Did it all start with a bang?
If we knew all we do about math and physics, but had never seen the heavens, what would we conclude?
“Both the solutions must be rejected, and as these are the only statical solutions of the equations… the true solution represented in nature must be a dynamical solution.” -Willem de Sitter
We take for granted how easily accessible our night sky is, even in our light-polluted modern world. The thousands of points of light, the plane of our Milky Way, the planets and Moon, and the diffuse nebulae all visible to the naked eye set our imaginations alight at once.
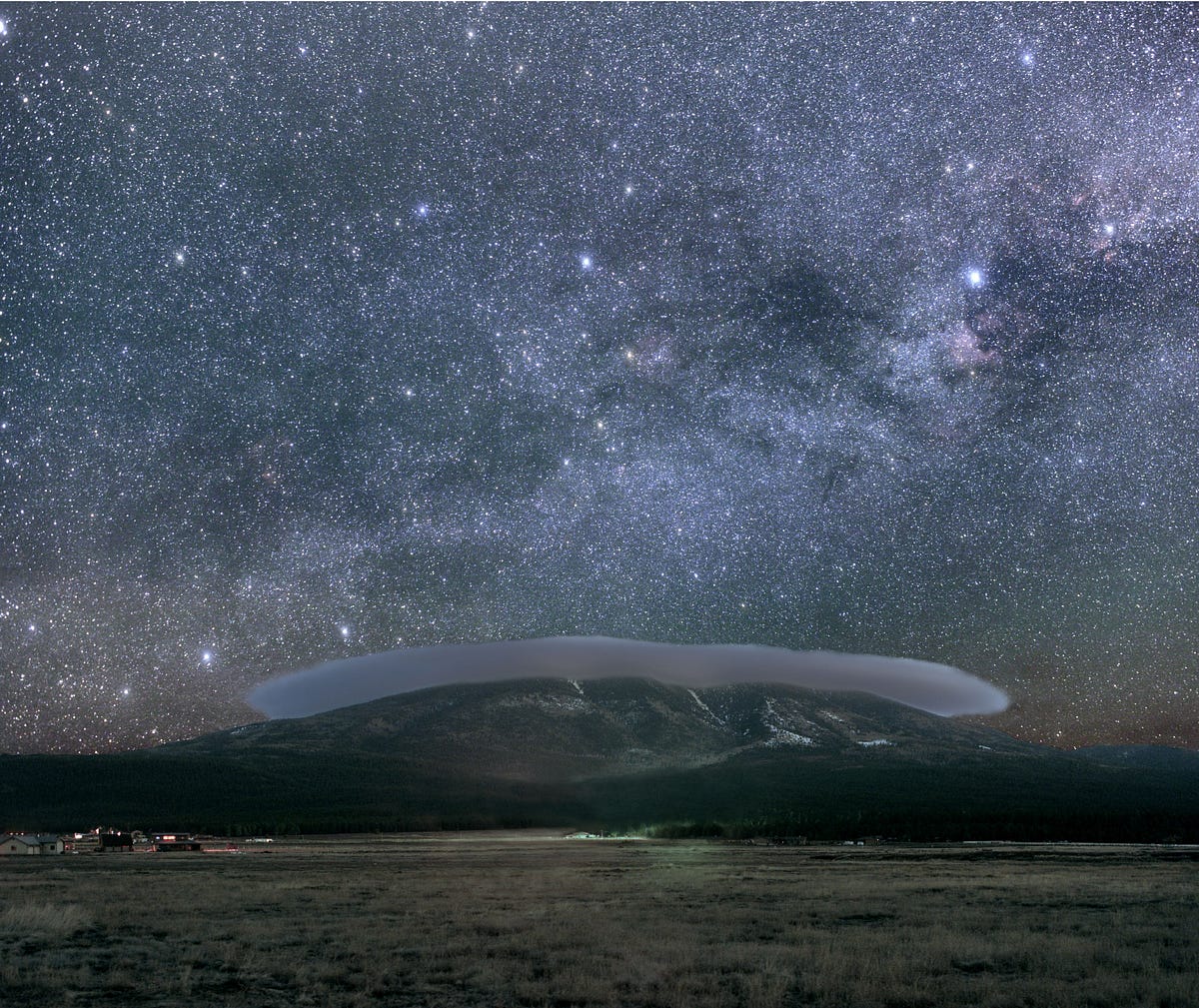
But not every world is like ours. Indeed, a great many worlds with considerable atmospheres are continuously covered in clouds, preventing anyone on the surface from seeing the Universe beyond, and preventing anyone from above seeing the surface. (At least, in visible light.) This is true of all the gas giants in our Solar System, of our rocky neighbor Venus, and even of Saturn’s giant moon, Titan.
