The most important supernova of the new millennium
How the closest supernova in a generation — soon to be visible to skywatchers almost everywhere — is about to help us better understand the entire Universe.
“I saw a star explode and send out the building blocks of the Universe. Other stars, other planets and eventually other life. A supernova! Creation itself! I was there. I wanted to see it and be part of the moment.” -Ronald D. Moore
It’s one of the most important processes in the Universe. Sure, the stars fuse elements in their cores, turning the primordial hydrogen and helium of the Universe into heavier and heavier elements. But it’s when the more massive stars run out of fuel — or experience an event that triggers a runaway fusion reaction — that the real fun starts.
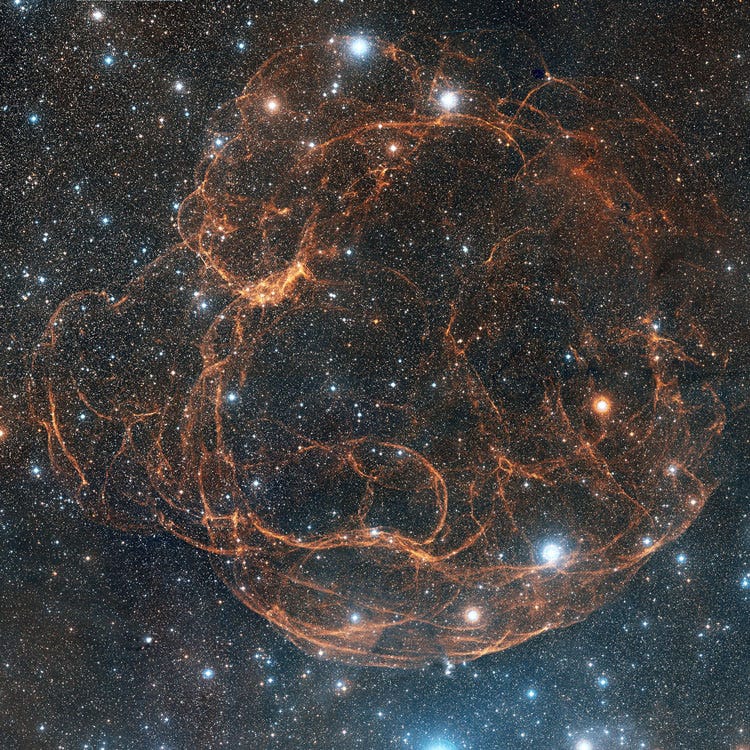
It’s when we get a supernova explosion that the heavy elements, fused within the cores of stars, can actually reach the very end of the periodic table as we know it! Moreover, the stars don’t keep these heavy elements buried within their cores, but rather spit them back out into the interstellar medium, enriching the next generations of stars and allowing for the creation of rocky planets, complex molecules, and — with a little luck — life.
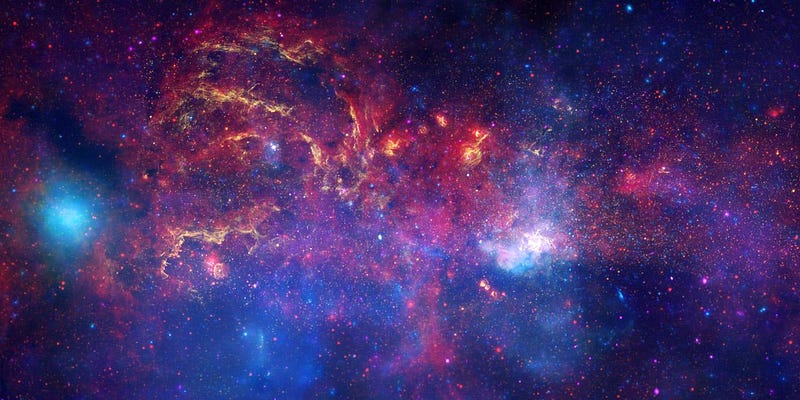
There are two main types of supernovae that occur in the Universe.
The simplest is when a very massive star fuses elements all the way up to iron-nickel-cobalt in its core. All the while, as progressively heavier and heavier elements fuse together, the temperature increases, the radiation pressure increases, and it needs to, to prevent the core from collapsing under the tremendous mass of the surrounding stellar envelope. But iron-nickel-cobalt are the most stable elements (per-nucleon), and they’re like “ash” in the sense that you’re unable to extract energy from them by burning them further. With the incredible mass pushing in on them, they ignite a runaway collapse in the core of the star, producing a spectacular Type II supernova explosion.
