Relativity and the physics of immortality
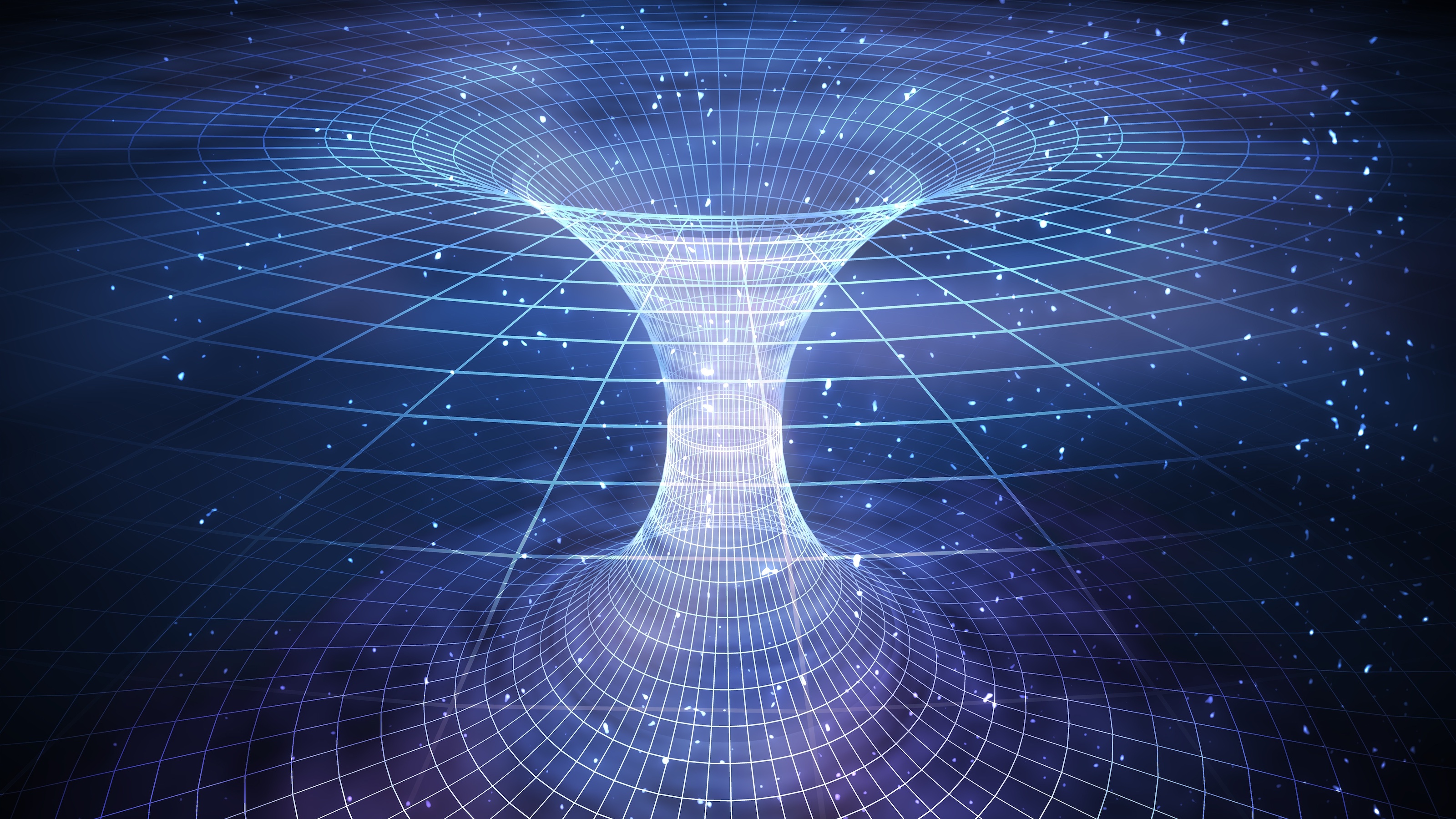
- No matter who, what, or where you are, or how fast you travel, time will always travel at the same rate for you, the observer: at the rate of one second per second, always, and under all circumstances.
- However, by boosting yourself to near-light speeds, time will pass for the rest of the Universe more quickly than it will for you, enabling you to observe all of cosmic history unfold before you die.
- By leveraging a few tricks, like becoming a photon or experiencing gravitational time dilation, it may seem possible to endure permanently, but that’s only a trick of perspective. In the end, all will succumb to time’s inevitable passage.