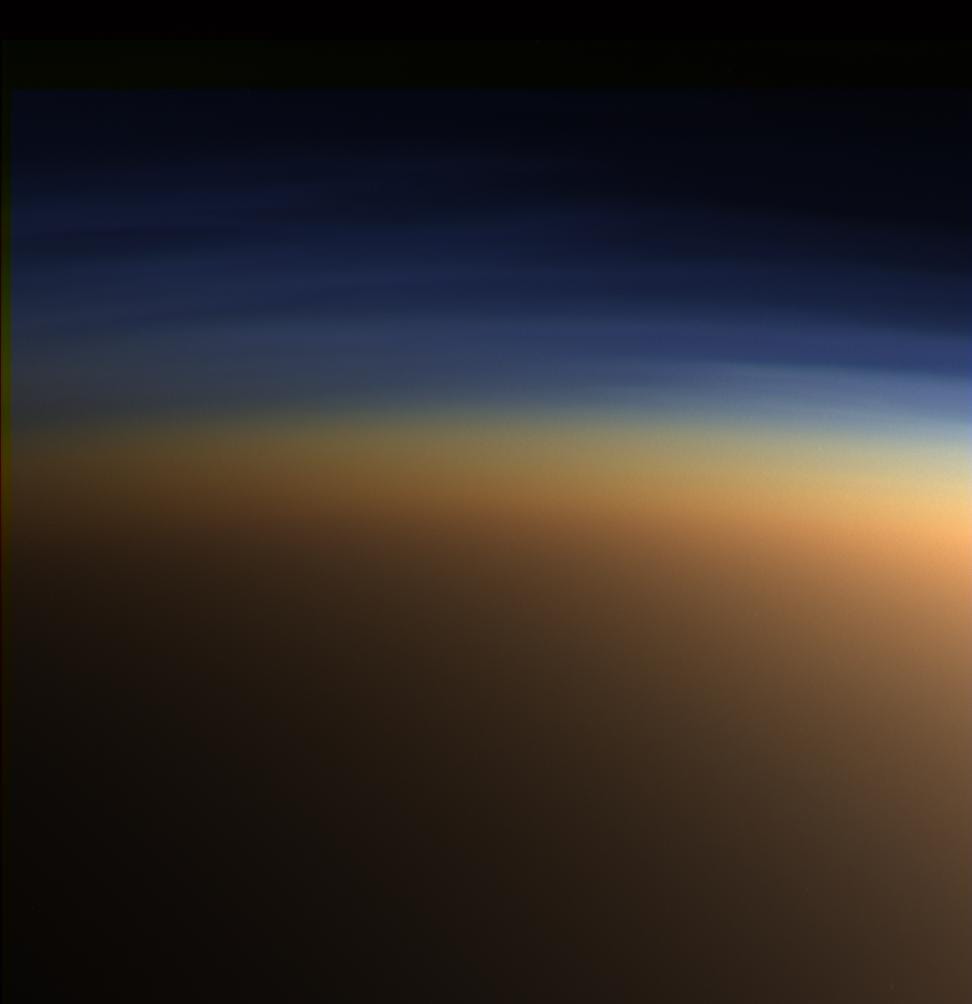
Well, what if that was our world? What if that was our sky? What if, instead of thousands of years of gazing up at the stars and the Universe beyond our planet, we had only ever had an opaque canopy above?
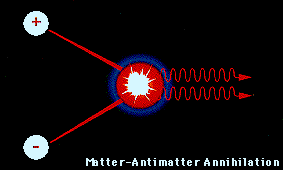
We’d still have all the terrestrial science we know and love, including all of particle physics. We could have discovered the rotation of the Earth from a Foucault pendulum, and we could have discovered General Relativity from experiments involving the red/blueshifting of light in our planet’s gravitational field.
But without a view of the heavens, we wouldn’t have any way to know about the Universe. Yes, day-and-night, as well as the seasons, would provide a clue as to the existence of the Sun. Terrestrial measurements could show the Earth’s shape, size and axial tilt. And tides, eclipses, and luminance changes in the night sky could all provide evidence for the Moon as well. But what if we were able to look at the Universe beyond our planet for the very first time tonight, but with the full complement of modern technology?
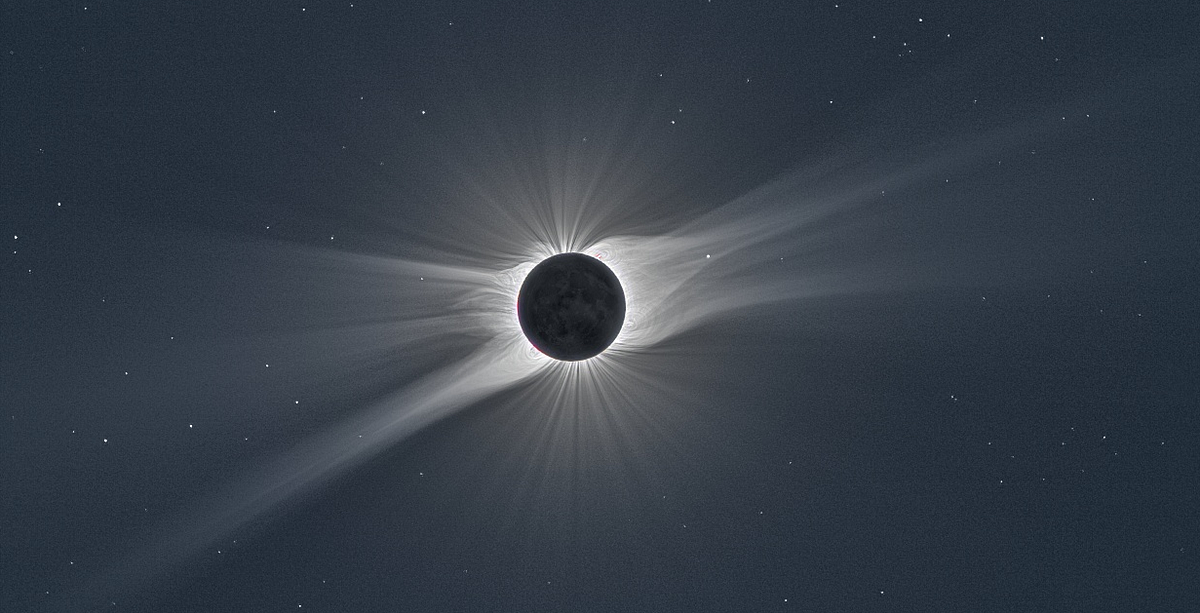
Yes, you’d notice the stars right away, and if you looked in the direction of the Sun, you’d notice that the stars nearest it had their positions shift as the Sun moved relative to them, confirming General Relativity as our theory of gravity. Each one of these stars — as well as our Sun — would show a characteristic spectrum, a set of absorption lines, laid over a broad blackbody spectrum representative of that star’s temperature. The spectrum of lines would allow us to learn immediately what these stars were made up of.
