Throwback Thursday: The Little Bit Of Dark Matter We Know
Dark matter makes up the vast majority of mass in the Universe, and most of it is unknown. But not all of it.
“A cosmic mystery of immense proportions, once seemingly on the verge of solution, has deepened and left astronomers and astrophysicists more baffled than ever. The crux … is that the vast majority of the mass of the universe seems to be missing.” –William J. Broad
It was in the 1930s, looking at dense clusters of galaxies (like Coma, below), that Fritz Zwicky first noticed that the mass in the Universe didn’t add up.
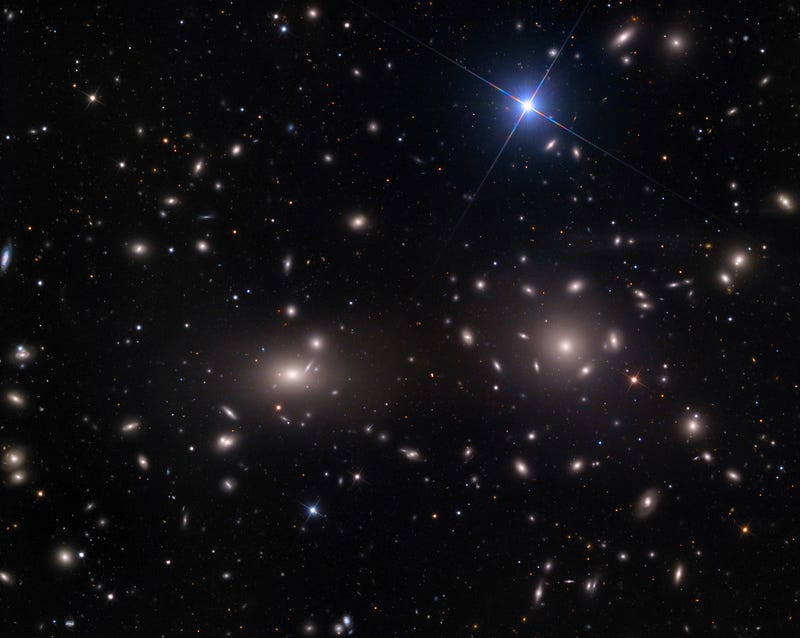
We knew how gravity worked, so it was pretty straightforward — based on how the galaxies within the cluster were moving — to calculate how much the total mass of this cluster was.
It was also straightforward, because we knew how stars worked, too, to calculate how much mass was in all the stars in all the galaxies that made up the cluster. All you had to do was measure the starlight, and there you had it: two different ways to measure the mass of the same set of objects.
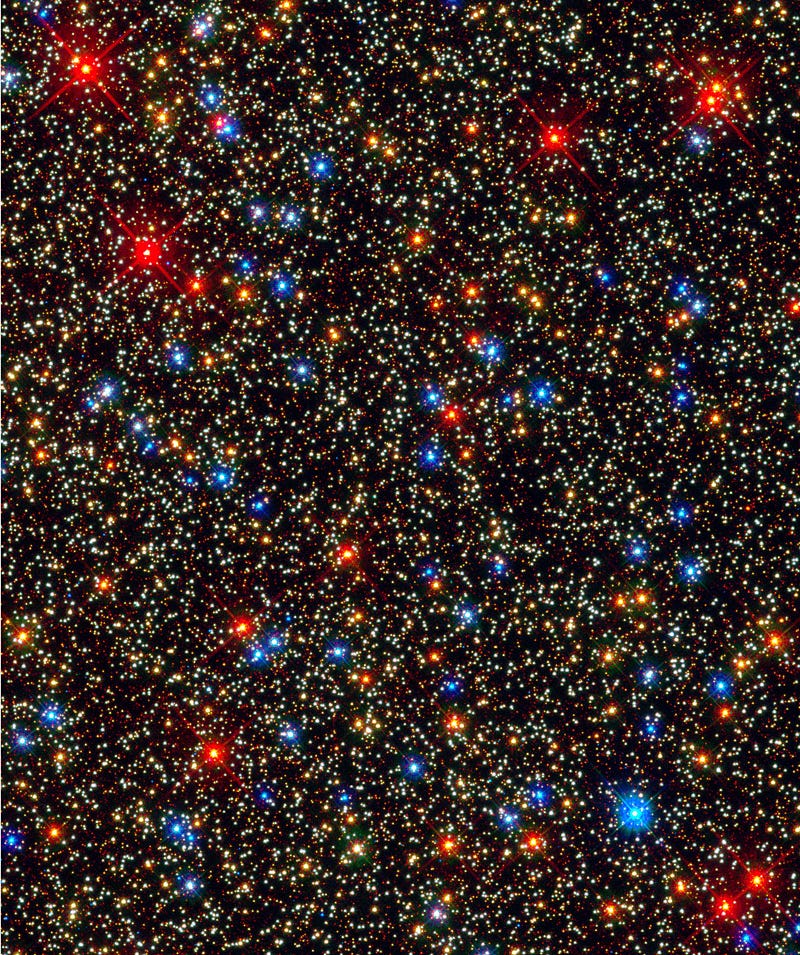
At least, you’d expect these two numbers to match up, if the stars were what made up the mass in the Universe. It turns out not to be the case, and it turns out not to be close: the two numbers you get for mass — the one from gravity and the one from starlight — differ by a factor of fifty.
