Science’s Greatest Lesson For Humanity Is ‘How To Be Wrong’
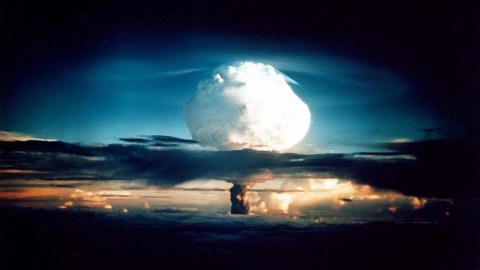
If you don’t learn this one lesson, you’ll not only never be good at science, you’ll never learn anything new.
“Right is right even if no one is doing it; wrong is wrong even if everyone is doing it.” –Augustine of Hippo
For some, science is one of the most frustrating subjects out there. On the one hand, it holds the promise of learning how the world or even the Universe works, and has proven itself more successful than perhaps any other method of inquiry. But on the other hand, it’s incredibly difficult to get it right. Science demands a lot from its practitioners, in the form of problem solving, experimental setup and care, and mathematical analysis. There is no scientist in history, living or dead, who ever got everything right at every turn. We’ve all been mistaken at one point or another, but the greatest lesson that science teaches you isn’t that we’re fallible, but how to behave when you discover that you’re wrong.
Coming up with a scientific worldview is no small feat. You only begin with laws: simple relationships between measurable quantities that might not appear to be superficially related. Relationships between force and acceleration (Newton’s law), pressure and volume (Boyle’s law), or velocity and distance for galaxies (Hubble’s law) aren’t the pinnacle of science, but merely the beginning. Noticing those relationships and applying them is an important step, as it can teach you how to find an unknown quantity if you have the right pieces of information.
But what causes those laws to exist as they do? What’s the reason these relationships are what they are? That requires a deeper understanding: a scientific framework that underlies how these quantities affect one another. Newton’s law exists because momentum is conserved in our Universe; Boyle’s law exists because gases are made of molecules that obey the conservation of energy; Hubble’s law occurs because the Universe is expanding. These are frameworks that you can learn, although the people who came up with them had many false starts before getting it right. Yet the real difficulty comes with the next step.
How do you put the laws and frameworks together in an overarching way to describe a large suite of related phenomena? For example:
- How is momentum conserved in a non-inertial reference frame, or in a gravitational field?
- How do large numbers of particles obeying simple conservation rules behave on a macroscopic level?
- What does the fact that the Universe is expanding imply for the past… and the future?
This requires that you move from a mere law and a framework to a scientific theory. And this is where things get really messy.
Sure, Einstein’s theory of General Relativity answers that first question. The field of statistical mechanics answers the second, and the Big Bang theory, followed by the heat death of the Universe, answers the third. We know that now: in 2017. But we didn’t always know these solutions, and moreover, we know that these aren’t the final solutions to all our problems. A valid scientific theory is the most advanced level of understanding you can reach about our natural Universe, but it isn’t the same as an absolute truth. It’s simply the best quantitative description we have for the Universe today, and it’s always subject to revision, improvement, or even revolution.
Einstein’s Relativity can’t explain what happens to the gravitational field of an electron as it passes through a double slit. Statistical mechanics doesn’t tell you how to avoid quantum decoherence in systems you can manipulate, such as quantum computers. And the Big Bang doesn’t explain where the ultimate birth of space and time came from. These sub-fields of physics — quantum gravity, quantum information theory, and quantum cosmology — are all in their infancies, and mistakes are being make left-and-right by the best and brightest scientists working on them. It doesn’t take long, in any scientific field, before you begin asking questions that bump up against the limits of human knowledge.
But that’s exactly the secret! You ask a relevant question, you test that question out (by performing an experiment, making an observation or measurement, etc.), you collect the full suite of relevant data, and you see if you’ve learned the answer. Sometimes you do; most times you don’t. Then you go back and ask that question in a different way, test that out, and see if you learn anything new. Eventually, hopefully, you gain enough knowledge to come up with a definitive answer to your question. And then you attempt to put the pieces together into a framework or even a full-blown theory that’s full of predictive power, giving you new phenomena to measure and test.
Science is a never-ending story of careful investigation that requires you to continually challenge your assumptions, to revise your understanding in the wake of new and better data, and to improve your methods and ideas over time. It requires you to throw out the frameworks and theories and ideas that didn’t describe reality as well as others did, no matter how elegant or compelling you found them to be. It necessitates not only saying, “I was wrong,” but to seek and find a description of the physical Universe that’s more correct than the wrong ideas you previously held. In short, science teaches you to be humble before the Universe, and the way you do that is by continually looking for superior explanations for everything you can imagine.
Can you imagine a world where humanity valued learning and revising your opinions as much as strongly-held convictions that were unshakable, no matter what the evidence indicated? Where we rejected ideological reasoning in favor of evidence-based decision making? Where actions were judged on their impacts and outcomes, rather than their intentions? Where people were lauded for admitting their mistakes and doing better in the future, rather than requiring them to be some unattainable paragon of infallibility?
The truth is that sometimes, we’re all wrong. That’s the cost of having an original thought; of having an opinion. The big question for each one of us, then, is what we do about it. Do you double-down on your original opinion, seeking ways to justify it, despite the contrary evidence? If you learned the most important lesson that science has to offer, you won’t. Instead, you’ll continue to ask questions about how and why things occur, and how you can test out your own ideas. You’ll see to revise and improve them over time, and to base your decisions on what the full suite of evidence shows. So enough with the false pretenses that we know it all. It’s time to take the most important lessons science has to offer to heart. It’s time to learn.
Ethan Siegel is the author of Beyond the Galaxy and Treknology. You can pre-order his third book, currently in development: the Encyclopaedia Cosmologica.