Why did the Universe start off with Hydrogen, Helium, and not much else?
Where the first atoms in the Universe — the progenitors of all the normal matter that makes up everything we know — came from.
“I see a lot of new faces. But, you know the old saying, ‘out with the old, in with the nucleus.’” –The Simpsons
Looking around the Universe today, there’s no doubt that there’s plenty of hydrogen and helium around; after all, it’s the nuclear fusion of hydrogen into helium that powers the vast majority of stars illuminating the entire cosmos!
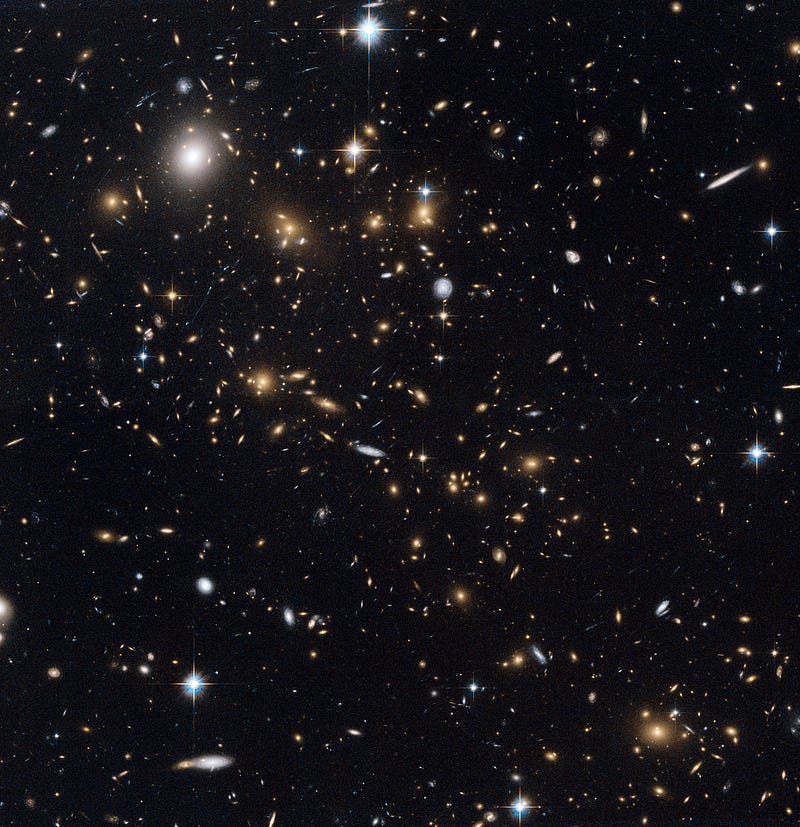
But here on Earth, hydrogen and helium are only a small part of the world we inhabit. By mass, hydrogen and helium combined make up far less than 1% of the Earth, and even if we restrict ourselves to the Earth’s crust, it’s still just a tiny percentage compared to the other, heavier elements.
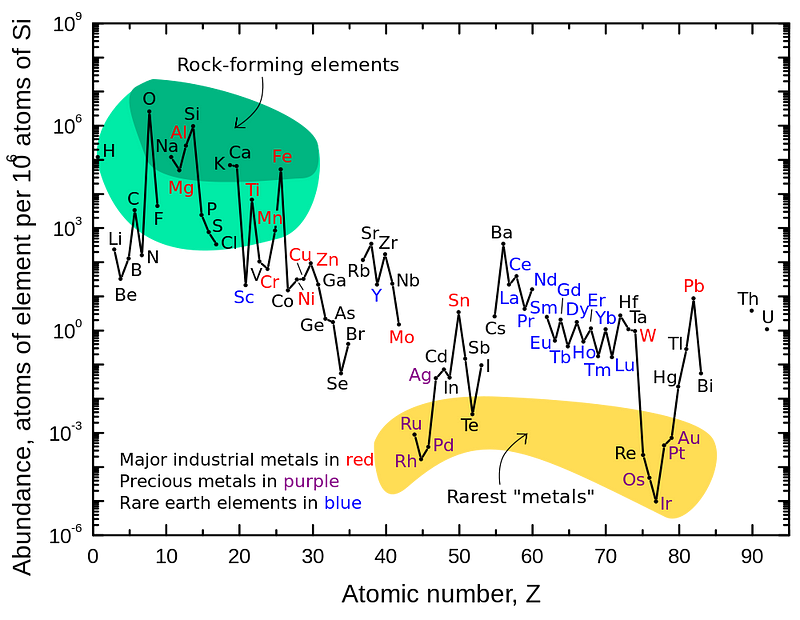
Practically all of these heavy elements were formed in generations of stars: stars that lived, burned their fuel into heavier elements, died and shed their heavy, enriched elements back into the cosmos. Those heavier elements, along with a mix of the original ones, were incorporated into the next generations of stars and eventually — when the heavier elements became abundant enough — rocky planets.