“That’s okay,” you say. Because there’s ever so much more than stars out there.
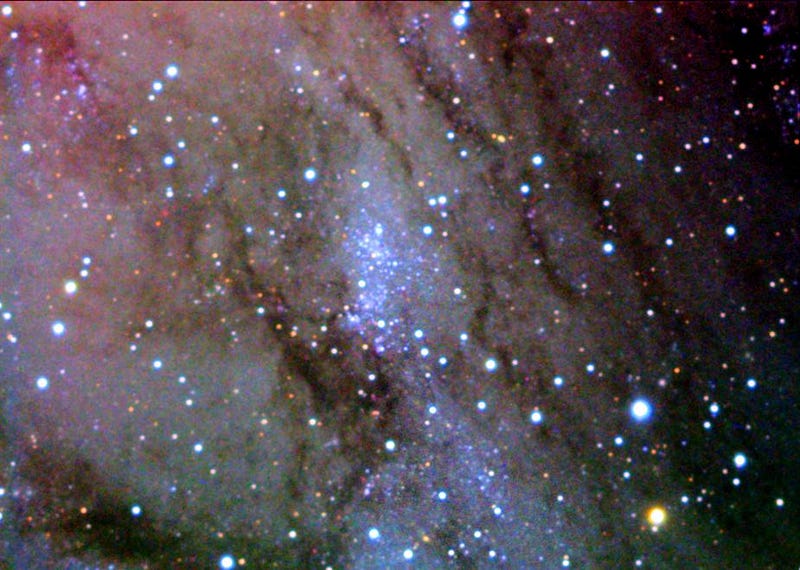
Matter not only clumps and clusters into stars, but also planets, gas, dust, plasma, asteroids, comets, ice, and — every once-in-a-while — living creatures. So you could imagine that this “missing mass” out there, the other 98%, was some other form of protons, neutrons, and electrons like the stars are.
We actually have three completely independent ways to measure, very accurately, just how much of the Universe is made out of this “normal stuff” that we’re familiar with.
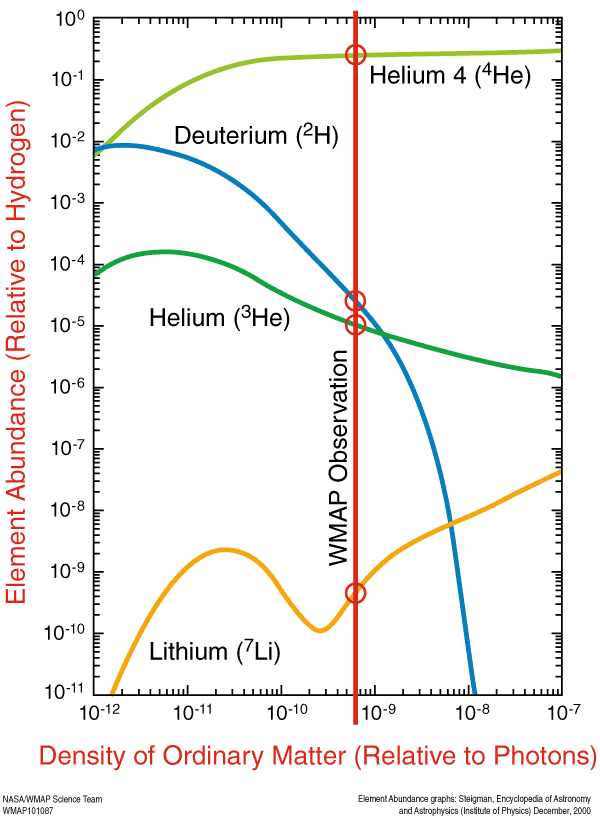
We can trace back the physics of the Early Universe to the first few minutes after the Big Bang, and calculate how much of the light elements — hydrogen, helium, lithium and their isotopes — should have been created during this period of Big Bang Nucleosynthesis (BBN). It’s dependent on one parameter and one parameter only: the ratio of number-of-baryons (protons and neutrons combined) to photons (which we can count). So we measure the light elements and we get a number for how many protons and neutrons (and since the Universe is neutral, we know electrons, too) there are in the Universe.
