What Was It Like When The Universe Made Its Second Generation Of Stars?
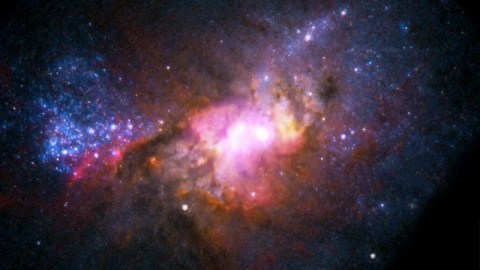
The Big Bang happened everywhere at once, but stars are a different story.
The Universe, back at its inception, was almost perfectly identical everywhere. It was the same high temperature everywhere, the same large density everywhere, and was made up of the same quanta of matter, antimatter, dark matter, and radiation everywhere. At the earliest times, the differences were at the 0.003% level, owing to quantum fluctuations left over from inflation.
But gravity and time have a way of changing everything. Antimatter annihilates away; atomic nuclei and then neutral atoms form; gravity pulls matter into overdense regions, causing them to grow. Because overdensities differ by such great amounts on all scales, there are regions where stars form rapidly, within 100 million years or fewer, while other regions won’t form stars for billions of years. But where the earliest stars form, that’s where the most interesting things happen first.
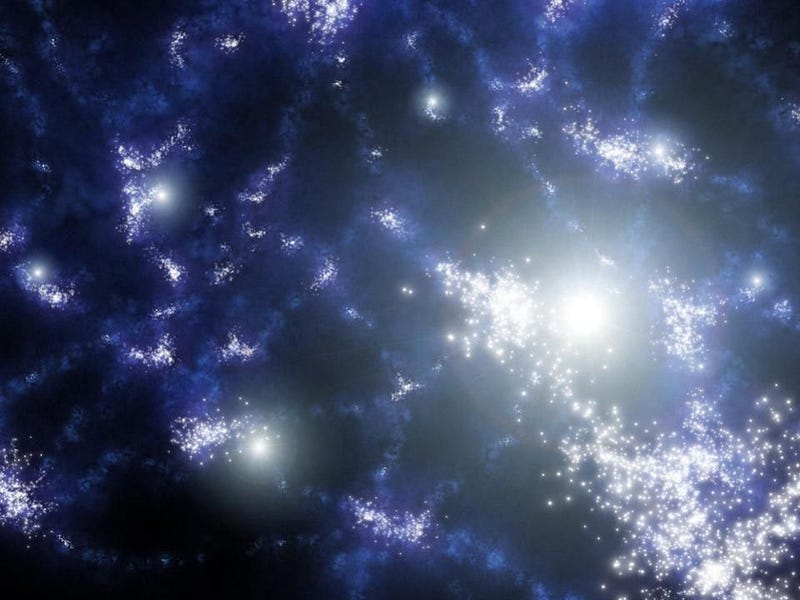
The very, very first stars are born somewhere between 50 and 100 million years after the Big Bang, and are much more massive than the stars we see today. As very massive stars, they live fast, burning through all their fuel within just a few million years and dying with either a supernova or a direct collapse to a black hole.
And where this happens, that’s the end for the first stars. The outer layers of the stars that went supernovae, making up the majority of the former star’s mass, get blown off back into interstellar space. The neutron star remnants, many of which are in binary systems, have a chance to collide with other neutron stars, giving rise to gamma-ray bursts and the heaviest of the elements. All of a sudden, it’s not just hydrogen and helium anymore.