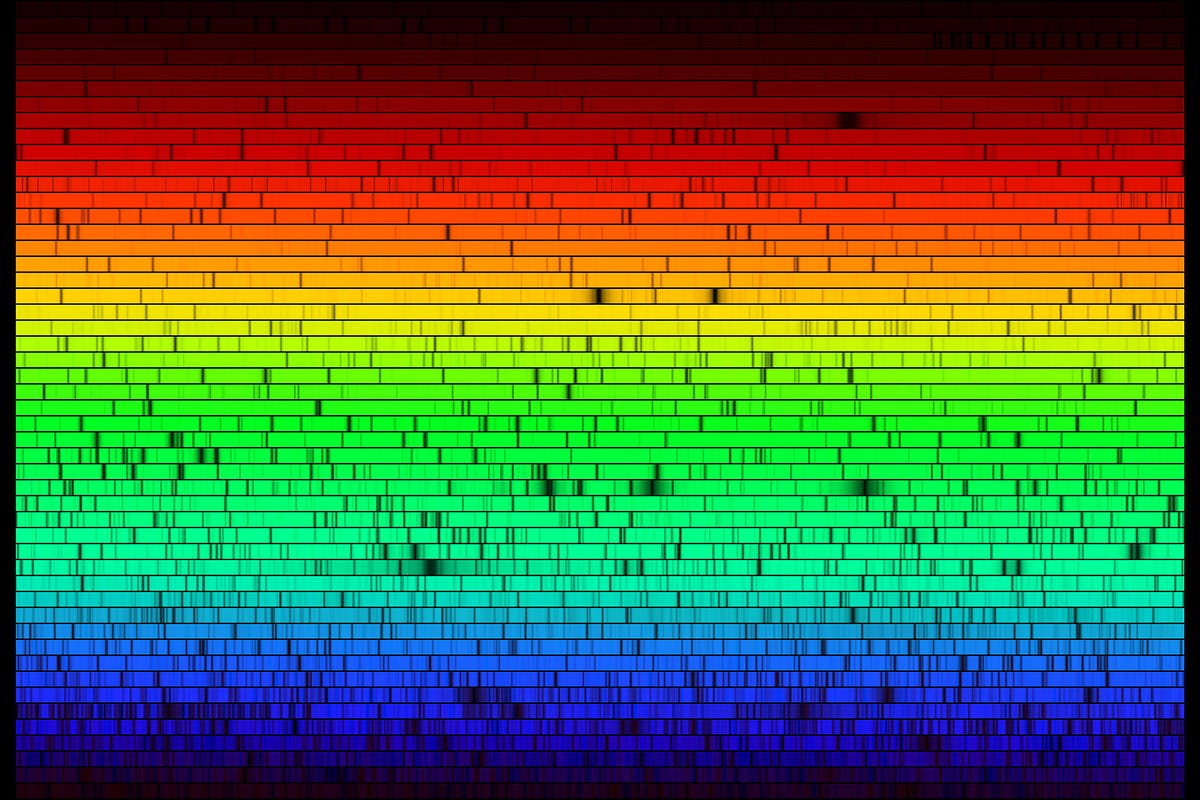
All of these lines correspond to atoms in different states, and the thickest of these lines correspond to the elements hydrogen and helium, which are present in far greater abundance than all other elements combined. If we were very subtle in our measurements (and we are), we’d notice that some of these stars had their spectral lines all shifted towards the red, while others had them shifted towards the blue. This is due to their motion: objects moving away from us have their light shifted towards the red and objects moving towards us have it shifted towards the blue. This happens in the same way a siren moving towards you sounds higher-pitched and one moving away sounds lower-pitched, via the Doppler Effect!
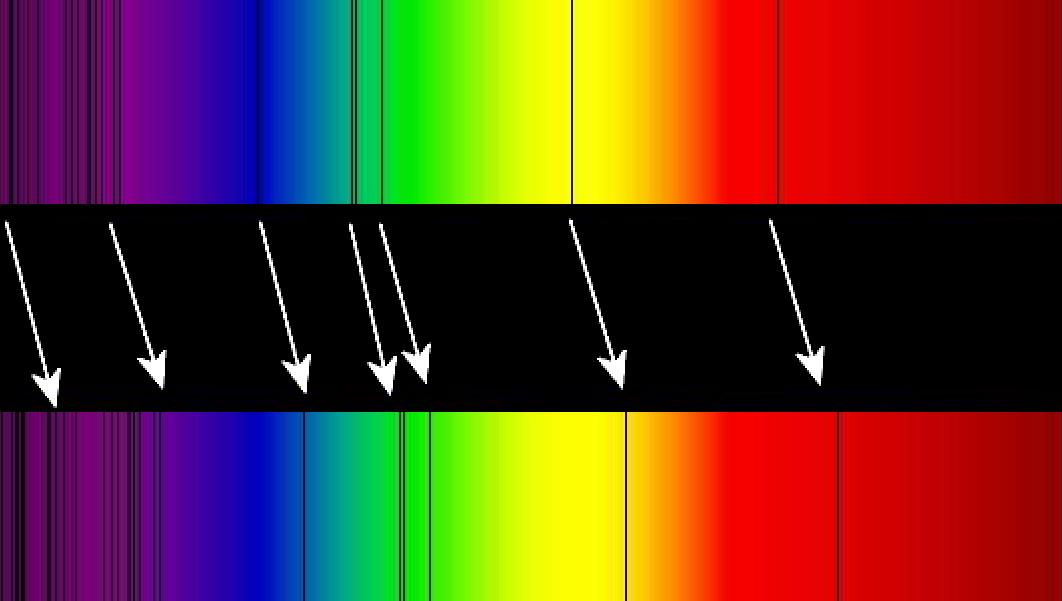
We’d also discover a number of variable stars, or stars whose intrinsic brightness varied over the timescales of hours, days, or many months. There would be many different classes of variable stars, with different luminosities (or intrinsic brightnesses) and different periods-of-variation.
But the brightest among these, the Cepheids, would exhibit a fantastic correlation that could have been discovered in mere weeks: the brighter they are intrinsically, the longer it takes for them to pulse!
This is tremendous, because it means if you measure how long it takes one of these stars to undergo a variation, you know how intrinsically bright it is.