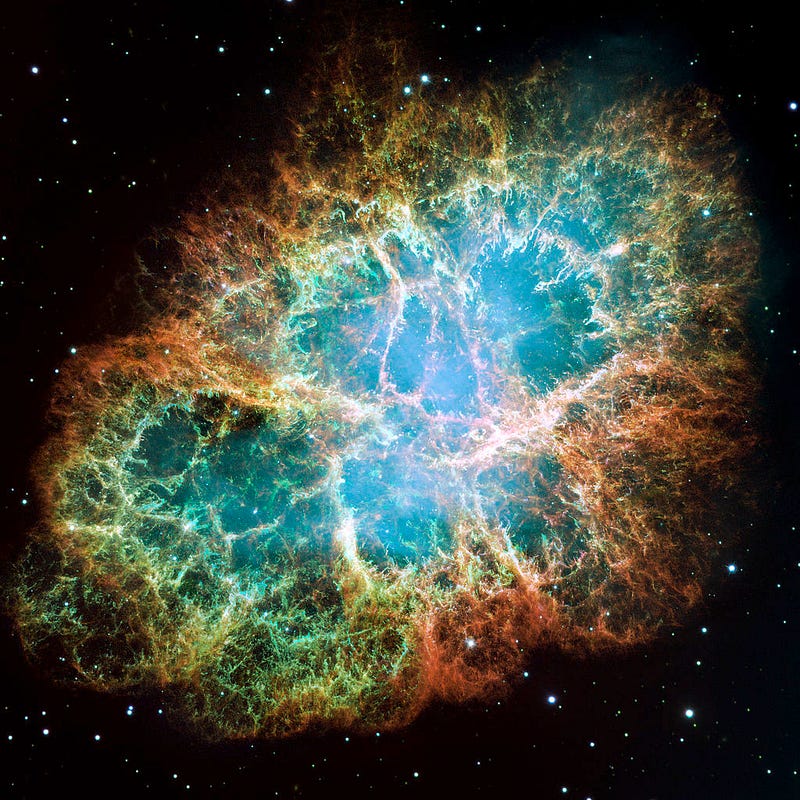
But stars come in a huge variety of masses, their outer layers are often stripped off in various amounts, and the properties of these supernovae vary wildly. They are important for enriching the interstellar medium and the main source of elements heavier than helium in the Universe today, but they’re not particularly useful for understanding how far away objects are.
But there’s a second main type of supernova, and every star that wasn’t massive enough to have this story, above, be its fate — so 799 out of every 800 stars — get a second chance.
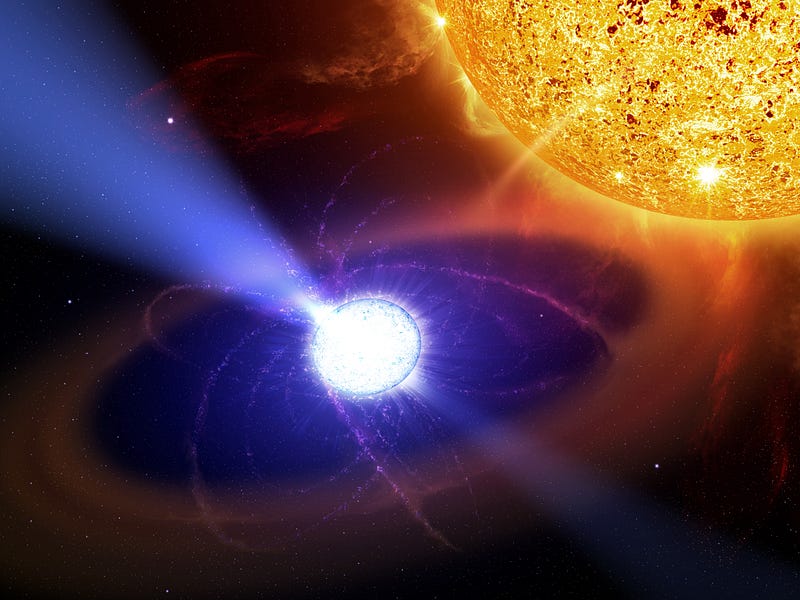
When less massive stars — including our Sun, in the future — run out of the fuel in their core, their outer layers are blown off, while the inner layers contract down to a white dwarf: a very massive object around the size of the Earth, but hundreds of thousands of times as dense and massive. The white dwarf fuses no elements in its core, but instead the pressure from the electrons inside of it prevent gravity from collapsing the star any further.
