Injectable gel repairs severe spinal cord injuries and enables mice to walk
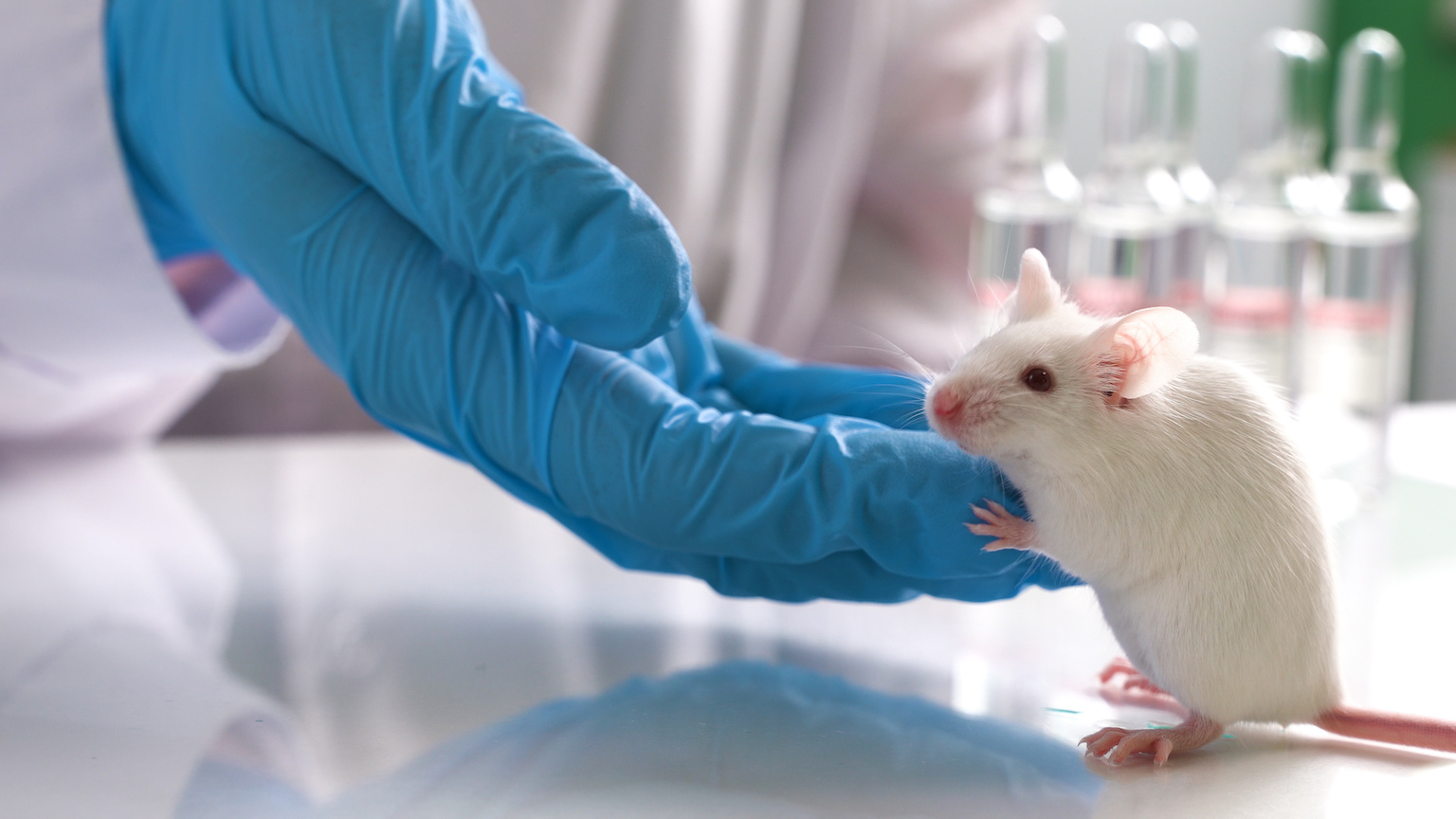
- Although drugs can stimulate neural regeneration, no therapeutics stimulate full recovery following a spinal cord injury.
- Cells at the site of spinal injury protect the neurons from further damage and promote healing, but they also form a scar that impedes neuron regeneration.
- An injectable gel that prevents scar formation and stimulates regeneration successfully repaired severe spinal cord injuries in mice.
The body is bad at repairing neural damage. This is bad news for the nearly one million people who will suffer from a spinal cord injury (SCI) this year. Surgical interventions and physical therapies improve recovery of motor skills following SCI, but a full recovery is rare. There are compounds that stimulate neural repair in a petri dish, but we don’t know how to administer those compounds to living organisms.
A group of researchers may have figured out how. Mice paralyzed by severe SCI regained the ability to walk three weeks after a single injection of their new therapeutic, according to a recent study published in Science. Their secret? Make the drug dance in Jell-O.
Glial scar: friend and foe
Surprisingly, the initial mechanical injury to the spinal cord is rarely the direct cause of paralysis. The initial event does kill a lot of cells and sever axons (the long, branch-like extensions that connect neurons to each other). However, the event rarely severs all the axons at the site of injury. If at least 5% of the original nerve connections remain, neurological function (e.g., for muscle control or sensory perception) is sustained. However, this initial event starts the cascade that results in paralysis.
As soon as the injury occurs, the clock starts. Within minutes, dying neurons leak their cellular contents into the local environment, triggering inflammation. Immune cells rush out of blood vessels and into the injury site. Their job is to clear out the damaged tissue, providing space for regeneration to occur. Unfortunately, this process also results in collateral damage to the few healthy neurons that remain. If the remaining axons are severed, the chance of recovery is all but lost.