From your own experiential perspective, the laws of physics are stacked against you if you ever hope to achieve immortality. From a thermodynamic perspective, every system tends toward increasing entropy-and-disorder, and the only way you can combat that is by constantly inputting an external source of energy; in other words, your body and mind will eventually break down. And although you might try to leverage the power of relativity to dilate time and slow its passage, that will never work from your individual perspective; time only dilates or slows relative to an observer in a different reference frame from your own.
While this may confine a human’s dream of immortality to solutions that rely on technological enhancements or science-fiction level technology that relies on novel physical laws and/or phenomena, there’s still plenty for relativity to say about living forever: at least, relative to the rest of the Universe. While nearly all of us living today will certainly be dead in another century, should we all remain on Earth, the lessons from both special and general relativity teach us that there are a few physical situations that we should strive for if we truly want to maximize the amount of time that we can spend as living creatures within our Universe. Here’s the key insight we all need to understand.
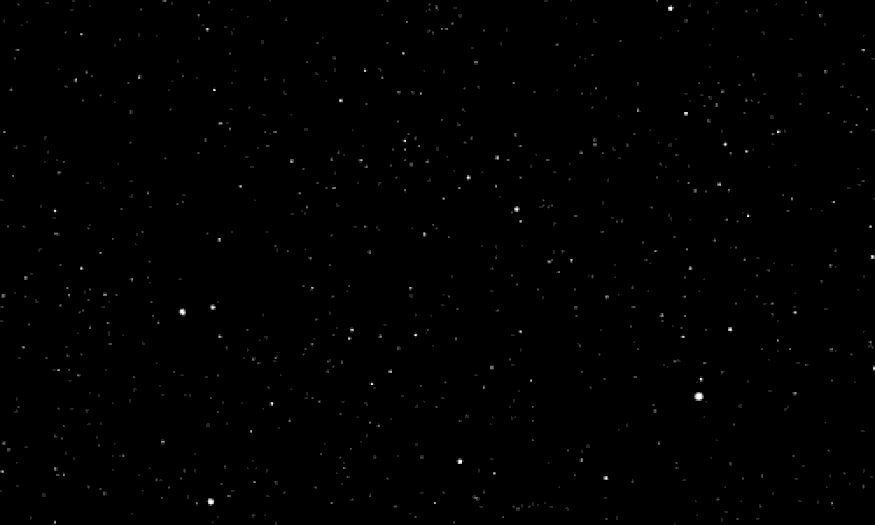
The foundation of relativity: spacetime
Even though we normally credit Einstein with overcoming the disparate ideas of space and time that had held sway since the time of Newton and coming up with the revolutionary concept of a four-dimensional fabric that weaves them both together — spacetime — it wasn’t Einstein at all that came up with that key insight. It’s true that 1905 was indeed a banner year for Einstein, with the two key insights that powered special relativity key among them:
- That the laws of physics are invariant, or that they do not change, in all non-accelerating frames of reference.
- And that the speed of light in a vacuum, c, is identical for all observers, regardless of their motion or of the motion of the light source in question.
While these insights were enough for Einstein to build out the framework that contained special relativity, including the phenomena of length contraction and time dilation experienced by different observers and the relativity of the notion of “simultaneous,” it didn’t necessarily put space and time on the same footing as one another. The person who did that, perhaps ironically, was Einstein’s former professor Hermann Minkowski, who built upon his former student’s work by weaving space and time together into a single four-dimensional entity: spacetime.