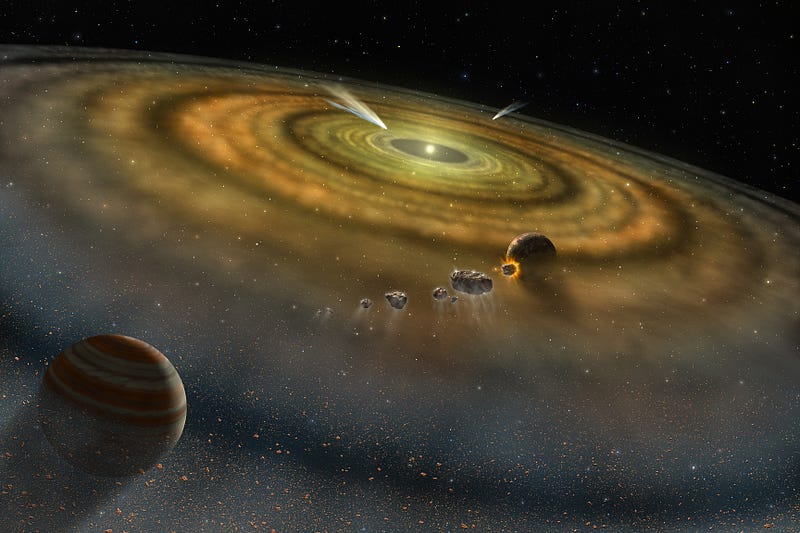
But the Universe didn’t start off with these heavier elements at all. In fact, if you’ll remember what the Big Bang says, the Universe is expanding (and cooling) now, meaning that all the matter in it was closer together — and the radiation in it was hotter — in the past. If you go back to a sufficiently early time, you’ll find that the density was high enough and the temperature was hot enough that you couldn’t even form neutral atoms without them immediately being blasted apart! When the Universe cooled through that phase, that’s when neutral atoms formed for the first time, and where the cosmic microwave background comes from.
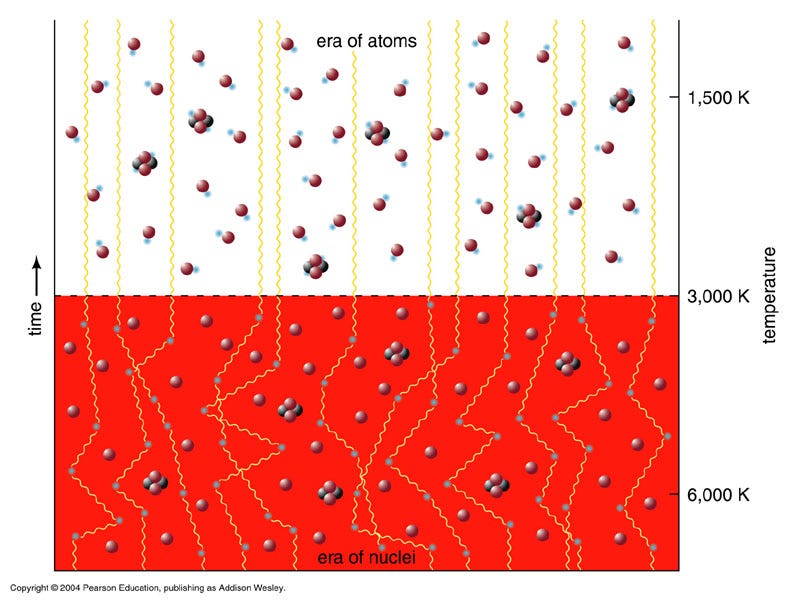
At that time, the Universe was made out of about 92% hydrogen atoms and 8% helium atoms by number (or about 75-76% hydrogen and 24-25% helium by mass), with trace amounts of lithium and beryllium, but not much else. But you might wonder how it got to have exactly that ratio? After all, it didn’t have to be that way; if the Universe was hot and dense enough to undergo nuclear fusion early on, why did it only fuse atoms up to helium, and why didn’t more of the Universe become helium than it did?