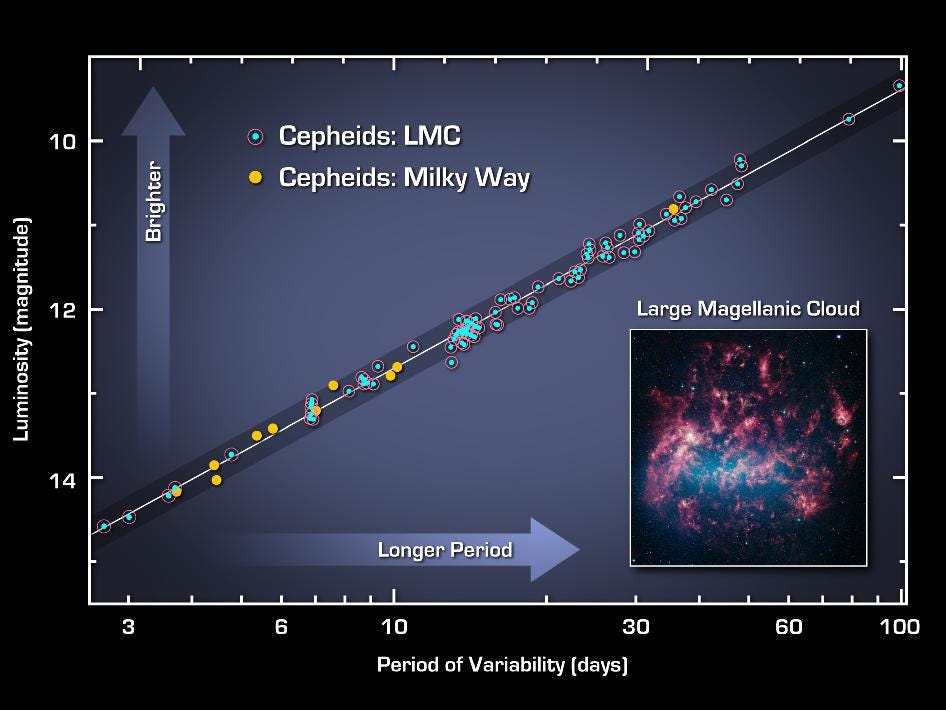
And therefore, once you measure a variable star’s apparent brightness, you can immediately know how far away it actually is. But stars in our own galaxy aren’t the only thing you’d see.
You’d also notice, among the stars, many nebulous object that would come into focus with the power of a telescope: spiral and elliptical extended objects. Some of them would be found in relative isolation, while others would be found clumped together. Some would appear quite large and bright, while others would appear tiny. And even though their shapes would be apparent with modern technology, their nature wouldn’t be so immediately clear.
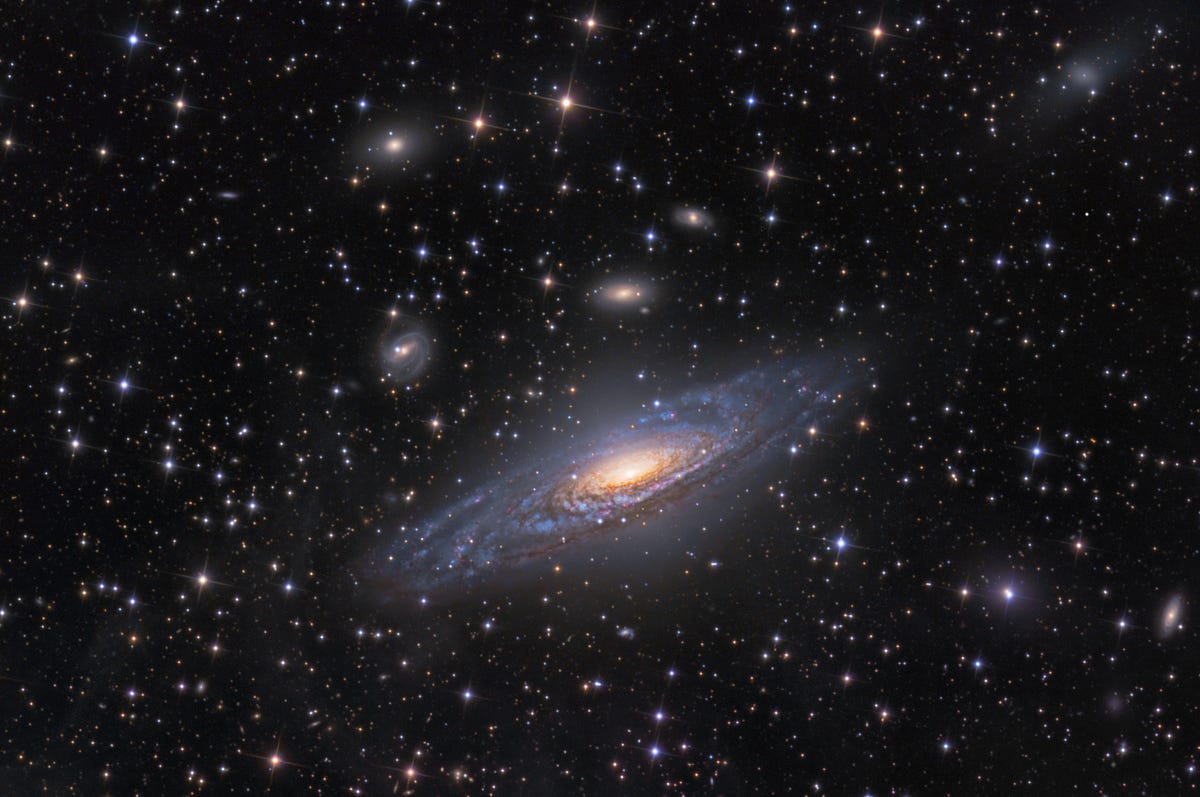
Unless, of course, we looked for two things that are present in all of the ones that are the brightest to our eyes and tools:
- Spectral lines, which would allow us to measure how quickly each object is either moving towards or away from us, and
- Variable stars, which would allow us to know — by measuring their periods and apparent brightness — how far away each object actually is.
And based on those two things alone (although we could use other methods, as well), this is what we’d find.