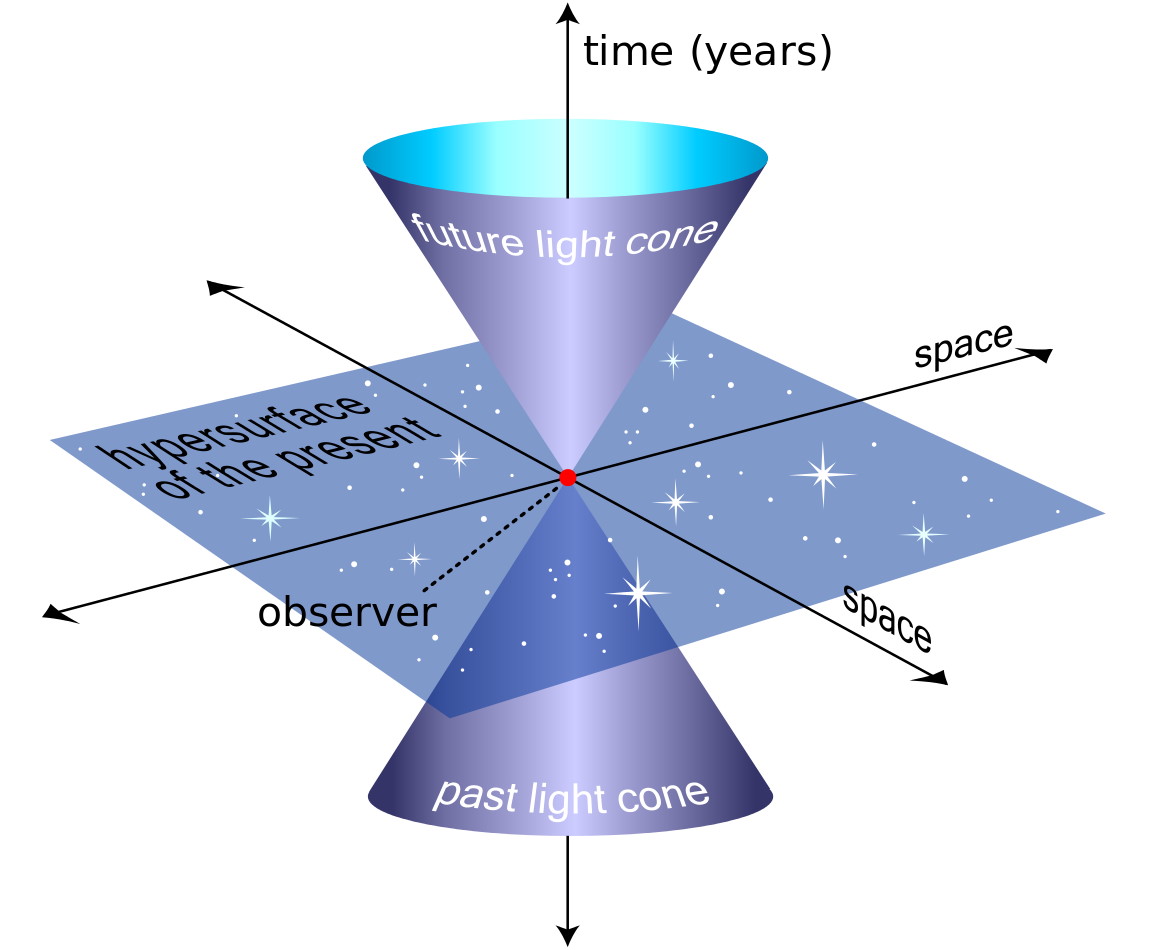
Minkowski’s famous quote, delivered in a lecture occurring less than a year before his untimely demise at the young age of 44 due to an acute case of appendicitis, reads as follows:
“The views of space and time which I wish to lay before you have sprung from the soil of experimental physics, and therein lies their strength. They are radical. Henceforth space by itself, and time by itself, are doomed to fade away into mere shadows, and only a kind of union of the two will preserve an independent reality.”
Minkowski’s spectacular realization was that while neither time nor space were invariant (i.e., did not change) under relativistic transformations, there was a quantity that did remain invariant: the spacetime interval, or as Minkowski called it, “the Einstein interval.” It shows that while your motion through space and time, individually, can take on any value ranging from no motion at all through motion up to the speed of light, the difference between your motion through time (squared) and your motion through space (squared) will always remain the same. That key realization led to the formulation of spacetime as the key important physical quantity to consider, and it would remain so even years later: when gravity entered the picture.