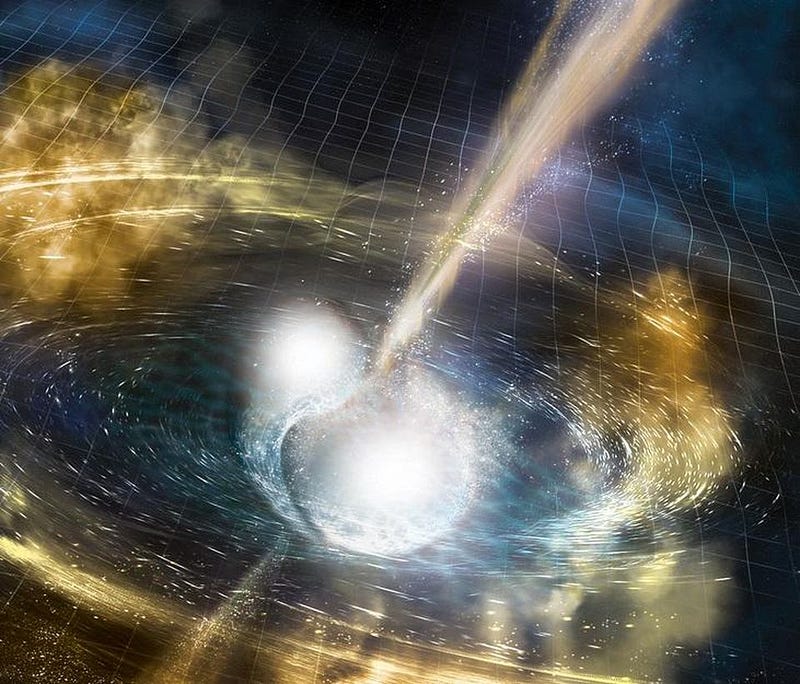
After all the millions of years it takes the first stars to form — perhaps as little as 50 million in some places, typically between 200 and 550 million in most, but not for 2 or 3 billion years in the rarest regions — they run out of fuel and die in as little as 2–5 million years. These very first stars, made out of the pristine elements formed just 3–4 minutes after the Big Bang, have practically no survivors for very long, as they’re all quite massive compared to stars today.
But now, the interstellar medium is enriched. It no longer has hydrogen and helium and one-in-a-billion parts lithium with nothing heavier, but suddenly there are abundant levels of carbon and oxygen, with copious amounts of silicon, sulfur, and iron, nickel, and cobalt, plus all the elements made in supernovae and kilonovae. It’s from these enriched materials, now flooding the interstellar medium, that the next generation of stars will form.
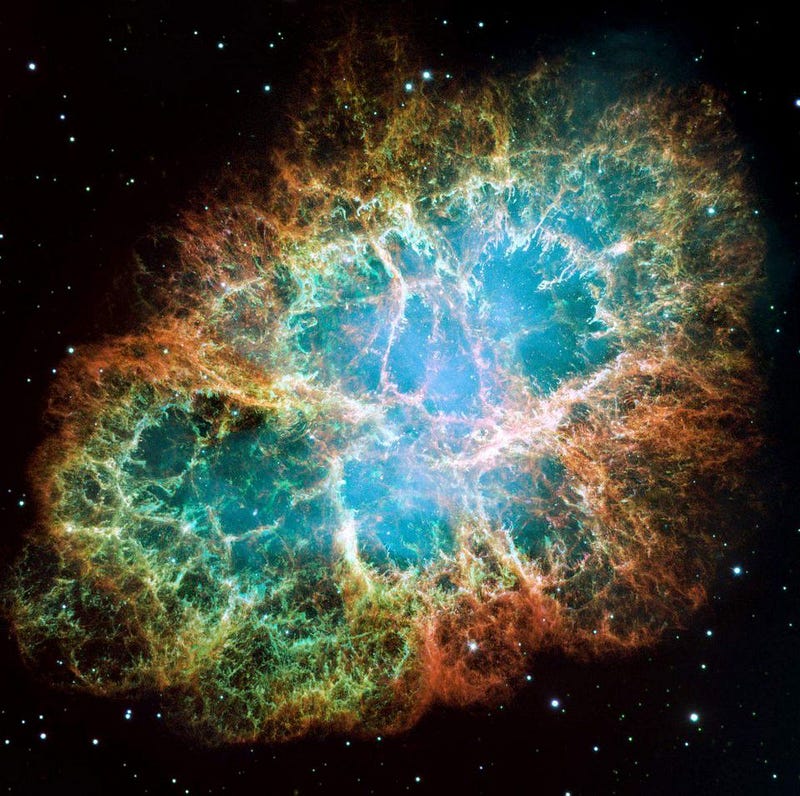
From the closest supernova remnant to us, the Crab Nebula, we can infer that every explosion pushes material outwards at approximately the rate we observe there: creating a nebula 10 light years across after approximately 1,000 years. Wherever the debris from the deceased first generation of stars cannot yet reach, the stars that eventually form there will still be pristine, since there’s no way for that processed material to make it into those pre-stellar nebulae.