There is, however, a limit to the mass of a white dwarf: about 140% the mass of our Sun. Beyond that, and the electrons won’t be able to prevent that collapse. Even though the vast majority of stars produce white dwarfs that are below this mass threshold, that’s not the end of the story, not by any means!
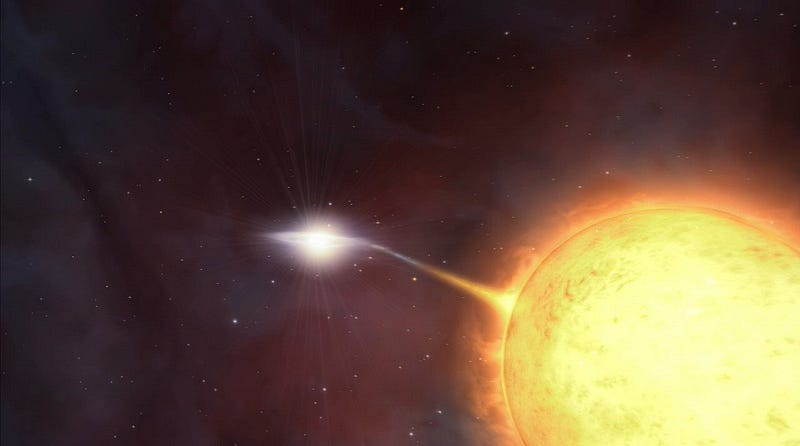
Many (if not most) star systems have multiple stars in them, unlike our own. A white dwarf is incredibly dense, and — if the conditions are right — it can gradually siphon mass off of its companion star until it passes that all-important threshold. There are other ways to beat that limit, too: you can collide with another star, or, most commonly, you can have a second white dwarf spiral in and collide with the first one.
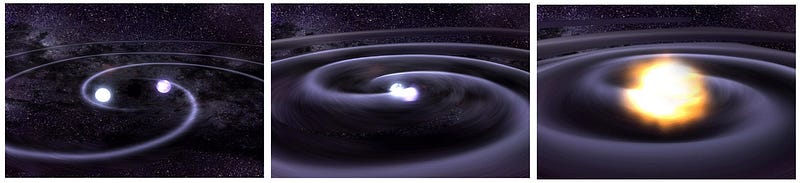
And when any of these things happen, it triggers a runaway fusion reaction in the interiors of these white dwarfs, resulting in the other common type of supernova seen in our Universe: Type Ia supernovae! These supernovae are incredibly important not only because of how common they are, although they are common: the last supernova visible to the naked-eye here on Earth, the one that happened in our own galaxy in 1604, was a type Ia! If you look in plain-old visible light, you can see the fireworks from that light show even today.
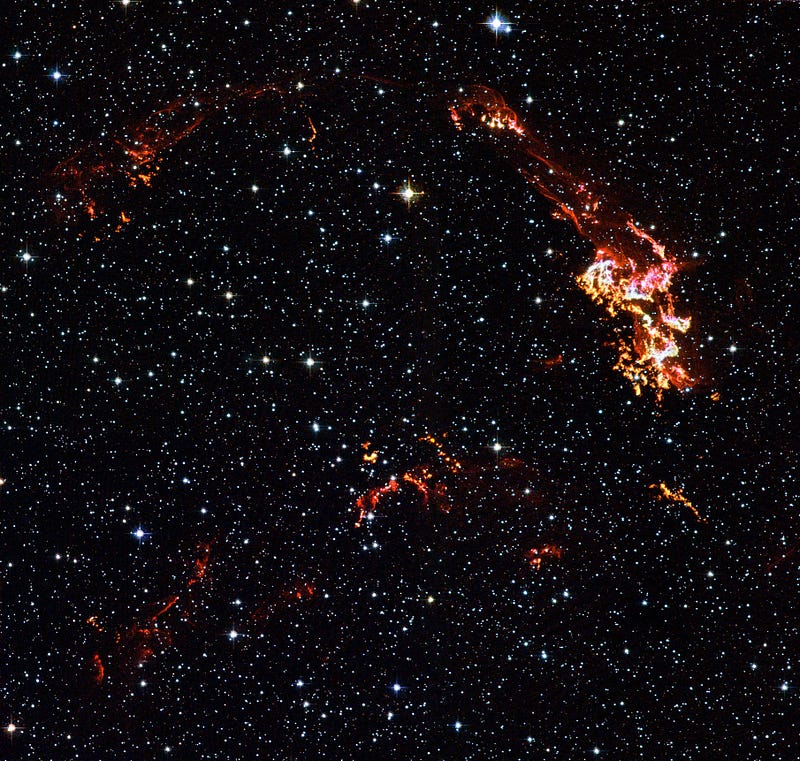
But there’s an important physical reason to look for these supernovae: not only are they ubiquitous and bright, but the light coming from them has very special properties: their peak brightnesses, time of brightening-and-dimming, and other light-curve properties are very well understood, and very close to universal.