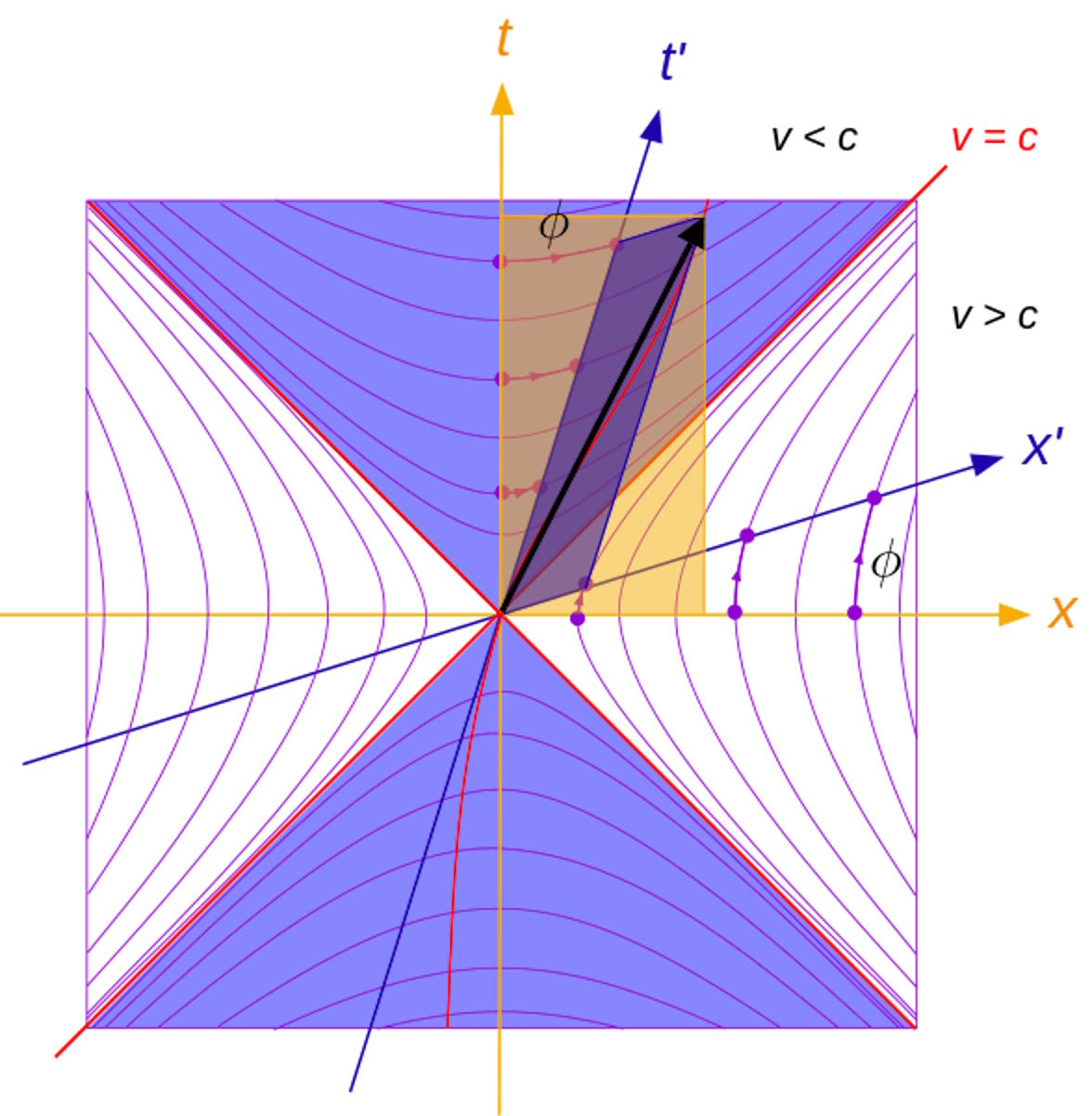
Time in flat, and curved, spacetime
Special relativity taught us something profound about time: that relative to some observer who remains at rest, someone who gets in a rocket ship and travels close to the speed of light will, upon returning to the observer who began and remained at rest, discover that they’ve both:
- traveled a much greater distance through space,
- and also traveled a much smaller amount through time.
This is consistent with everything that Einstein (and Minkowski) had to teach us, and is illustrated most famously by what’s known as the twin paradox, where the twin who goes close to the speed of light (and changes their reference frame) experiences the passage of time more slowly than the twin who remained at home.
But when relativity wasn’t considered in merely the special case of a flat, empty Universe but rather in the more realistic case of a Universe filled with matter and energy, including massive sources of matter that clumped together, spacetime would need to be generalized. Rather than the simplistic, flat spacetime that Minkowski proposed, an entirely new theory would need to be created:
- one where space and time were still woven together into a fabric that still contained a similar invariant interval,
- but where spacetime itself was allowed to be curved (and to evolve) owing to the presence and distribution of all the matter and energy within it.