But where the debris does reach, all of a sudden that material that’s available to form stars is full of atoms with heavier nuclei. It might seem silly to you, under most circumstances, that astronomers throw every element heavier that helium into its own class — and call them “metals” — but this is really a big deal.
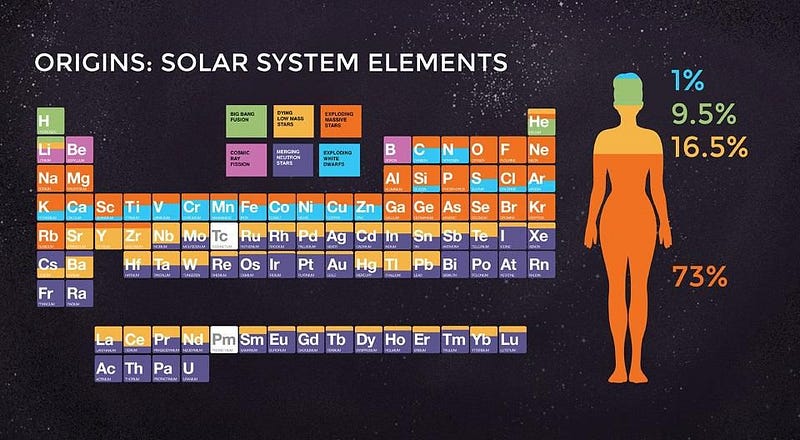
You see, when you form stars out of hydrogen at helium alone (in a metal-free environment), there’s no efficient way to radiate away the heat generated by gravitational collapse. Therefore, you need to have enormous clumps of matter in order to trigger gravitational collapse, leading to extremely massive stars, even on average.
But when you have metals present, even if they’re just 0.001% of the total fraction of atoms, they’re the excellent energy-radiators that the first stars were missing. As a gas cloud with these heavy elements collapses, heat radiates away much more efficiently than before, allowing the proto-stars to collapse much more quickly and with much lower masses.
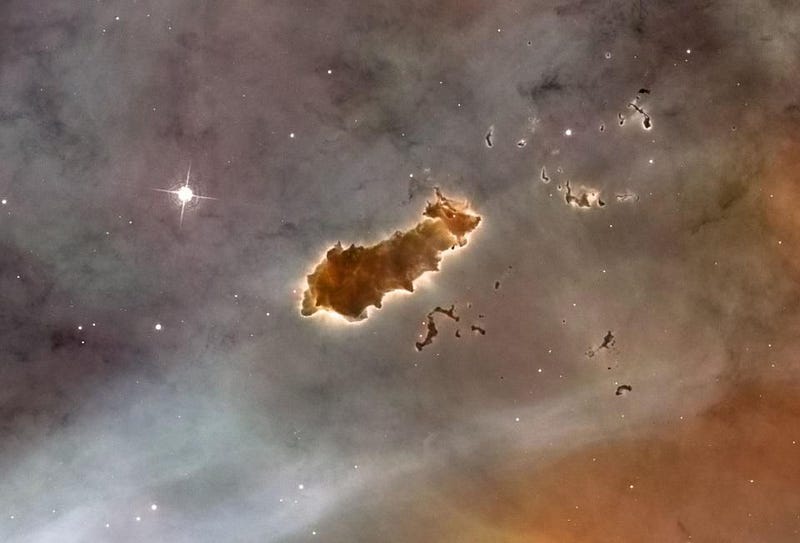
Additionally, nearby supernovae and other violent events can even, oftentimes, serve as a trigger for gravitational collapse and new star formation. The first stars don’t just provide the materials for a second generation of stars to form, but the impetus, especially in a gas-rich environment, to set them on their path.
The big result is that, shortly after the first stars form, live, and die, there will be another generation that crops up, wildly different in character than the first. These second-generation stars are no longer 10 solar masses, on average, but run the full gamut of star sizes and masses. Perhaps, if our understanding of star formation is correct, they’re similar to the stars we form today: 0.4 solar masses on average.