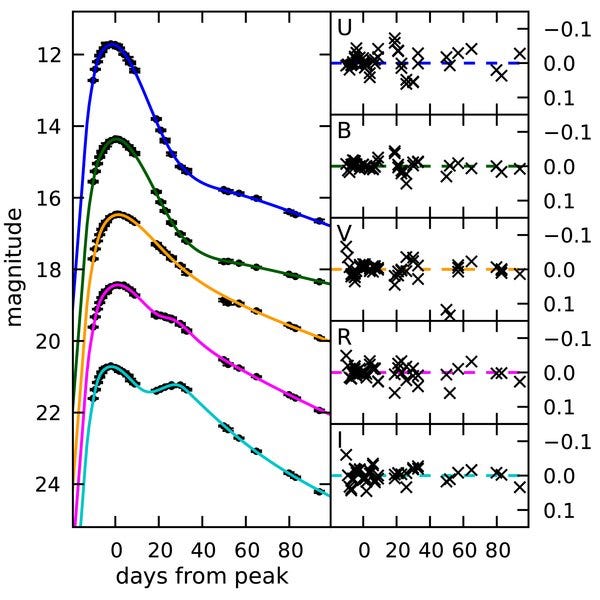
doi:10.1088/0004-637X/707/2/1064.
What this means, practically, is that if you measure the light-curve of a Type Ia supernova, and you measure how bright it appears to be to us, we can figure out how intrinsically far away the galaxy that it occurred in must be! That’s one of the most powerful things one can do in astrophysics — learn how far away a distant object is — because we can better understand how the Universe has expanded over its entire history with that information.
Using Type Ia supernovae was, in fact, how dark energy was discovered, and just two years ago netted the Nobel Prize in physics.
Part of the reason that this is such a powerful method is that the detonation inside a white dwarf when it goes (Type Ia) supernova is thought to be pretty much the same regardless of how it happens.
The toughest part of using this method — and the biggest uncertainty, scientifically — is the fact that the environments where these supernovae occur aren’t uniform. Even though the explosions themselves might be very clean, there’s always one of the astronomer’s greatest enemies to combat: light-blocking dust. It’s the reason we can’t see through the plane of our own galaxy, it’s the reason we have such a hard time seeing where new stars are forming, and it’s the number one source of uncertainty in our understanding of Type Ia supernovae.
If only we had a nearby one to study with today’s modern technology.
If only.
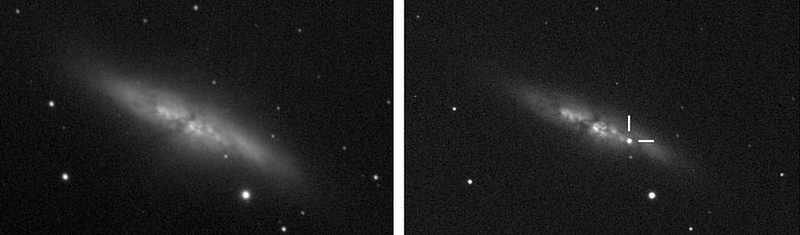
Well, guess what? The Universe has just answered that prayer for us! Some of you may recognize the galaxy, above: it’s the Cigar Galaxy, Messier 82, one of the closest and brightest galaxies in our night sky, located just 11.5 million light-years away.
And that new “point of light” you see, on the right, is a Type Ia supernova!
What’s really amazing about this is that M82 is an edge-on galaxy, full of dust, and full of new star-forming regions thanks to its gravitational interactions with its larger, more spectacular neighbor.