Within hours, the cells of the nervous system become aware of the looming danger. Glial cells (nervous system cells that play a support role for neurons) release chemicals that restrict the spread of inflammation. To increase their regulatory power, the glial cells replicate. A lot. More glial cells means more power to control inflammation. Within days, there is an army of glial cells, densely packed on top of each other. The inflammation is controlled, but the cost is high. The dense cells form a literal barrier across the spinal cord: the glial scar. While the remaining healthy neurons are safe, regenerating the severed axons may take decades — if it ever occurs at all.
For decades, scientists considered removing or preventing the glial scar to be the key to recovering from spinal cord injury. But it is not as simple as cutting the scar out of the tissue. That would be like trying to cut a scar off your hand; it would just result in a bigger scar. In fact, studies have shown that surgically removing the scar results in less axon regeneration than not removing it. It turns out that, even though the scar acts as a barrier for regeneration, it also provides an environment that stabilizes communication between remaining neurons and stimulates axon regeneration (albeit at a very slow rate).
So, when a team of Northwestern University researchers set out to design a therapeutic for SCI, they knew it must prevent the formation of a dense barrier, while also mimicking the stabilizing, regenerative environment.
Jell-O and dancing growth signals
Cells are suspended in an environment called the extracellular matrix (ECM), kind of like how Earth is suspended in space. Like space, the ECM was once considered to be inert — just a place for cells to float. But space isn’t inert. It is a chaotic orchestra of activity: gravitational forces, radiation, and the occasional chunk of rock, all of which influence our planet. Similarly, the ECM also is not inert, and it influences how cells behave.
Key components of the ECM include long nanofibers composed of a collection of proteins that maintain a structural architecture (such as collagen, which is used to make the primary ingredient in Jell-O) and provide biological signals (such as growth signals that tell cells to replicate). The ECM is constantly being remodeled to support its resident cells. For example, during cellular regeneration, stem cells need continuous stimulation by growth signals. Local cells produce the growth signal molecules, and the ECM remodels itself to capture the molecules, keeping them right next to the stem cells instead of floating around aimlessly.
Over the past couple decades, an interesting compound has been shown to mimic the fibrous structure of the ECM. In 2008, Samuel Stupp, an expert in regenerative medicine, worked with a team of neurobiologists to show that growth signals could be embedded into this compound, and the resulting solution could restore partial motor function after a mild spinal cord injury in a mouse. But Stupp had an idea for how compounds could do more than restore partial function: make the growth signals dance.
“Receptors in neurons and other cells constantly move around,” Stupp said. “The key innovation in our research, which has never been done before, is to control the collective motion of more than 100,000 molecules within our nanofibers. By making the molecules move, ‘dance’ or even leap temporarily out of these structures, known as supramolecular polymers, they are able to connect more effectively with receptors.”
Supramolecular polymers are composed of individual molecules (called monomers) held together by reversible molecular interactions. Because these interactions are reversible, monomers are in constant motion, momentarily disassociating and re-associating with the collective nanofiber. Stupps hypothesized that increasing the rate of association would help growth signals interact with neural receptors more efficiently, thus improving neuron regeneration. Essentially, he wanted to make the drugs in his “Jell-O” dance to the same tune as neural receptors.
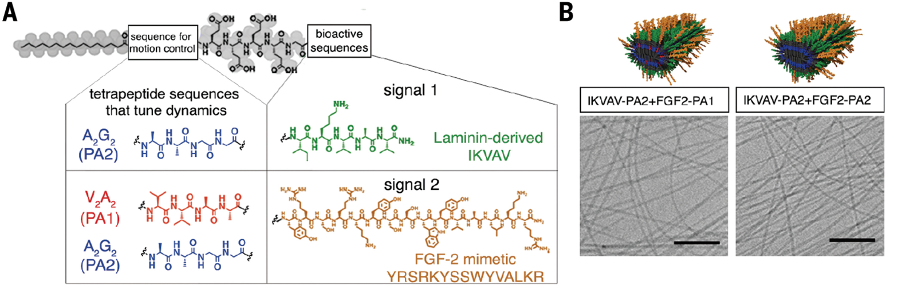
To achieve this, they created small mutations in the growth signal molecules. These mutations did not affect the molecules’ biological function, but it did make them dissociate and reassociate at higher rates. Then, they tested the new therapy: 24 hours after a severe spinal cord injury, mice were treated with the high-mobility supramolecular polymer solution, low-mobility supramolecular polymer solution, or saline (which served as a control). If their hypothesis was correct, mice treated with the high-mobility solution should recover the most.
Get up, pick up your mat, and walk
After three weeks, mice that received the high-mobility solution had 50% greater muscle control (i.e., the ability to walk) than the mice that received the low-mobility solution, and nearly 300% greater control than the mice that received saline. After 12 weeks, the mice injected with the high-mobility solution exhibited 50 times more axon regrowth than the control group. Additionally, the treatment blocked glial scar formation.
While the improvement in muscle control is impressive, it may not reveal the therapeutic’s full potential. These mice did not undergo physical therapy during their recovery, but a person would. During physical therapy, existing neurons are re-trained to walk. The more neural connections available, the easier it is to retrain.
While we do not yet know if these findings will translate to a human model, the researchers did observe that their high-mobility solution was also more effective at stimulating growth of human neural progenitor cells in the lab. “We are going straight to the FDA to start the process of getting this new therapy approved for use in human patients,” Stupp said.