To find the answer, we need to go way back in time. Not just to the first few hundred thousand years of the Universe, when it was making the first atoms, nor even to the first years, days, or hours. No, we need to go back to when the temperatures were so high, when the Universe was so hot, that not only could atomic nuclei not form (for they’d be immediately be blasted apart), but to a time when the Universe was so hot that the Universe was filled with nearly equal amount of matter-and-antimatter, when it was just a fraction of a second old!
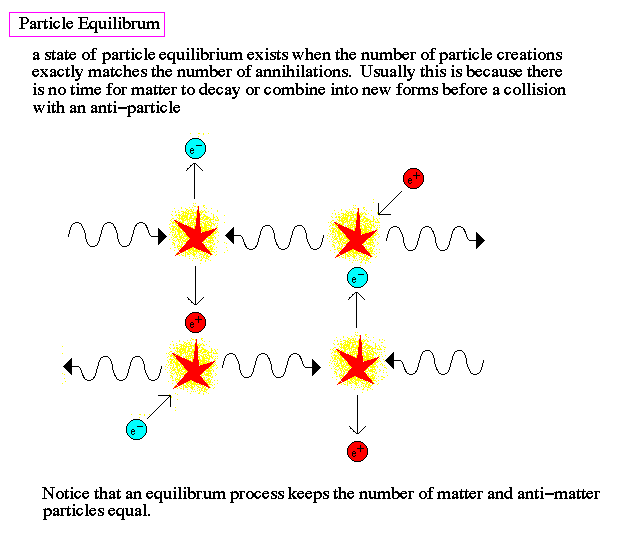
It was once so hot that the Universe was filled with nearly equal amount of matter and antimatter: protons and antiprotons, neutrons and antineutrons, electrons and positrons, neutrinos and antineutrinos, and of course photons (which are their own antiparticle), among others. (They’re not exactly equal; see here for more on that.)
When the Universe is hot — and by hot, I mean above the temperature needed to spontaneously create a matter/antimatter pair from two typical photons — you get huge amounts of that form of matter and antimatter. They get spontaneously created from photons just as quickly as they find one another and annihilate back into photons. But as the Universe cools, those matter/antimatter pairs begin to annihilate faster, and it becomes more difficult to find photons energetic enough to make them. Eventually, it cools enough that all the exotic particles go away, and all the antiprotons and antineutrons annihilate with protons and neutrons, leaving only a small asymmetry of matter (in the form of protons and neutrons) over antimatter, bathed in a sea of radiation.
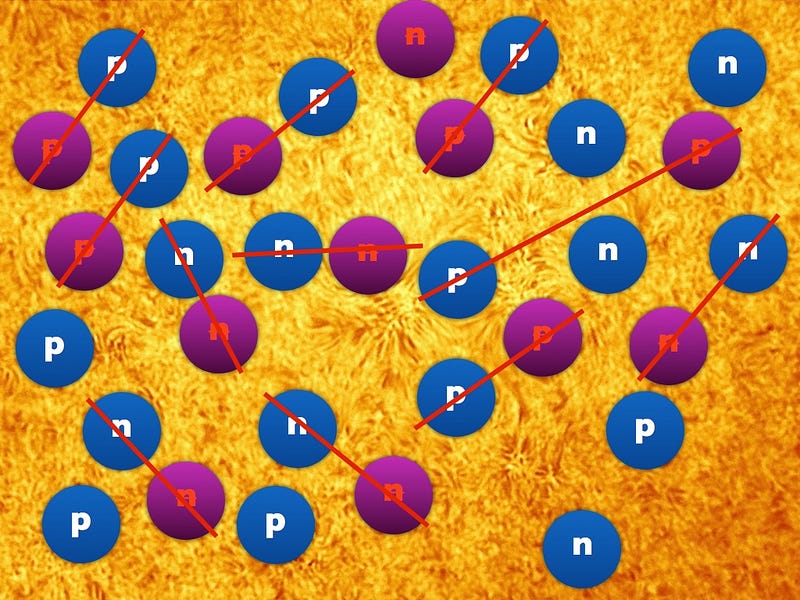
At this point, when the Universe is a fraction of a second old, there are roughly equal amounts of protons and neutrons: about a 50/50 split. These protons and neutrons will eventually become the atoms in our Universe, but they’ve got a lot to go through first. On the other hand, electrons (and positrons) are much lighter, so they still exist in huge numbers (and at great energies) for a while longer.