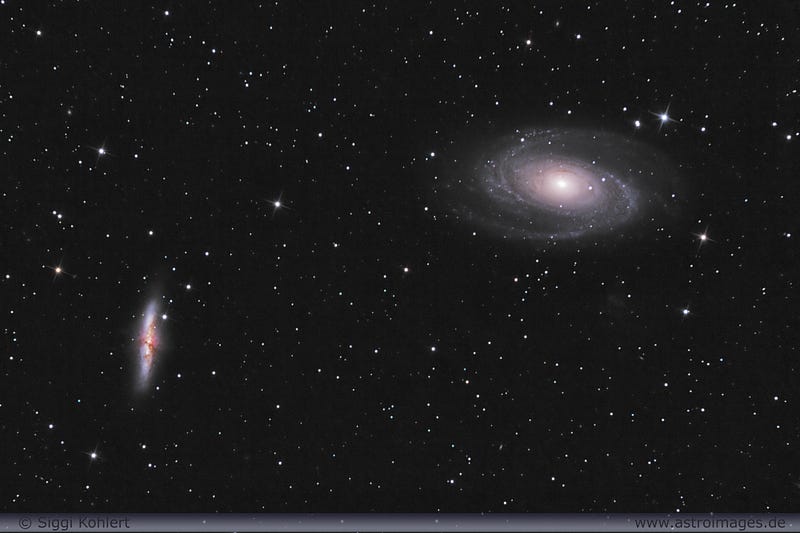
In fact, this is one of the most frequently observed galaxies in the entire night sky, and you yourself can see it quite easily with modestly dark skies and a pair of binoculars! Here’s how you can do it, from pretty much anywhere north of the Tropic of Capricorn.
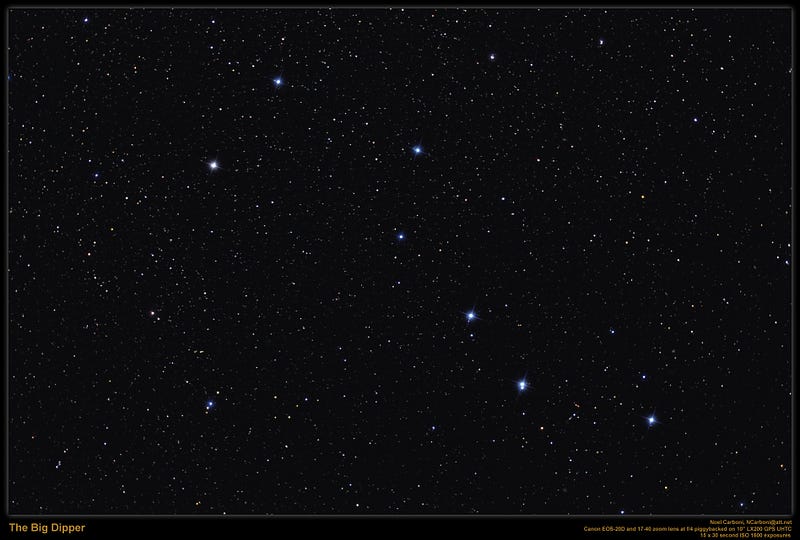
If you can identify the Big Dipper, you’re well on your way. The “cup” of the dipper has four stars in it, and the two you’ll need are the one at the very edge, Dubhe, and the one diagonal to it at the bottom of the other edge of the cup, Phad. If you draw an imaginary line from Phad to Dubhe, and then extend that line for an approximately equal distance beyond Dubhe (bent ever-so-slightly), just point your telescope/binoculars at that area of sky.
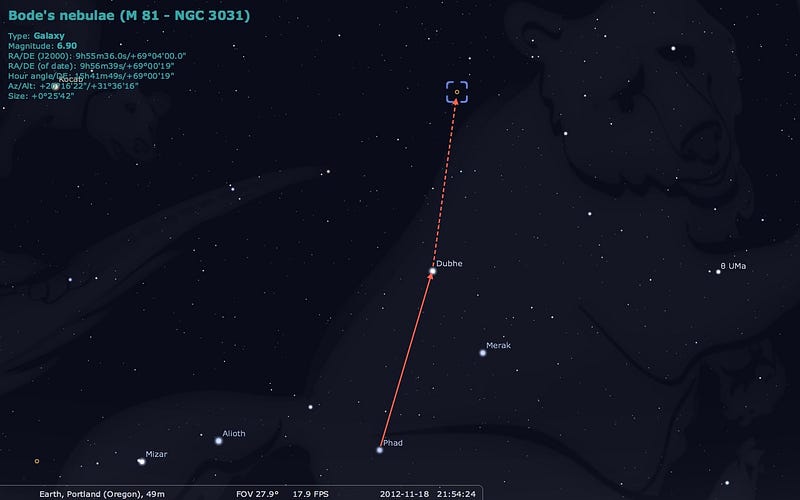
You’ll see that pair of galaxies — Messiers 81 and 82 — as two faint cloud-like objects that don’t move. The smaller one, Messier 82, is undergoing a tremendous burst of star formation, and has had three supernovae — all Type IIs — in the past decade.