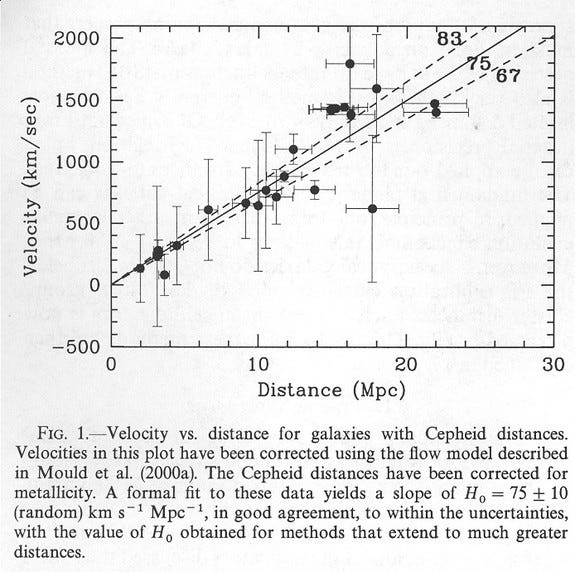
First off, we’d find that these objects were individual galaxies not so different from our own Milky Way, with billions or even trillions of stars comprising each one. And second, we’d find that the farther away the galaxies are, on average, the faster they appear to be receding from us!
There are a number of possible explanations for this, including that:
- Light gets tired, and loses energy the farther away an object is,
- The Universe began from an explosion with us almost perfectly at the center, and the galaxies moving away faster received more energy from the explosion,
- The Universe is truly static, but some fundamental constants are changing in concert with one another to make it look like these galaxies are receding,
- The Universe goes through oscillatory phases of expansion and contraction, and where we are is going through an expansion phase right now, or
- The very fabric of the Universe is expanding, and these galaxies are redshifted because of the properties of expanding space.
Some of these options are compatible with General Relativity and others aren’t, but the last one is particularly interesting, because — if it were true — would allow for a couple of new predictions that could be tested immediately.
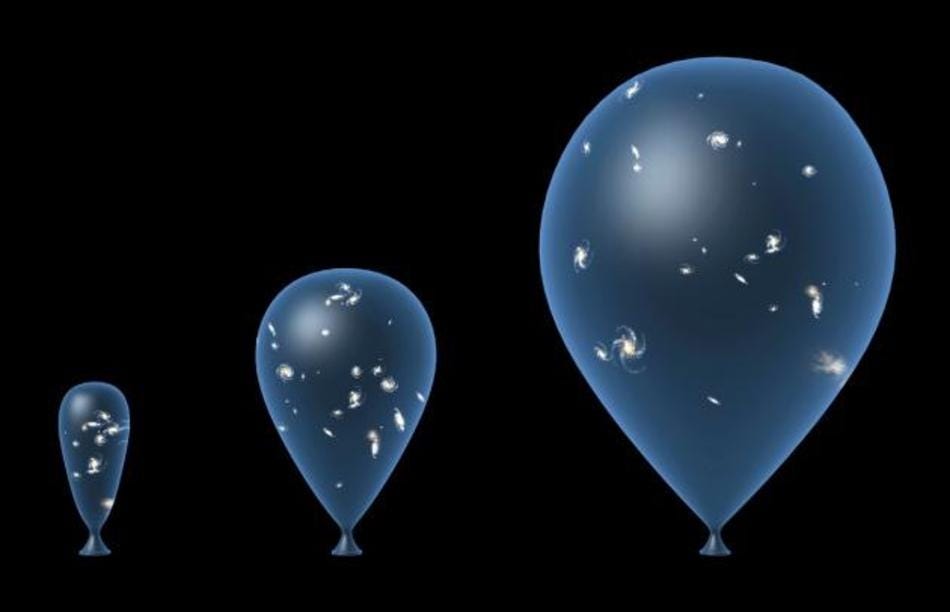
In an expanding Universe, things would have been closer together and denser in the past. Because of how radiation works, the radiation in the Universe should also be hotter in the past. Because of how gravitation works, matter should have been more uniform in the past, and clumpier now.
For that last part, we can point our telescopes at the distant Universe as well as the nearby Universe, and see how the clustering of galaxies changes, and whether it’s in line with this picture or not.

And our observations are aligned with what you’d theoretically predict, given this scenario. But there are other predictions that come about, too, thanks to the fact that the Universe was hotter, more uniform, and denser in the past. We should find, going back, that there’s an era where things were so uniform that there weren’t enough stars to ionize all of the neutral atoms in the Universe, making it transparent to visible light. So there should be some distance beyond which we cannot see in every wavelength, because there’s neutral dust blocking that light.
This prediction — of a Gunn-Petersen trough — means that distant objects should have their emission spectra cut off above a certain wavelength the farther away an object is.