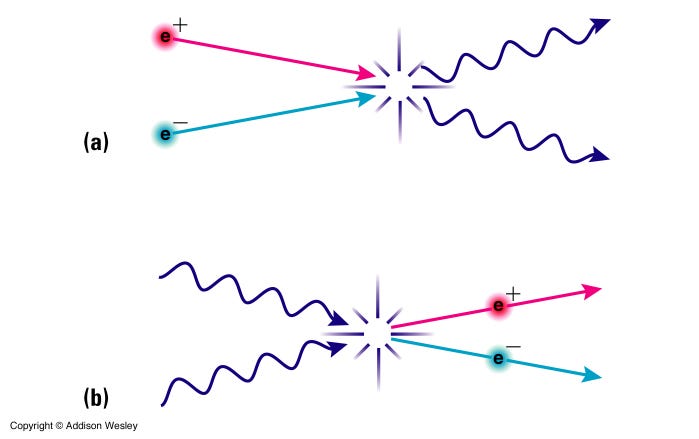
It’s still hot enough that protons and neutrons can convert into one another very easily: a proton can combine with an electron to make a neutron and (an electron) neutrino, while a neutron can combine with (an electron) neutrino to make a proton and an electron. While there aren’t that many protons and neutrons in the Universe at this time, electrons and neutrinos outnumber them by around a billion-to-one. The process is known as proton-neutron interconversion, and at these high temperatures, the reactions are equally efficient. This is why, early on, there’s about a 50/50 split of protons and neutrons.
Neutrons, as you’ll remember, are slightly heavier than protons: by about 0.2%. As the Universe cools (and the excess positrons annihilate away), it becomes rarer and rarer to find a proton-electron pair with enough energy to create a neutron, while it’s still relatively easy for a neutron-neutrino pair to create a proton-electron pair. This converts a substantial fraction of neutrons into protons during the first one-to-three seconds of the Universe. By time these interactions have become insignificant, the proton-to-neutron ratio has changed from about 50/50 to 85/15!
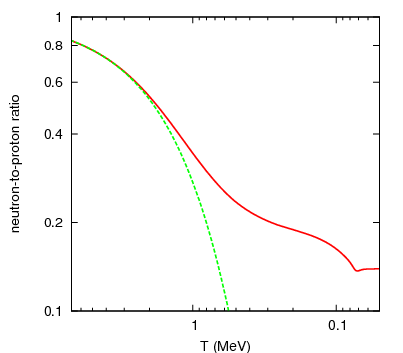
Now, these protons and neutrons are abundant, hot, and dense enough that they can fuse together into heavier elements, and believe me, they’d love to. But photons — particles of radiation — outnumber protons-and-neutrons by more than a billion to one, so for minutes of the Universe expanding and cooling, it’s still energetic enough that every time a proton and neutron fuse together to form deuterium, the first stepping-stone in nuclear fusion, a high-enough energy photon immediately comes along and blasts them apart! This is known as the deuterium bottleneck, as deuterium is relatively fragile, and its fragility prevents further nuclear reactions from occurring.
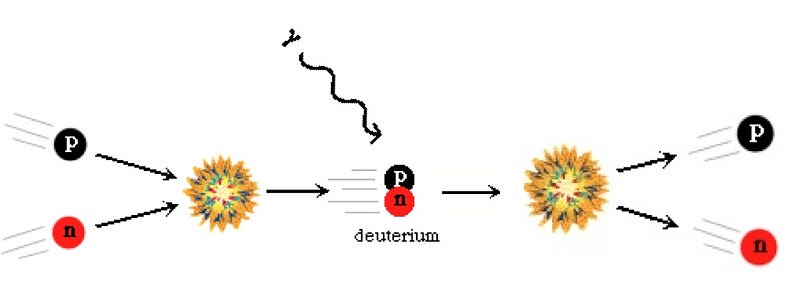
In the meantime, while the minutes tick by, something else is going on. A free proton is stable, so nothing happens to them, but a free neutron is unstable; it will decay with a half-life of about ten minutes into a proton, electron, and an (electron) antineutrino. By time the Universe has cooled enough that the created deuterium wouldn’t be immediately be blasted back apart, more than three minutes have gone by, further changing the 85%-proton/15%-neutron split to nearly 88% protons and just a hair over 12% neutrons.