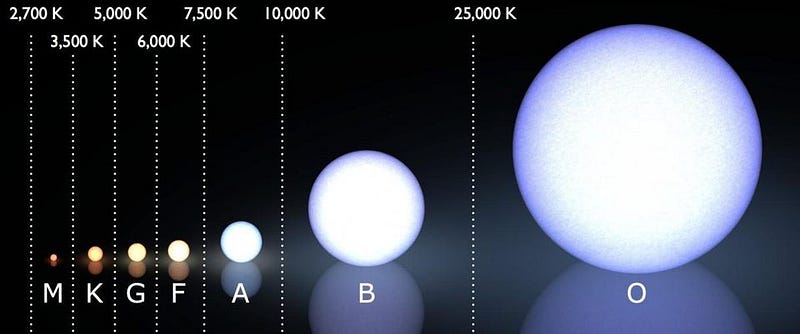
Yes, there will still be a few large, massive stars, but they won’t be as massive as the biggest among the first stars. There will be additional supernovae, neutron stars, and kilonovae that result. But in very short order, the earliest, first stars will wipe themselves out wherever they exist, only to be replaced by this second generation of stars, rife with smaller, redder, and less massive members.
As a result, in the very young Universe, we expect to see populations of first stars, which are exclusively hot and blue, alongside older regions, which already have black holes, second-generation stars, and low-mass, low-luminosity stars among them.
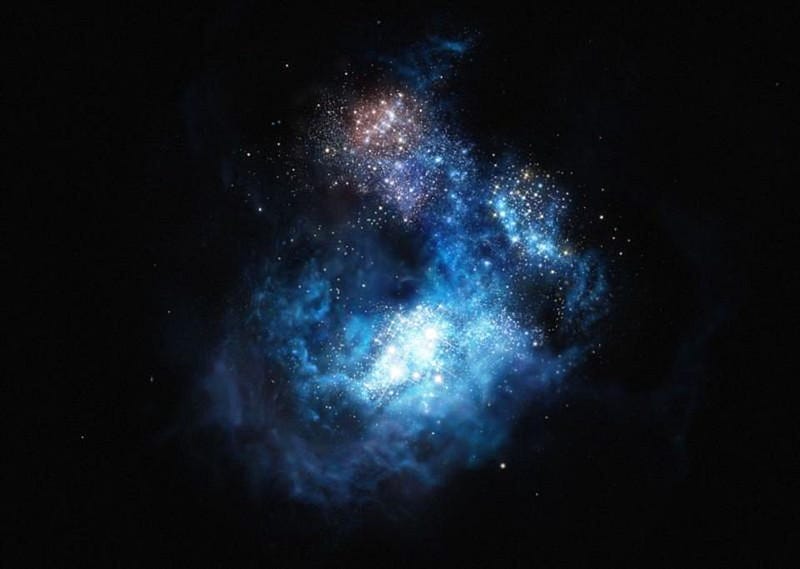
No one, to date, has ever found a first-generation star, counterintuitively known among astronomers as Population III stars. Why? Because stellar populations were named in the order they were discovered. The Sun is a Population I star, but it’s highly processed and made out of metal-rich material that has gone through many generations of stellar life-and-death.
The second population ever discovered, Population II stars, are these metal-poor stars that form as early as the second generation of all stars. They can live an extremely long time, and a few of them, like the famed Methuselah star, are still around in our galaxy today, despite being over 13 billion years in age. But Population III stars have yet to be discovered; they ought to exist, but are only theoretical at this point.