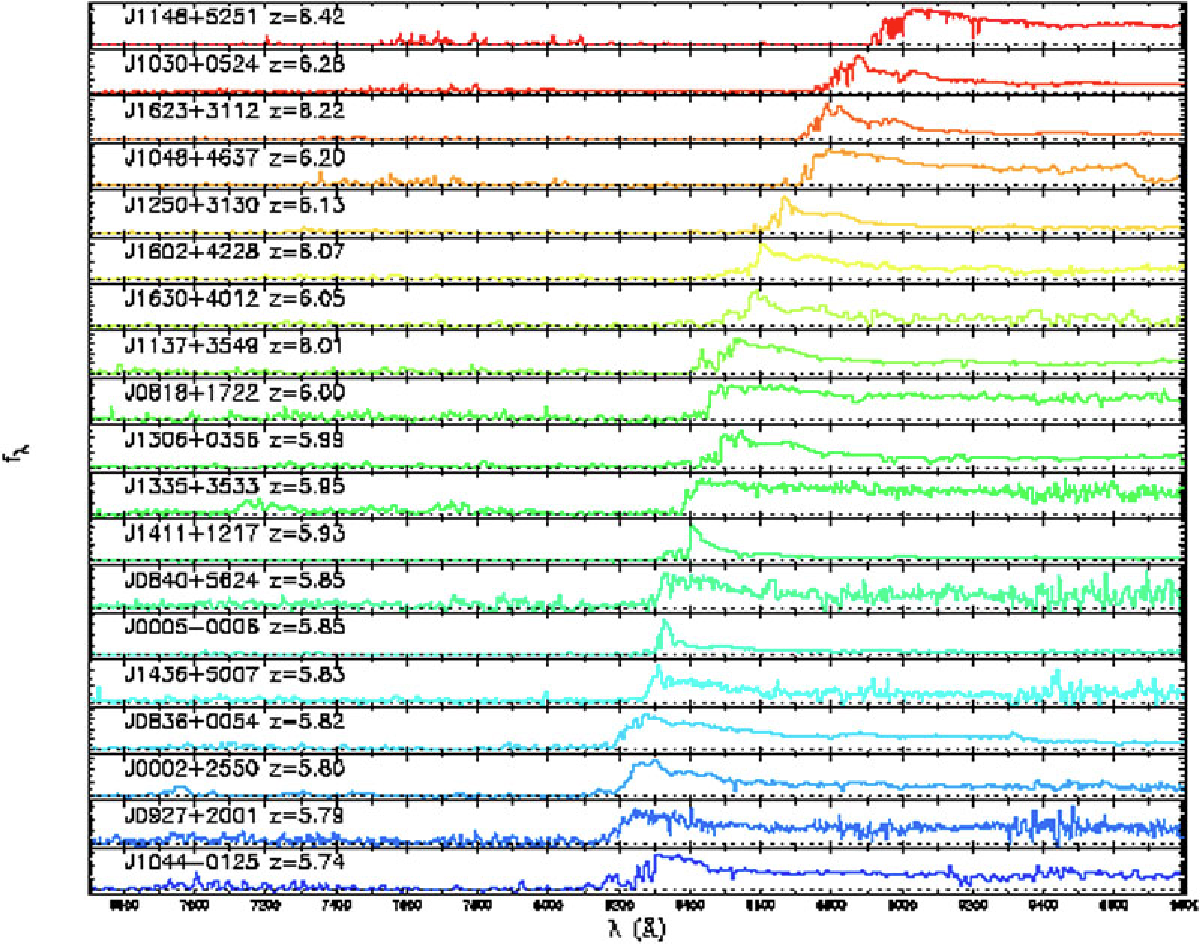
And we see it, above, right around a redshift of z=6, meaning all the spectral lines are shifted by a factor of 7 from their rest frame.
But if this is how the Universe works, we should be able to go even farther back, to a time before the first stars formed. Eventually, the Universe must have been so hot and so dense that not even neutral atoms could form! The reason for this is the same reason that if you continue to heat solid matter, it melts into a liquid, the liquid boils into a gas, and the gas then ionizes into a plasma: too much energy prevents something from stably binding together!
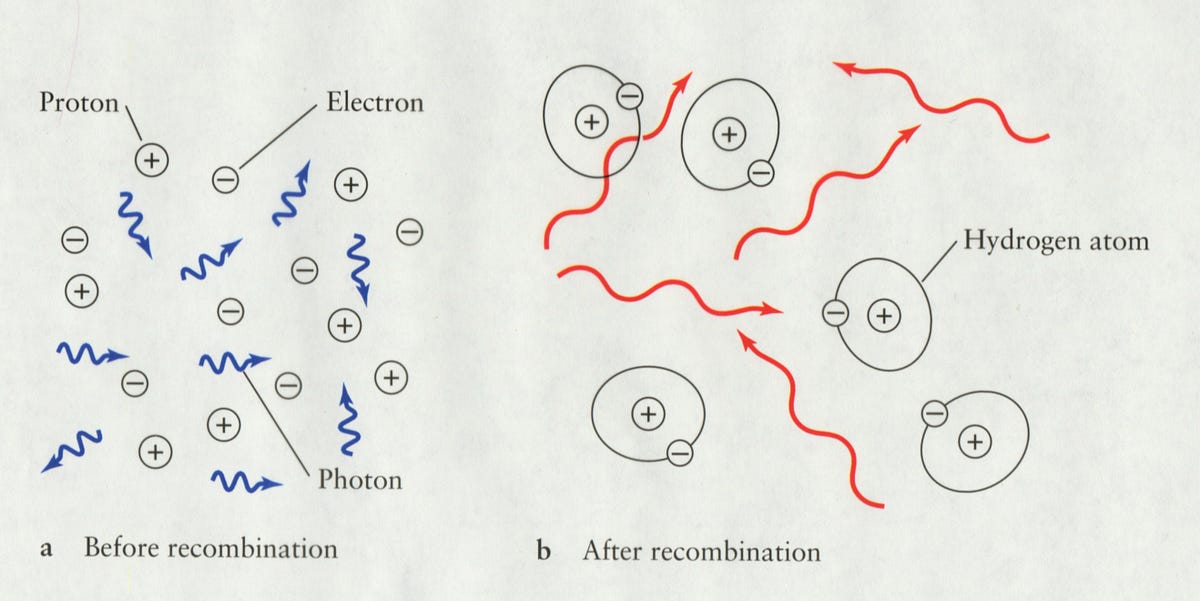
When this transition occurs — when the Universe cools enough to form neutral atoms — the radiation that was around at that time should finally be free to stream unimpeded through the Universe. Because of how expansion works in General Relativity, that leftover radiation from the primeval fireball should still be around today, although it should be redshifted to just a few degrees above absolute zero.
It should be almost exactly the same in all directions, and it should have the spectrum of a perfect blackbody. If we look for it, guess what we find?