Again, the more quickly you move through space, the less rapidly you experience the passage of time relative to someone who remains at rest, but this time there’s a twist. It’s as though the more severely the space that you occupy is curved, the more severely the passage of time gets curved as well, in exactly that same sort of “one increases, the other decreases” fashion. This is why time passes at different rates dependent on your elevation, and why your head (which is farther from the Earth’s center, and in a region of slightly less spacetime curvature) ages faster as compared with your feet.
The Parker Solar Probe, which comes closer to the largest mass in our Solar System (the Sun) than any other object, is presently the most asynchronous object with respect to Earth as far as gravitational time dilation is concerned. But the lessons from the generalized version of special relativity — general relativity — which includes gravitation, goes well beyond our Solar System. It teaches us that the denser an object is, and the closer you get to it, the more and more severe the curvature of space and time are. Under the most extreme scenario, right outside the event horizon of a black hole, practically no time at all will pass for you, while the rest of the external Universe continues to age as normal.
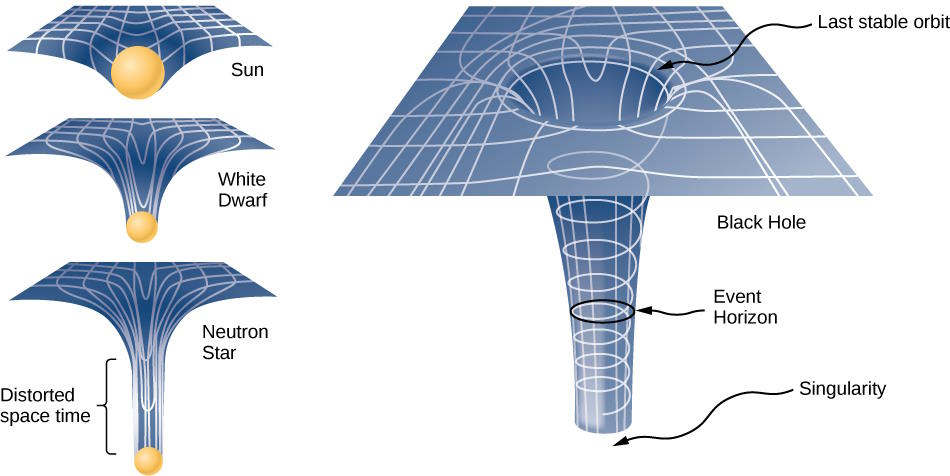
The physics of immortality
This sets up two different realistic routes to experience the far future of the Universe, as far as the passage of cosmic time is concerned, within a single, normal, non-augmented human lifetime.
- You can attempt to reach as close as possible to the speed of light, with the understanding that the closer you go to that vaunted speed limit, c, the greater the difference will be between how you experience time and how an observer who remains at rest experiences time.
- You can attempt to dive as deep as possible into a gravitational field, where the spacetime curvature is strongest, without crossing over the “point-of-no-return” (i.e., the event horizon), and the longer you remain there, the greater the difference will be between how you experience time and how someone far outside of the gravitational influence you’re succumbing to will experience time.
The first one relies on special relativity alone, and can be illustrated in a remarkably simple fashion: by imagining that you get into a rocket ship that’s capable of accelerating continuously at what we call “1 g” or at the acceleration provided by gravity at Earth’s surface: 9.8 m/s². As your speed ramps up, you’ll find that time passes at nearly the same rate for you as it does for any external observer, and that you approach, but never quite reach, the speed of light.