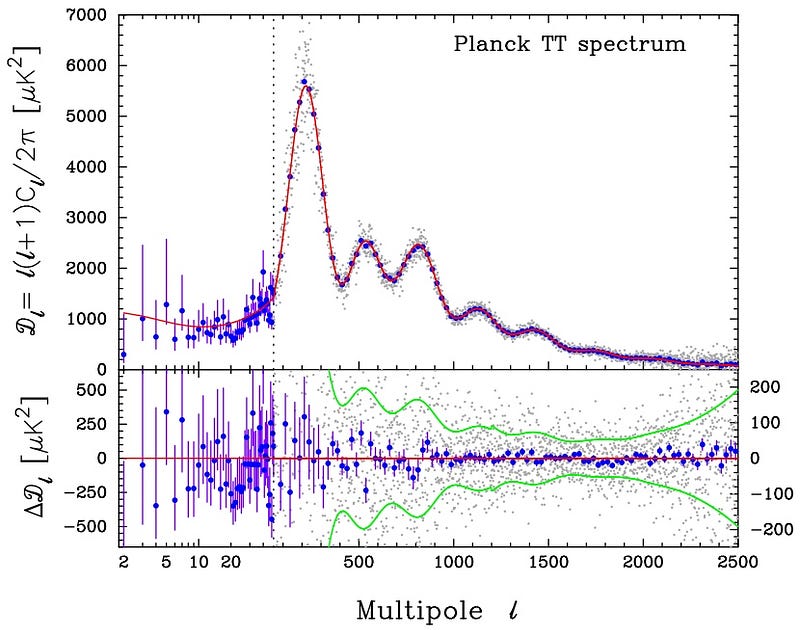
We can look at the pattern of fluctuations in the Cosmic Microwave Background (CMB). The positions and heights of the different peaks tell us two things: how much normal matter (protons, neutrons and electrons) there is in the Universe, as well as how much total matter (all things with a gravitational mass, combined) there is.
Again, we get a number.
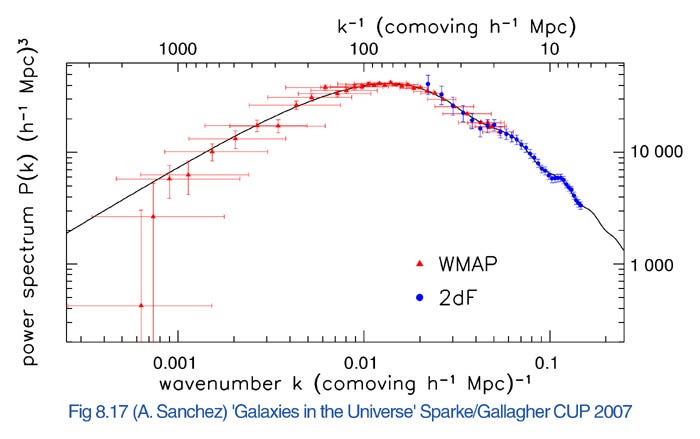
And finally, we can look at the Universe on the largest scales. We can observe how galaxies, clusters and superclusters of galaxies group together, and probe the Universe’s Large Scale Structure (LSS). The amplitude of those wiggles — as well as the overall height of this graph (the power) — gives us a different way of measuring those same two things: the amount of normal matter and the amount of total matter.
What we find is in remarkable agreement. All three measurements (BBN, CMB, and LSS) all give the same values: about 15% of the total amount of matter — including the 2% that’s in stars — is normal matter, and the rest of it, some 85%, is matter that doesn’t emit or absorb light. Throw in the fact that all of this matter combined is just over 30% of the total energy in the Universe (with the rest being dark energy), and we find that less than 5% of the Universe is made up of normal matter, using three independent measurements.