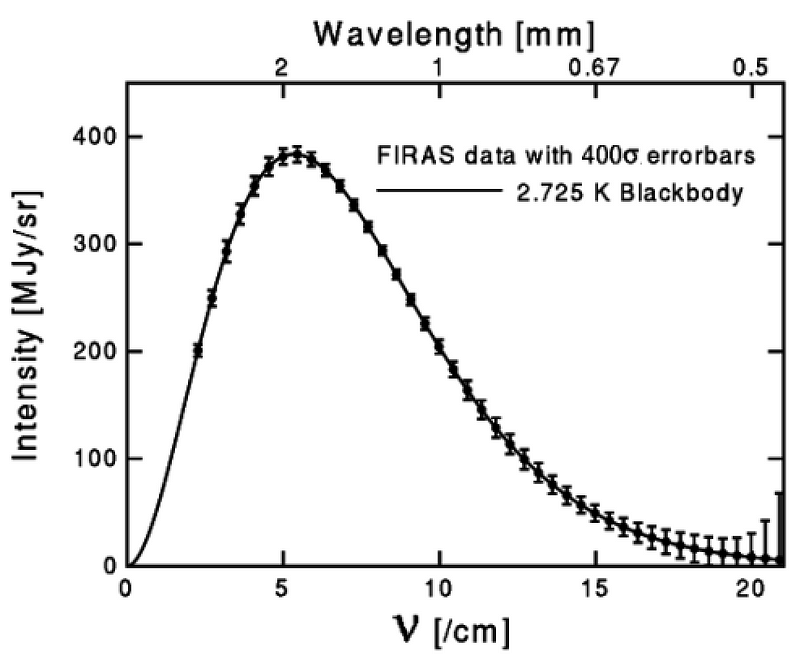
It fits perfectly. And finally, there’s one more prediction, going back even farther. If the Universe could have been hot-and-dense enough to prevent the formation of neutral atoms, then it must have been hot enough even earlier to blast apart any heavy atomic nuclei that formed!
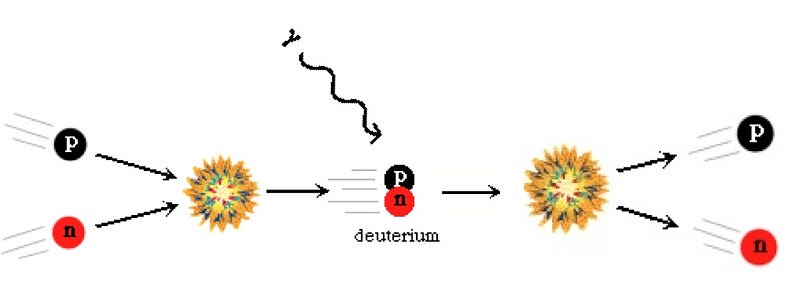
So if this were the case, we would expect that there were only free protons and neutrons for the first few minutes, unable to stably form anything heavier. When the Universe finally cooled below a critical temperature, they could fuse to form deuterium, helium and a little bit of Lithium, and we should be able to measure the pristine gas left over from this event today.
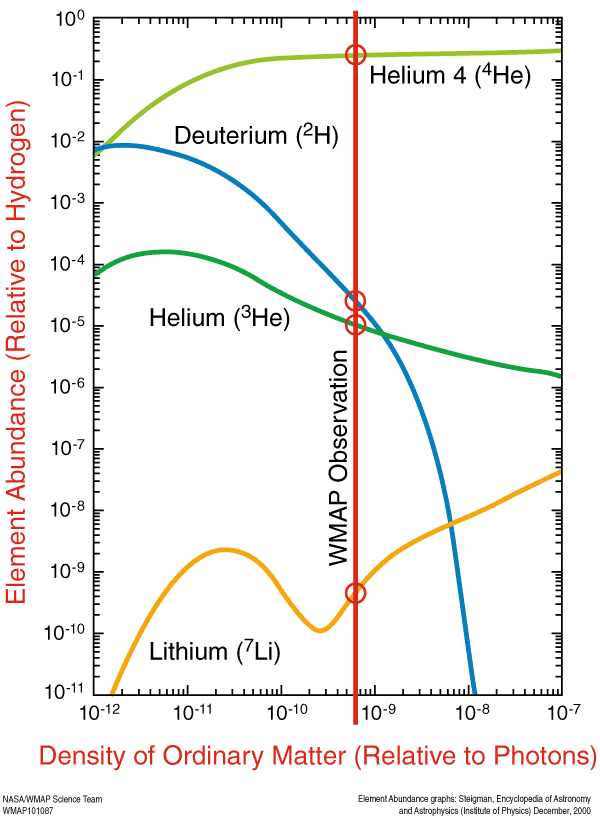
And just as you’d hope, our predictions and our observations match. In fact we have detected this pristine gas, billions of light years away, and it contains (and doesn’t contain) exactly the elements we expect. (Although, to be fair, it would probably take many years to find it if we just started looking today.)
There are plenty of other predictions of this scenario that have been supported by observation, but these pieces of evidence are more than sufficient. You see, this is what the Big Bang is! It’s this set of initial conditions — a hot, dense, uniform, matter-and-radiation-filled Universe — that expands and cools with General Relativity as our theory of gravity, that gives rise to all of these phenomena and more, including the sophisticated structure we see today.