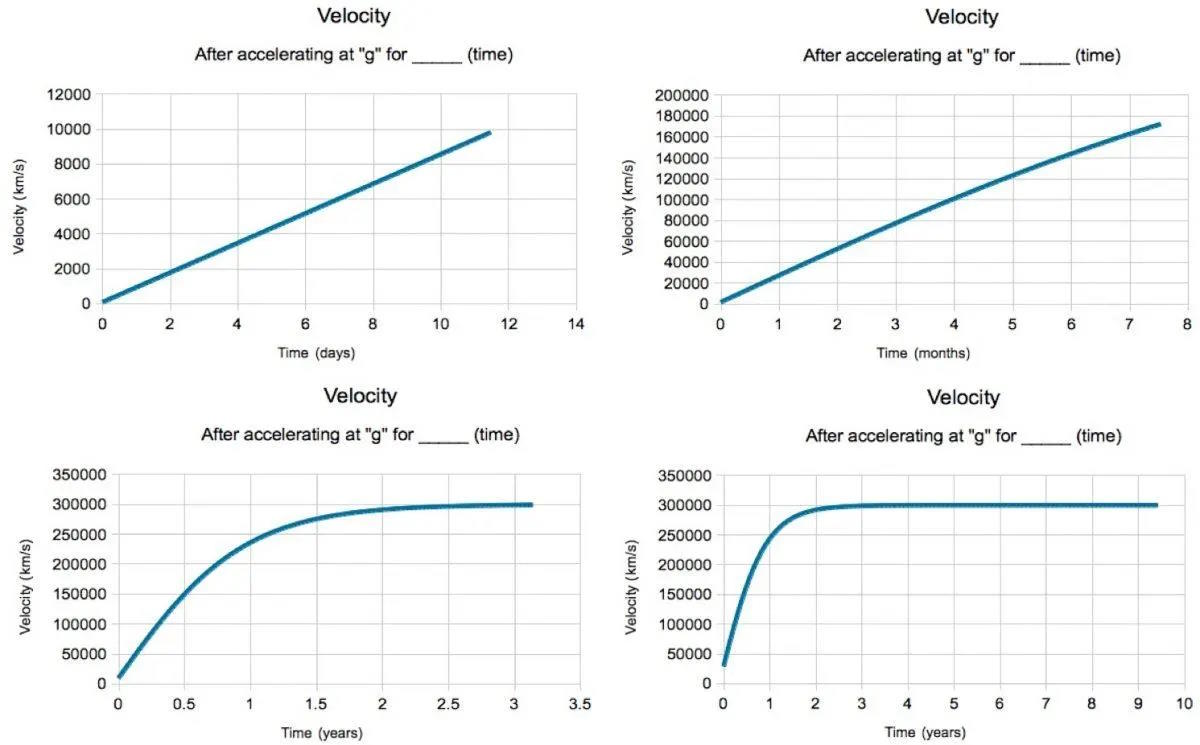
But as you get closer and closer to the speed of light — and as relativistic effects start to dominate over the conventional Newtonian ones — the entire cosmic future begins to pass you by. After about 10 years of accelerating at 1 g, you’ll find that you’re moving incredibly close to the speed of light relative to your surroundings: traveling at 299,792,457 m/s, or just 1 m/s shy of the speed of light. Your rocket ship will have already traveled more than 10 light-years (but less than 15), but someone back on Earth will have experienced more than 20 years of time passing. And this difference only gets more severe as you continue accelerating, especially at high speeds.
After 20 years in your ship, you’ll have traveled more than 100 light-years (because lengths contract), while someone on Earth will have aged hundreds of years (because time dilates).
After 30 years, you’ll have traveled thousands of light-years, and someone back on Earth will have aged nearly 10,000 years.
After 50 years, you’ll have traveled hundreds of thousands of light-years, and someone back on Earth will have aged millions of years.
And after 100 years, assuming you live that long (hey, it’s possible!), you’ll have traveled hundreds of billions of light-years (larger than the observable Universe), while hundreds of billions or even trillions of years (longer than the present age of the Universe) have passed for an observer back on (the now-destroyed) Earth.