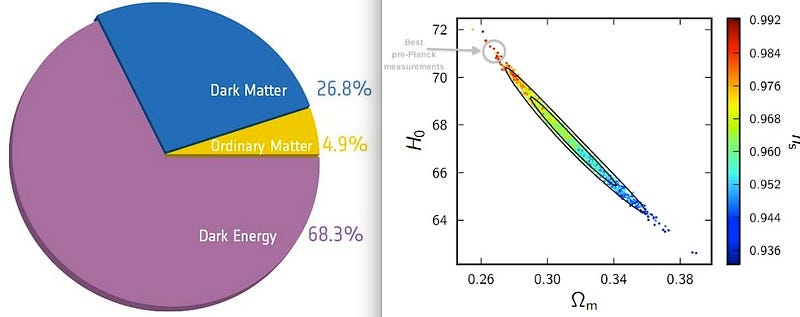
So what is that dark matter? What is that missing mass that doesn’t interact with light?
Believe it or not, there’s a candidate particle — the second most abundant type of known particle in the Universe — that comes to us directly, leftover from the Big Bang: the neutrino!
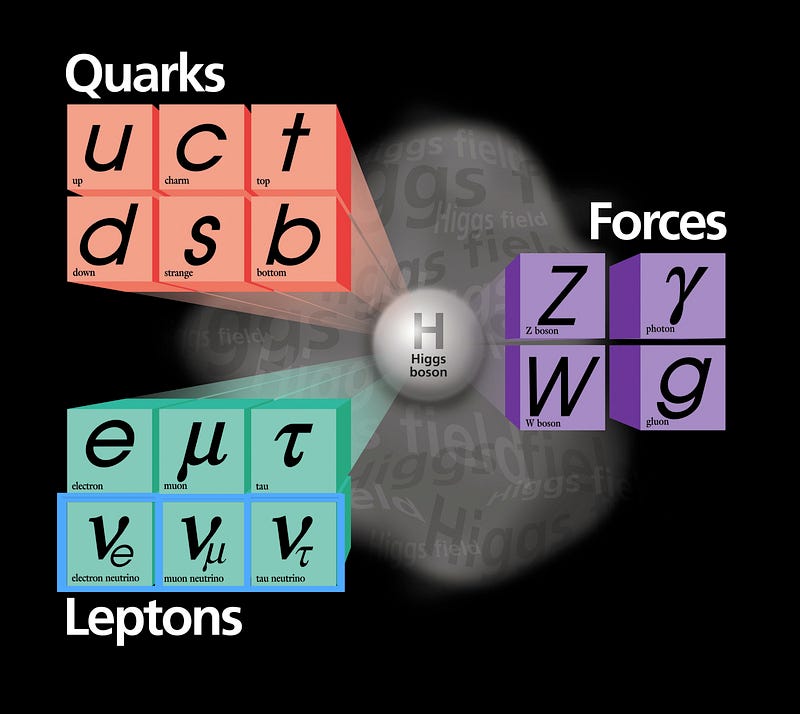
Particle-antiparticle pairs were all created in the early Universe indiscriminately and in great abundance, and there was a time when temperatures and densities were so high that even the humble neutrino (and the antineutrino) were created in that same great abundance.
But when the Universe was very young — about a single second after the Big Bang — neutrinos froze out, which means that temperatures and densities dropped enough so that they stopped interacting with other forms of matter and with themselves. If neutrinos had been completely massless, they would have had an energy spectrum and distribution very similar to what leftover photons from the Big Bang — the Cosmic Microwave Background — has today.
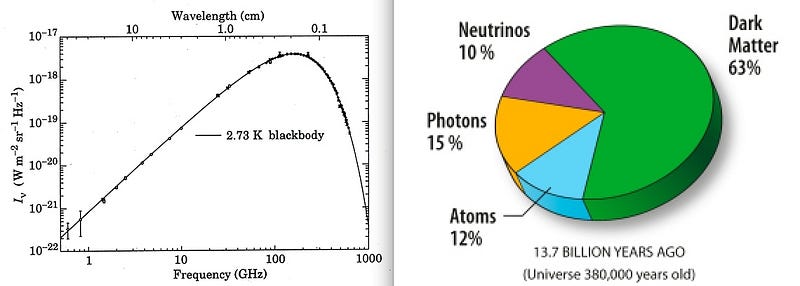
There would be slight differences: the neutrinos would be at a slightly lower temperature (1.96 K), they would have had only about 2/3 the total energy of the photons (due to both temperature and particle statistics differences), and there would be just under 300 of them permeating every cubic centimeter of the Universe. This was the case — that their behavior was analogous to this — back when the CMB was first emitted, and that’s why we’ve been able to detect the signatures of these neutrinos in the CMB!