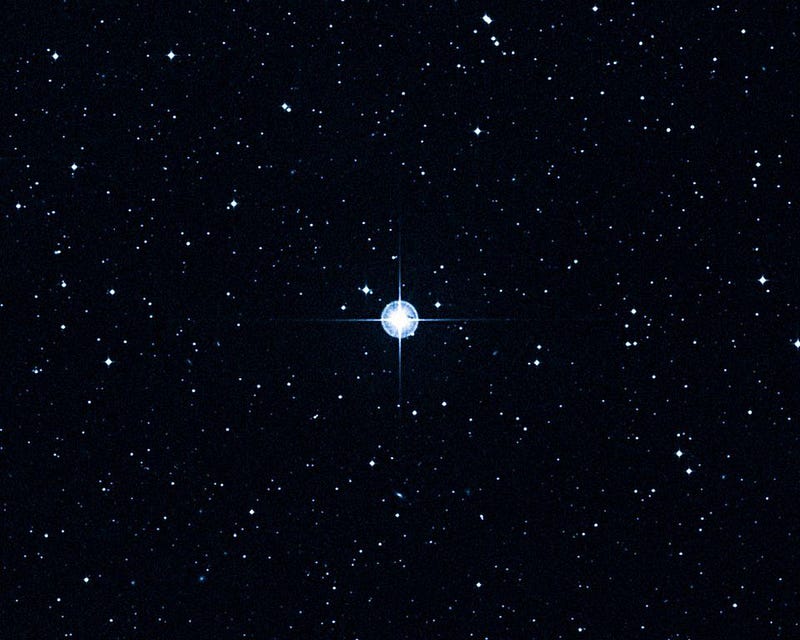
In addition, there’s one more difference between Population II stars and Population III stars: the possibility of planets. The very first stars, composed of hydrogen and helium alone, could only conceivably create tenuous, massive, puffy gas giants. Without a massive, dense core, they’re easily evaporated and dissociated by too much radiation.
But with the presence of metals, all of a sudden you can form dense, rocky clumps in your protoplanetary disk, which leads to a mix of rocky and gaseous planets. Once you make the second generation of stars, you can make planets too, complete with complex and even organic molecules.
The very first stars live only an extremely short time, owing to their high masses and large luminosities and rates-of-fusion. When they die, the space around them becomes polluted with the fruits of their lives: heavy elements. These heavy elements enable the second generation of stars to form, but they now form differently. The heavy elements radiate heat away, giving rise to a less massive, more diverse generation of stars, some of which survive even to the present day.
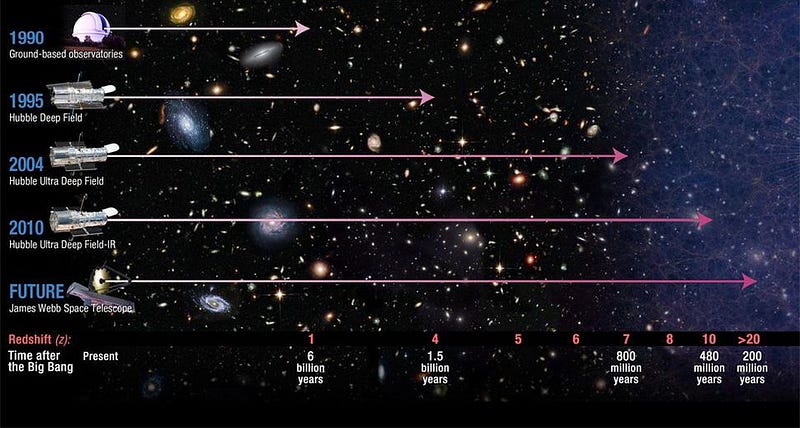
When the James Webb Space Telescope begins operations, it may yet reveal a population of these first stars, likely to be found alongside polluted, second-generation stars. But once these second-generation stars begin to form, they make something else possible: the first galaxies. And that, in just a few years, is likely where the James Webb Space Telescope will truly shine.
Further reading on what the Universe was like when:
- What was it like when the Universe was inflating?
- What was it like when the Big Bang first began?
- What was it like when the Universe was at its hottest?
- What was it like when the Universe first created more matter than antimatter?
- What was it like when the Higgs gave mass to the Universe?
- What was it like when we first made protons and neutrons?
- What was it like when we lost the last of our antimatter?
- What was it like when the Universe made its first elements?
- What was it like when the Universe first made atoms?
- What was it like when there were no stars in the Universe?
- What was it like when the first stars began illuminating the Universe?
- What was it like when the first stars died?
- What was it like when the Universe made its second generation of stars?