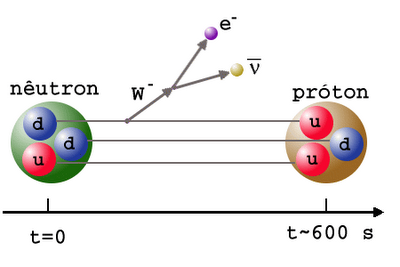
Finally, with deuterium forming, nuclear fusion can proceed, and it proceeds extremely rapidly! Through a couple of different fusion chains, the Universe is still hot and dense enough that pretty much every neutron around wind up combining with one other neutron and two protons to form helium-4, an isotope of helium that’s much more energetically stable than deuterium, tritium, or helium-3!
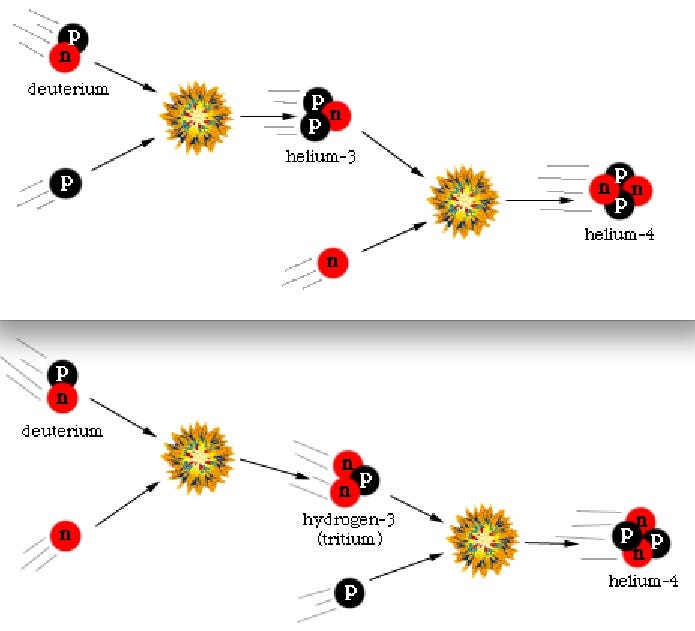
By time this happens, though, the Universe is nearly four minutes old, and is far too diffuse and cold to undergo the next major step of fusion. There are still protons and helium nuclei flying around, but a proton and a helium-4 nucleus can’t fuse, as there’s no stable mass-5 nucleus, and two helium-4s produce the highly unstable beryllium-8 isotope, which decays back to two helium-4s on timescales of ~10^-16 seconds! No, that next step is to fuse three helium-4 atoms into carbon-12, but the Universe is no longer dense or energetic enough to support that interaction; that process will have to wait tens of millions of years until the Universe’s first stars form!
But these hydrogen and helium-4 nuclei are stable, and there will also be a trace amount of helium-3 (which tritium will also decay into, eventually), deuterium (hydrogen-2), and very small amounts of lithium (and probably even smaller amounts of beryllium-9) formed by very rare fusion reactions.
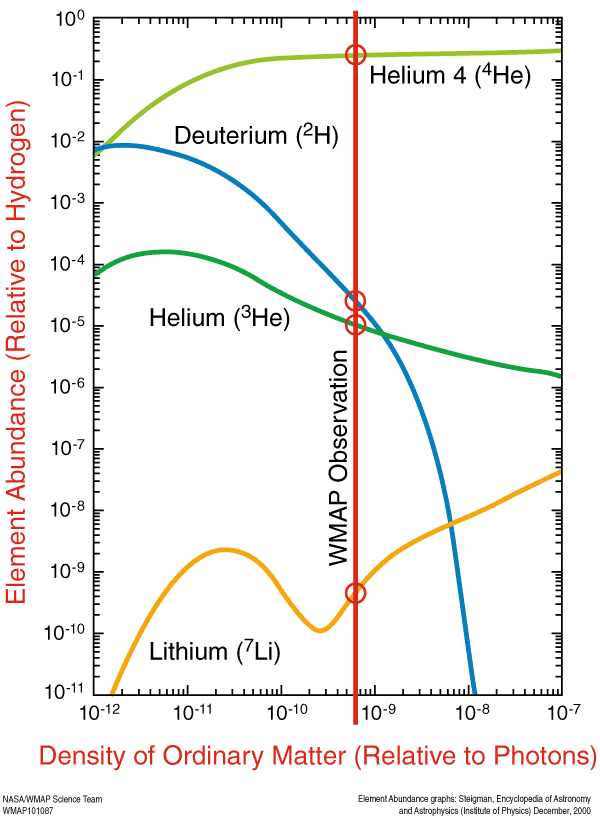
But the overwhelming majority of neutrons — 99.9%+ of them — wind up locked up in helium-4 nuclei. If the matter in the Universe contained just a hair over 12% neutrons and just a hair under 88% protons just prior to nucleosynthesis (the fusion into heavier elements), that means that all of those neutrons and and equal amount (just over 12% of the Universe) of protons winds up becoming helium-4: a total of 24-to-25% of the mass, leaving 75-to-76% of the Universe as protons, or hydrogen nuclei.
