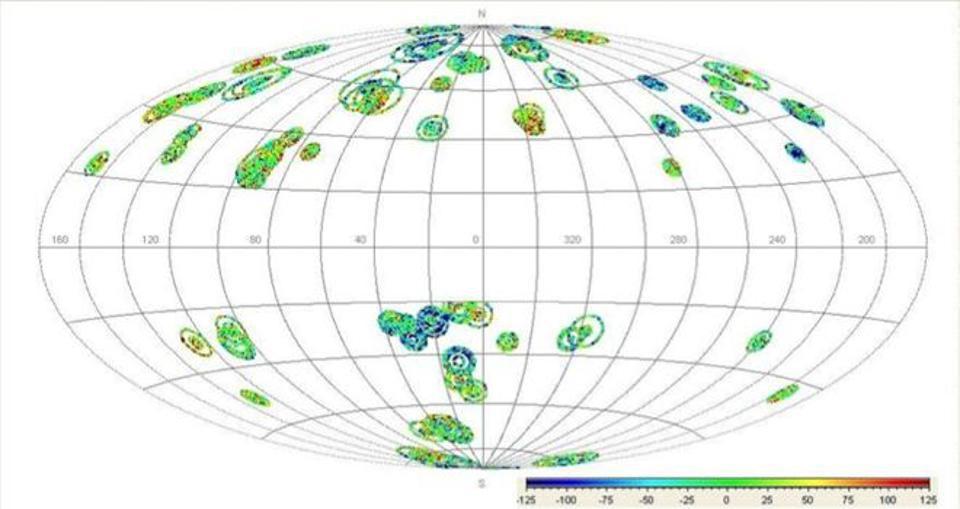
Unfortunately, every physical model put forth — at least so far — has failed to make unique predictions that can do the following three things.
- Reproduce all the successes, like the already-observed phenomena, that the inflationary hot Big Bang has already successfully accounted for.
- Explain and/or account for observed phenomena that the prevailing theory cannot.
- Make new predictions that differ from those predicted by the current leading model, that we can then go out and test.
Perhaps the most famous attempt at this is Roger Penrose’s Conformal Cyclic Cosmology (CCC), which does make a unique prediction that differs from the standard cosmological models: the existence of Hawking points, or circles of unusually low temperature variance in the cosmic microwave background. Unfortunately, these features do not robustly appear in the data, relegating the idea that our Universe was birthed from a black hole — and the idea that black holes give rise to baby Universes — back to one that’s purely speculative.
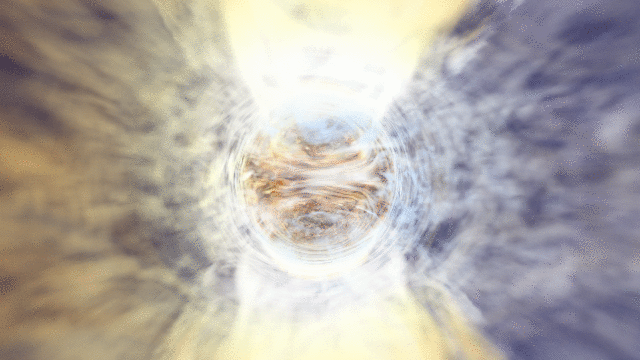
Credit: Andrew Hamilton, JILA, University of Colorado
There’s a lot to like about the idea that there’s a connection between black holes and the birth of Universes, from both physical and mathematical points of view. It’s plausible that there’s a connection between the birth of our Universe and the creation of an extremely massive black hole from a Universe that existed before our own; it’s plausible that every black hole that’s been created in our Universe has given rise to a new Universe within it.
What’s missing, unfortunately, is the key step of a uniquely identifiable signature that could tell us whether this is the case or not. That’s one of the most difficult steps for any theoretical physicist: to determine the imprint of a new idea on our observable Universe, distinguishing that new idea from our old, prevailing ones. Until we successfully take that step, work will likely continue on these ideas, but they will only remain speculative hypotheses. We don’t know whether our Universe was birthed by the creation of a black hole, but at this point, it’s a tantalizing possibility that we would be foolish to rule out.
An earlier version of this article, previously published in January 2021, has been removed and replaced with this version by Dr. Ethan Siegel.