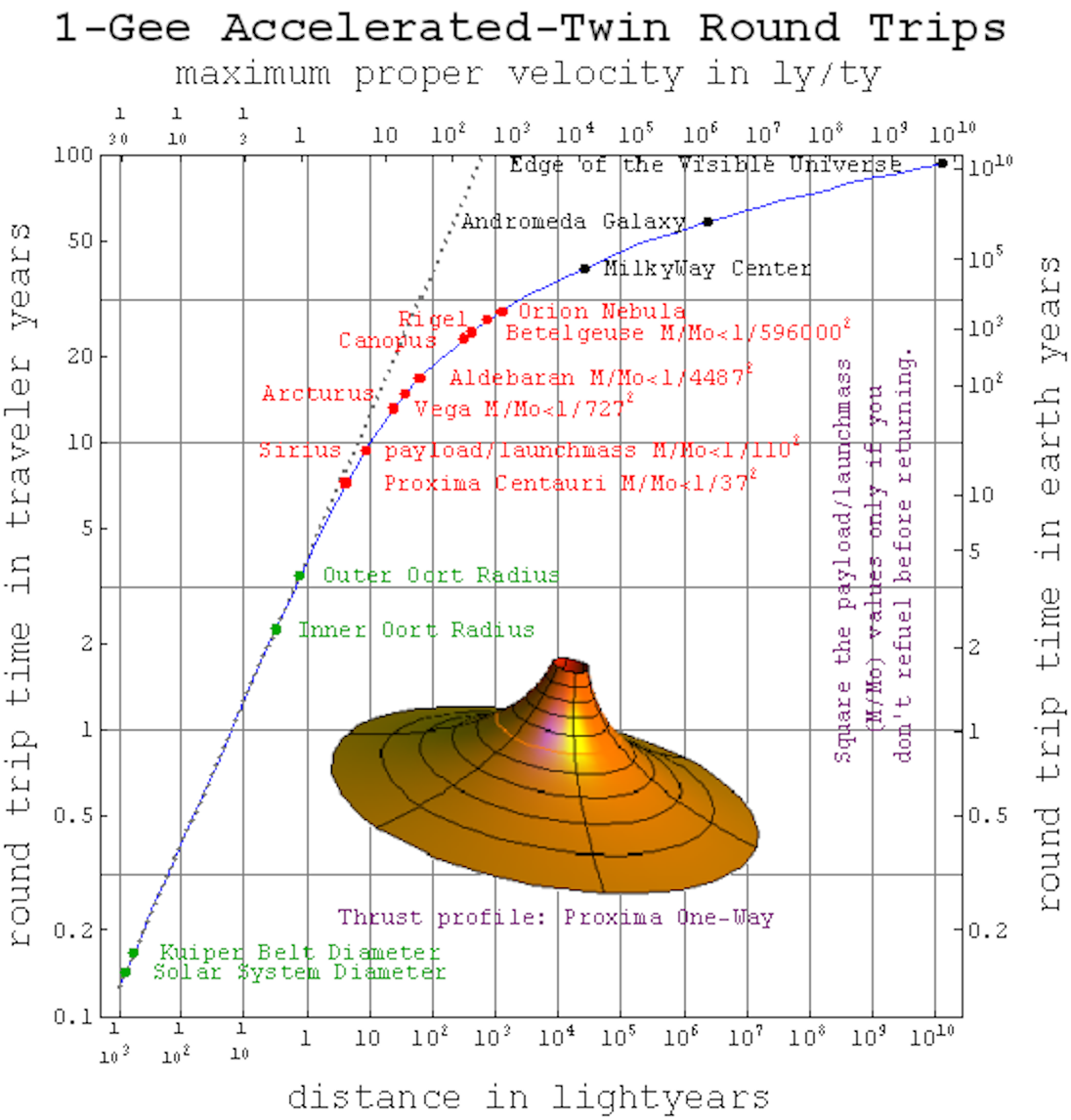
On the other hand, if you don’t want to go the route of traveling as close to the speed of light as possible, perhaps because:
- you learned physics and understand the impossibly large energy requirements to sustain acceleration like this,
- you learned physics and know how rockets must accelerate their future-use fuel as well as the mass of the payload,
- or you learned physics and understand how interstellar/intergalactic matter, including dust grains, stray atoms, and even the leftover radiation from the Big Bang will cause you to “brake” as you travel,
there is another physical option to explore: entering the vicinity of a black hole.
The deeper and deeper into a black hole’s potential well you go, and this is true whether your black hole is non-rotating, rotating slowly, or rotating at nearly the speed of light, the closer you’ll get to the event horizon, and the more severely you’ll find that spacetime is curved. As you enter these regions of increasingly severe curvature, you won’t experience any changes for yourself; time will still appear to pass as normal, and the only physical changes you’ll experience are twofold:
- it will be as though space is “dragging you inward” toward the central singularity, and you’ll have to fire your rocket engines with ever-increasing forces to fight that impulse,
- and the gravitational tidal forces acting on you — i.e., the “tear apart” forces that attract every part of you to the same, singular point — will increase.