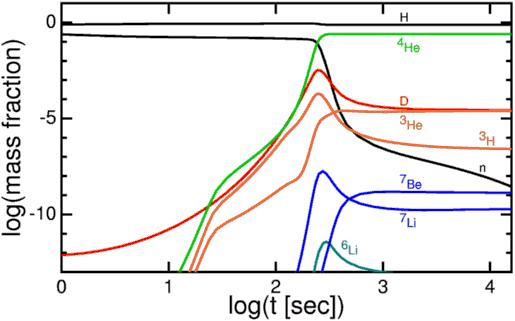
So that’s why, by mass, we say 75-76% was hydrogen and 24-25% was helium. But each helium nucleus is around four times the mass of a hydrogen nucleus, which means that, by number of atoms, the Universe is around 92% hydrogen and 8% helium.
This primordial, unprocessed material has actually been detected observationally, and is one of the three cornerstones of the Big Bang, along with Hubble expansion and the cosmic microwave background. And that’s where all the elements in the Universe started from! Everything you are, everything you know, and every material object you’ve ever interacted with came from this primordial sea of protons and neutrons, and was once a mere collections of hydrogen and helium atoms. And then the Universe happened…
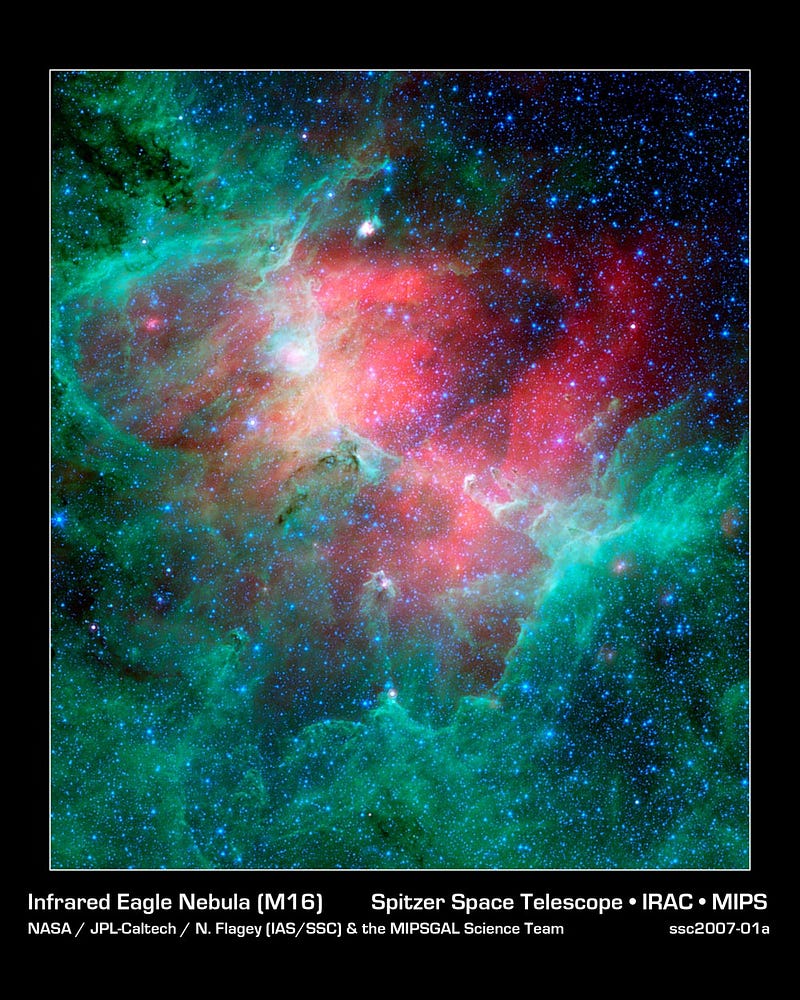
and here it all is! And that’s where — if you go way, way back — all the atoms we have in our Universe today began.
An earlier version of this post originally appeared on the old Starts With A Bang blog at Scienceblogs.