But that’s assuming neutrinos were massless; if they had a mass — even if that mass were tiny compared to the other known particles — the expansion and cooling of the Universe could have left these cosmic neutrinos, outnumbering protons by more than a billion-to-one, as the primary mass source of the Universe!
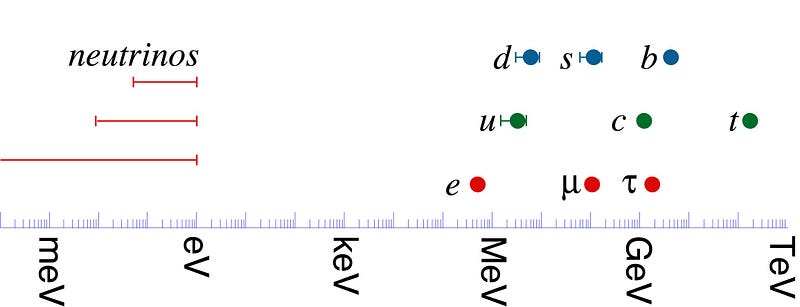
If the neutrinos — and remember, there are three different types, all in equal abundance to one another — had had a mass of just 3.7 eV apiece (and remember, the next lightest particle, the electron, has a mass of about 511,000 eV), then 100% of the dark matter would have been accounted for by neutrinos. In fact, it wouldn’t have mattered how it was distributed; so long as the masses of the three types of neutrinos (e, μ, and τ) had summed up to 11.2 eV, they would have been all of the dark matter.
So a little bit could have gone a really, really long way!
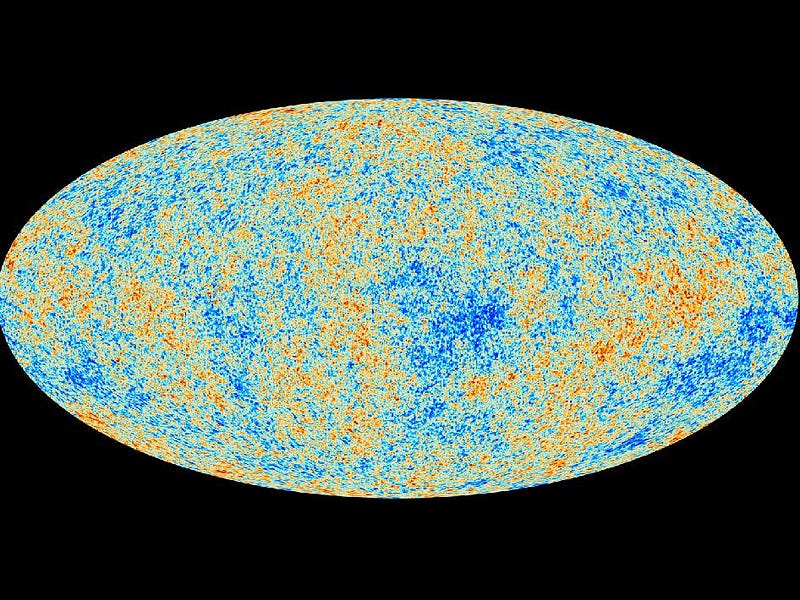
But even the most beautiful theory, at the end of the day, has to contend with the Universe as it actually exists. From measurements of the cosmic microwave background, where the strongest constraint comes from, we find that the upper limit on the sum of the masses of the three types of neutrino is only 0.18 eV, which means that a maximum of 1.6% of the dark matter is in the form of neutrinos.
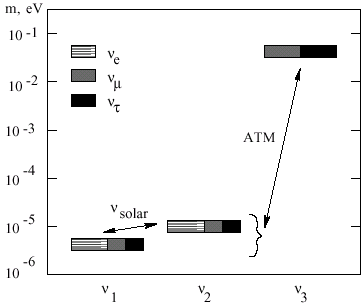
And from observations of neutrino oscillations, we know that there’s a lower limit on the sums of the neutrino masses: 0.06 eV, meaning that at least 0.55% of the dark matter is in the form of neutrinos.
Neutrinos were moving quickly when the Universe was younger, which means it’s a form of hot dark matter. Dark matter that was moving slowly when the Universe was younger was colder, and different structures form on different scales in the Universe dependent on whether the dark matter is hot, warm or cold.