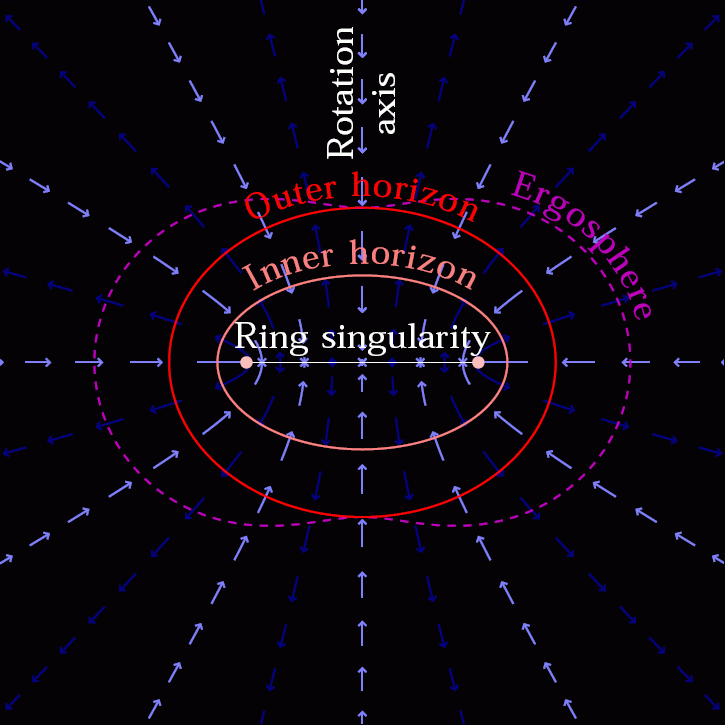
But while you’re spending your time fighting the gravitational pull of the black hole, you’re also spending time in this incredibly, severely curved region of spacetime: where this severe curvature means that time is passing very differently for you compared to an outside observer. The longer you spend there, and the closer you spend your time to the event horizon, the more you exacerbate the difference between your conception of time and the passage of time for the outside Universe.
If this story sounds familiar, it may be because it was a plot point of the movie Interstellar, where a journey deep into a black hole (or its end-to-end connected analogue: a wormhole) causes time to pass at different rates for those who go on the journey versus those who remain at home. In the most severe instances, right up to but just outside the event horizon, mere seconds for you can correspond to billions of years for the outside Universe. The effect of gravitational time dilation, although extremely small for even most cosmic applications (like in binary black hole systems or for gravitationally lensed supernovae), can be extreme just exterior to the event horizon’s edge in general relativity.
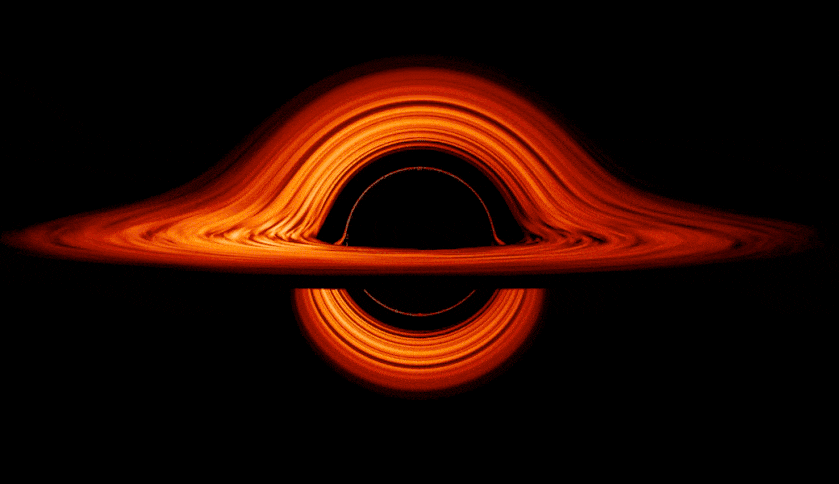
But even through leveraging these tricks, even to the maximum extent that’s physically possible, it still won’t allow you to experience the passage of an infinite amount of time. For the case of traveling close to the speed of light, your motion through space will cause you to inevitably encounter a background of radiation owing to the existence of dark energy: and that radiation will always offer some kind of braking effect that prevents you from achieving truly arbitrary speeds. Similarly, black holes will eventually evaporate due to the related Hawking radiation emanating from them, causing them to decay and leading to the destruction of your severely curved spacetime.
In the end, any observer’s experience of this Universe will still be finite, just as the amount of time that you can exist within it is finite as well. Even though physics may inevitably prevent you from living forever, it does offer two great ways to extend your life to the maximum possible extent:
- by moving as quickly as possible through the fabric of spacetime, leveraging the effects of special relativity and relativistic time dilation,
- or by getting as close as possible to the event horizon of a black hole, leveraging the effects of spacetime curvature and gravitational time dilation.
As long as the known laws of physics remain true, these methods might be the closest way to achieving immortality that any creature within this Universe can experience.