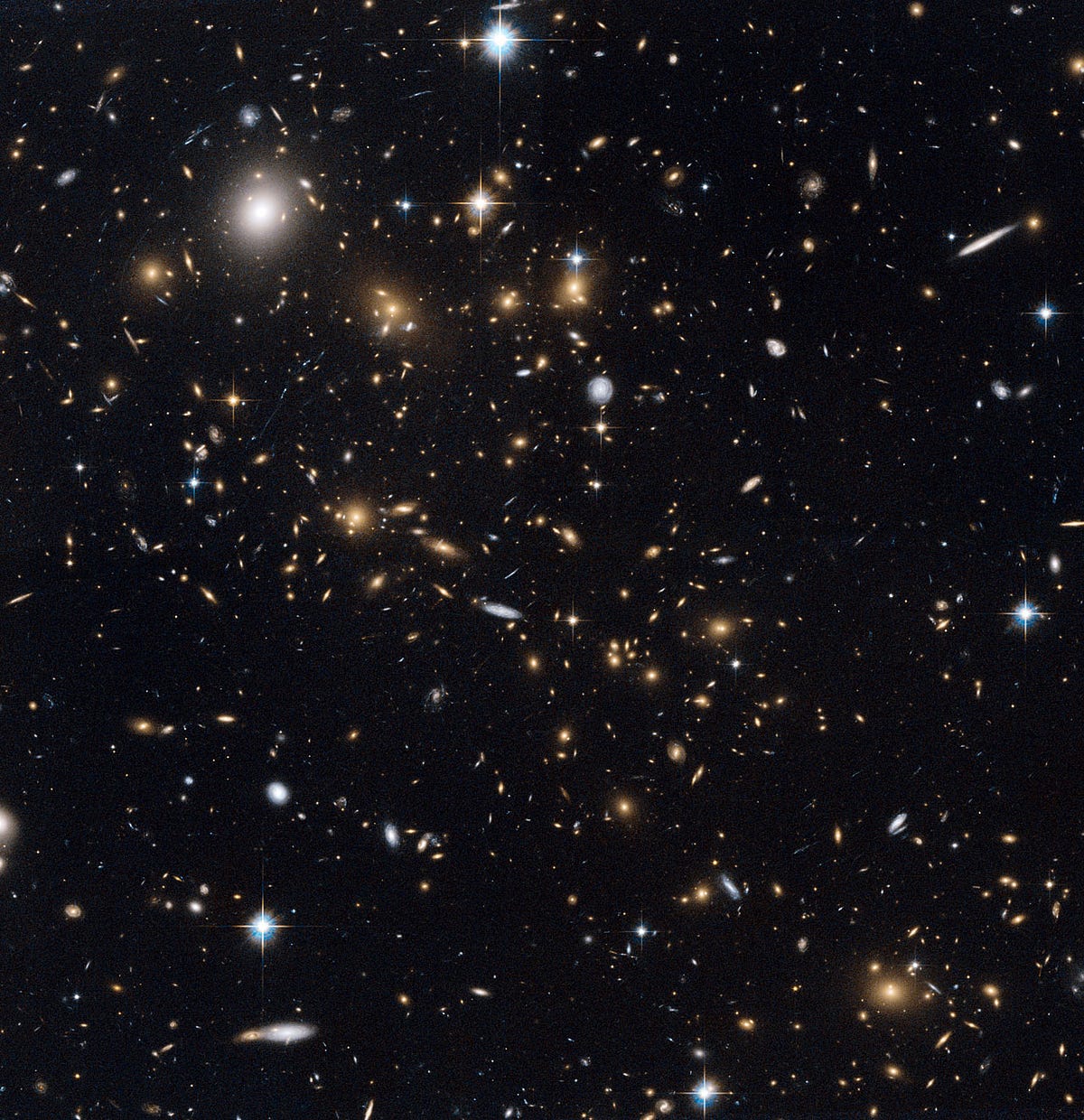
To explain all of the details, such as the patterns of clustering, the fluctuations in the microwave background, gravitational lensing and the way the expansion rate evolves over time, you need some additional things as well: dark matter, dark energy and cosmic inflation. That’s okay, because the Big Bang isn’t the end of knowledge, it’s simply the only self-consistent, predictive framework that’s consistent with all of these observations!
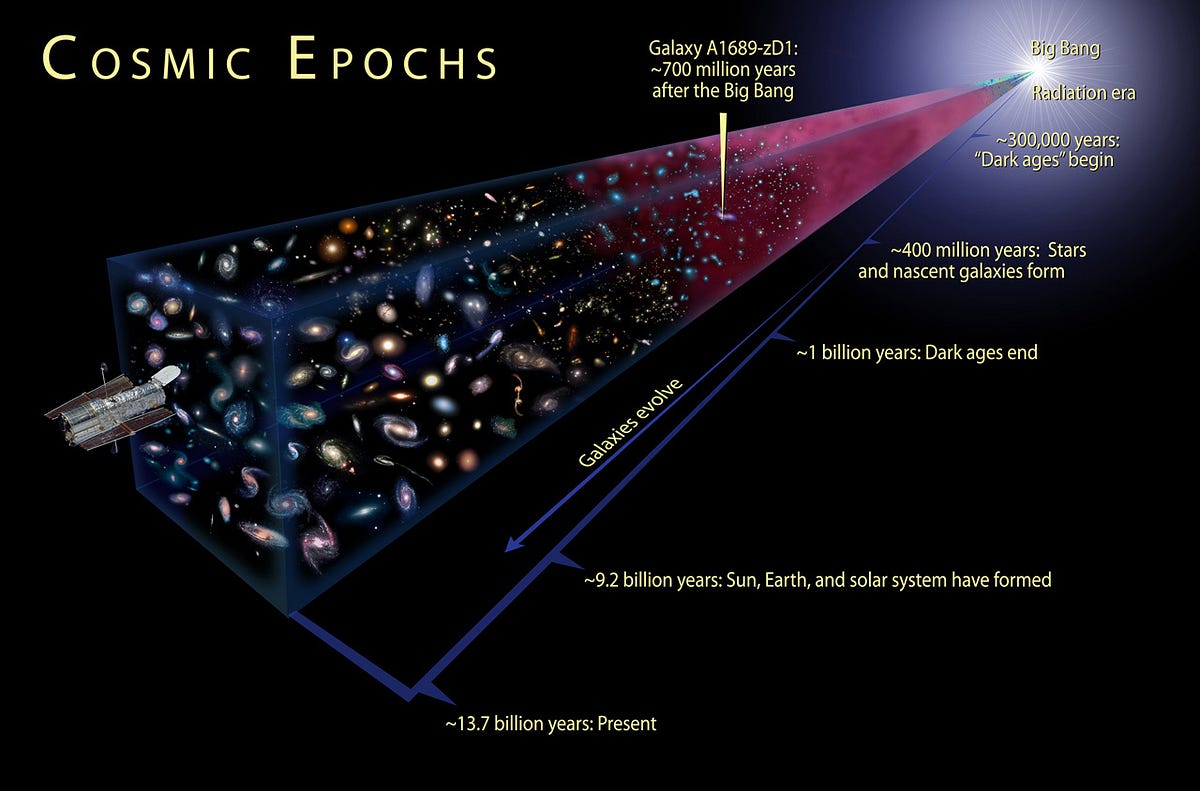
Even if we had never seen the heavens before, and we looked up for the very first time tonight, we’d be able to deduce all of this in mere weeks, if we knew where to look and we had the right tools at our disposal. To the best of our knowledge, yes, it all started with a bang, and now you know why that’s the best conclusion the Universe has to offer about its origins!
Want to participate in a long-form conversation about this post? Head on over to the Starts With A Bang forum for this post at Scienceblogs!