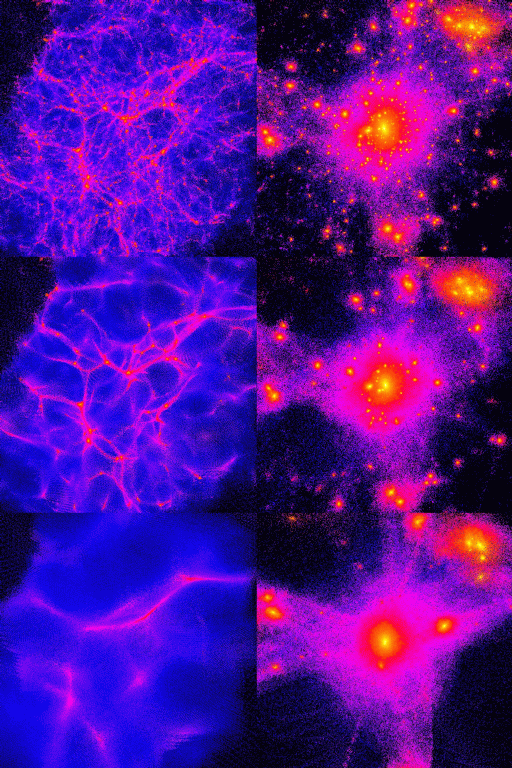
While large-scale structure tells us that the vast majority of the dark matter must be either cold or warm, we know that there is a tiny bit of hot dark matter, and that’s in fact what the neutrinos are! So while the large scale structure in the Universe agrees (within the measurable errors) with cold dark matter (CDM, in the figure below), we know that there’s a tiny mix — between 0.55% and 1.6% — of Hot Dark Matter, in the form of neutrinos, thrown in there!
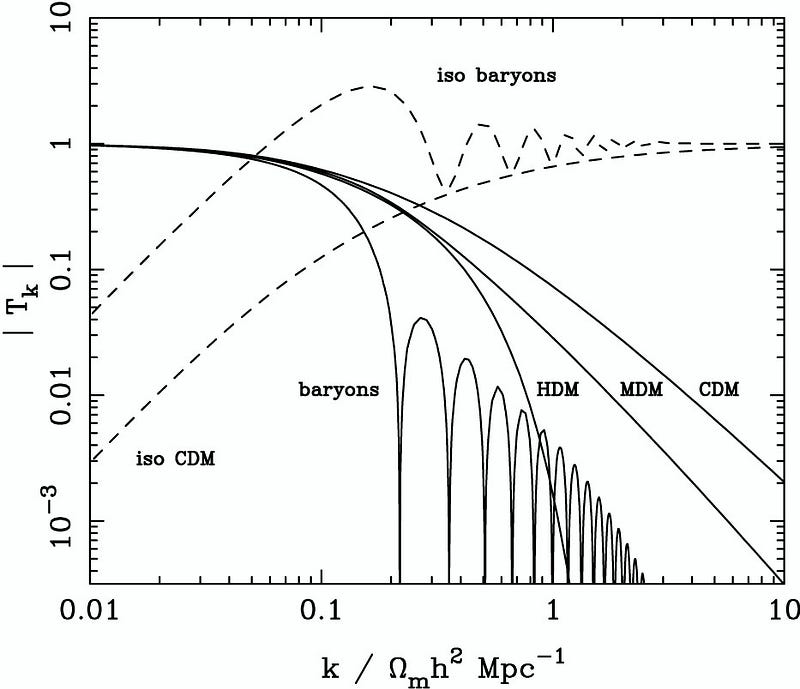
Now getting the other 98.4-to-99.45% of non-baryonic dark matter has proved elusive so far, and we’ve been looking in earnest. But neutrinos — the only form of dark matter beyond baryons that we know of — are not only the tip of the dark matter iceberg, they’re actually the only bit of dark matter we know and understand today! Looking at all the matter in the Universe, here’s how it’s made up:

About 15.5% is normal matter: stuff made out of protons, neutrons, and electrons in all their different forms.
Between 0.5% and 1.4%, of all the total matter, is neutrinos: the particles with the smallest non-zero mass known in the Universe, outnumbering protons, neutrons and electrons by more than abillion to one.
And, alas, about 83% of the Universe is some “other” form of dark matter, which must either be cold or warm (and not hot), and which we still haven’t found.
And that’s the story of the little bit of dark matter that we know!
Leave your comments on our forum, and support Starts With A Bang on Patreon!