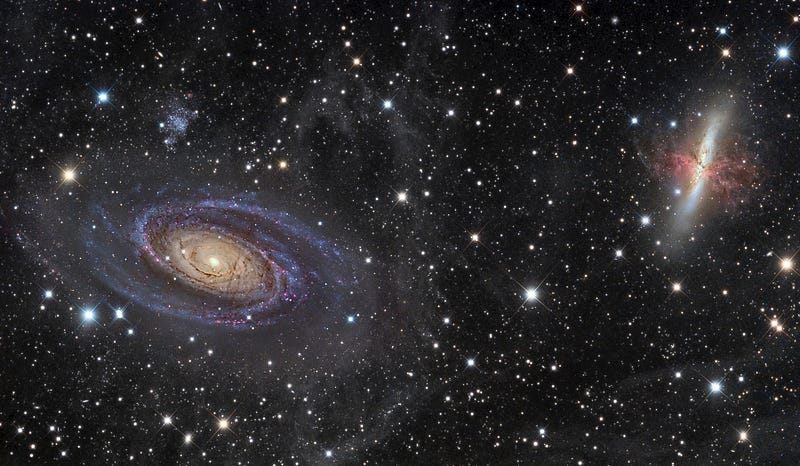
But this one is different! Rather than the kind formed by the end-of-life of very massive stars, this is the rarer type, formed from the death of a white dwarf star!
As you can see in the animation below, there’s a new “point of light” that was just discovered, and it will continue to brighten over the coming weeks and months.
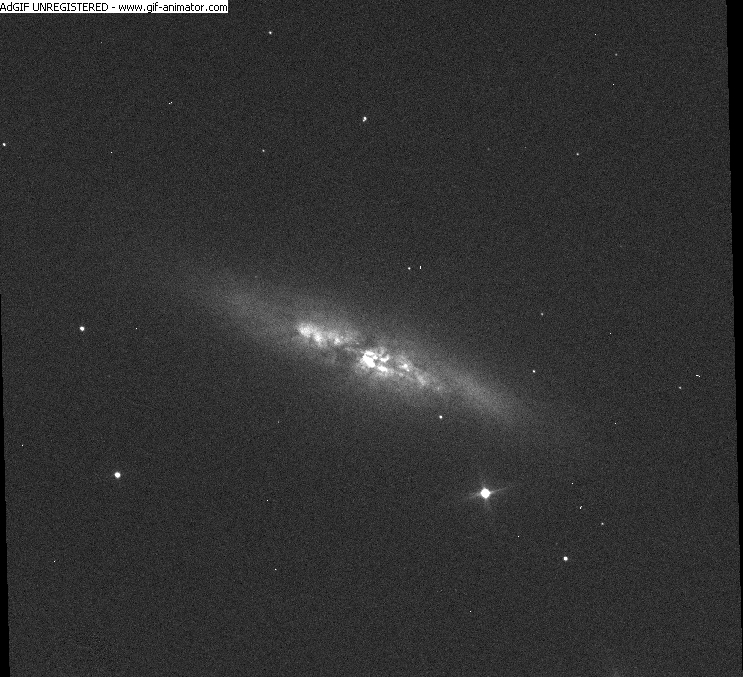
It’s the new “point” in the plane of the galaxy, and it’s spectacular! A few years ago, there was another close supernova, but this one is less than half the distance from us, making it the closest one since 1987, and the closest Type Ia in centuries! As the data continues to come in, we’re going to learn just how the dust affects the light coming from these objects, and this is going to be of tremendous importance in understanding how dusty environments play a role in the light that comes from Type Ia supernovae.
It’s not only no stretch to say this is the most important supernova of the new millennium, but that it’s got the potential to help us understand how the Universe has expanded over its history better than ever before!